Magnesium isotopic systematics of metapelite in the deep crust and implications for granite petrogenesis
Affiliations | Corresponding Author | Cite asKeywords: magnesium isotopes, fractionation, granite, metamorphism, biotite, garnet
- Share this article
-
Article views:9,230Cumulative count of HTML views and PDF downloads.
- Download Citation
- Rights & Permissions
Abstract

Figures and Tables
![]() Figure 1 Magnesium isotopic compositions versus metamorphic grade for the bulk metapelites, biotites and garnets. The average value of bulk upper continental crust (δ26Mg = -0.22 ‰) is represented by the blue line (Li et al., 2010). Data are reported in Table S-1. | ![]() Figure 2 Magnesium isotope fractionation between biotite and garnet (Δ26MgBt-Grt = δ26MgBt - δ26MgGrt) as a function of 1/T2. Empirically and theoretically determined equilibrium equations of clinopyroxene-garnet Mg isotope fractionation are also shown for comparison. ① is an empirical equilibrium fractionation equation of Δ26Mgclinpyroxene–garnet = 0.83 × 106/T2 from Li et al. (2011); ② is also an empirical equilibrium fractionation equation of Δ26Mgclinpyroxene–garnet = 0.86 × 106/T2 from Wang et al. (2012); ③ and ④ represent the theoretically determined equilibrium fractionation equations at pressures of 0 and 10 kbar (Huang et al., 2013), respectively. The temperatures for the studied metapelites, estimated using biotite-garnet Mg-Fe exchange geothermometer, are from Bea and Montero (1999). Data are reported in Table S-1. | ![]() Figure 3 The variation of Grt/(Bt+Grt) ratio, δ26MgBt, δ26MgGrt and temperature as a function of pressure (crustal depth). Also shown is a cartoon illustrating the generation of granitic melts at different crustal depths. For those samples whose mineral separates are unavailable, Mg isotopic compositions of biotite and garnet are calculated based on the mineral modes and chemistries, assuming an inter-mineral Mg isotope fractionation of ~ 1.00 ‰. The mineral modes, compositions and temperatures are from Bea and Montero (1999). |
Figure 1 | Figure 2 | Figure 3 |
Supplementary Figures and Tables
![]() Figure S-1 Sketch map showing the location of Ivrea Zone, NW Italy (modified from Bea and Montero, 1999). The labels indicate the samples listed in Table S-1. | ![]() Figure S-2 Plots of biotite δ26Mg versus biotite mode (a) and garnet δ26Mg versus garnet mode (b) in metapelites. The modal abundances of biotite and garnet are from Bea and Montero (1999). The Mg isotopic data are reported in Table S-1. | ![]() Figure S-3 Modelling showing the evolution of melt δ26Mg values as a function of melt fraction (a) and peritectic garnet mode (b) during progressive biotite dehydration melting. The stoichiometry of biotite dehydration melting reaction is: 1 biotite + 0.55 plagioclase + 0.14 aluminosilicate + 0.76 quartz = 1.72 melt + 0.73 garnet (Patiño Douce and Johnston, 1991). Biotite-limited melting and plagioclase-limited melting indicate that melting terminates when biotite and plagioclase in the source is exhausted, respectively. In the modelling, the protoliths are assumed to have δ26Mg value of +0.15 ‰ and MgO of 3 wt.%; the partition coefficient of Mg between biotite and garnet (Dbiotite/garnet) is roughly assumed to be 2 based on the mineral major compositions (Bea and Montero, 1999). The mineral modes in P1 (biotite : plagioclase : aluminosilicate : quartz : others = 0.26 : 0.09 : 0.11 : 0.49 : 0.05) and P2 (biotite : plagioclase : aluminosilicate : quartz : garnet: others = 0.22 : 0.17 : 0.04 : 0.52 : 0.015 : 0.035) are taken as examples to model plagioclase-limited melting and biotite-limited melting, respectively. The inter-mineral Mg isotope fractionation between biotite and garnet is assumed to be ~1.00 ‰. | ![]() Table S-1 Magnesium isotopic compositions (‰) of bulk rocks and mineral separates for the metapelites from the Ivrea Zone, NW Italy. |
Figure S-1 | Figure S-2 | Figure S-3 | Table S-1 |
top
Introduction
Fluid-absent incongruent melting of hydrous minerals such as muscovite, biotite, and amphibole that takes place in the continental crust give rise to large volumes of granitoids (Clemens and Vielzeuf, 1987
Clemens, J., Vielzeuf, D. (1987) Constraints on melting and magma production in the crust. Earth and Planetary Science Letters 86, 287-306.
; Clemens and Watkins, 2001Clemens, J., Watkins, J. (2001) The fluid regime of high-temperature metamorphism during granitoid magma genesis. Contributions to Mineralogy and Petrology 140, 600-606.
). Pelitic rocks containing abundant mica minerals are the most fertile lithologies in the deep (middle and lower) continental crust, and can produce as much as 50 vol.% melt as the temperature rises above 700 ºC (Le Breton and Thompson, 1988Le Breton, N., Thompson, A.B. (1988) Fluid-absent (dehydration) melting of biotite in metapelites in the early stages of crustal anatexis. Contributions to Mineralogy and Petrology 99, 226-237.
; Vielzeuf and Holloway, 1988Vielzeuf, D., Holloway, J.R. (1988) Experimental determination of the fluid-absent melting relations in the pelitic system. Contributions to Mineralogy and Petrology 98, 257-276.
). Dry, granulitic residues after an early melt extraction are relatively refractory but may still be melted at higher temperatures (e.g., >830 ºC; Whalen et al., 1987Whalen, J.B., Currie, K.L., Chappell, B.W. (1987) A-type granites: geochemical characteristics, discrimination and petrogenesis. Contributions to Mineralogy and Petrology 95, 407-419.
). Consequently, the elemental and isotopic diversity of granitoids results from a combination of protolith heterogeneities, melting reaction patterns, melt extraction and ascending processes (Barbero et al., 1995Barbero, L., Villaseca, C., Rogers, G., Brown, P. (1995) Geochemical and isotopic disequilibrium in crustal melting: an insight from the anatectic granitoids from Toledo, Spain. Journal of Geophysical Research: Solid Earth 100, 15745-15765.
; Bea, 1996Bea, F. (1996) Controls on the trace element composition of crustal melts. Geological Society of America Special Papers 315, 33-41.
; Patiño Douce, 1999Patiño Douce, A. (1999) What do experiments tell us about the relative contributions of crust and mantle to the origin of granitic magmas? Geological Society Special Publications 168, 55-75.
; Brown, 2007Brown, M. (2007) Crustal melting and melt extraction, ascent and emplacement in orogens: mechanisms and consequences. Journal of the Geological Society 164, 709-730.
; Stevens et al., 2007Stevens, G., Villaros, A., Moyen, J.-F. (2007) Selective peritectic garnet entrainment as the origin of geochemical diversity in S-type granites. Geology 35, 9-12.
; Clemens and Stevens, 2012Clemens, J., Stevens, G. (2012) What controls chemical variation in granitic magmas? Lithos 134, 317-329.
; Lee and Morton, 2015Lee, C.-T.A., Morton, D.M. (2015) High silica granites: Terminal porosity and crystal settling in shallow magma chambers. Earth and Planetary Science Letters 409, 23-31.
).Magnesium (Mg) in anatectic melts may come exclusively from the mafic hydrous minerals participating in the melting reactions. Taking anatexis of the metapelitic system as an example, micas (mainly muscovite and biotite) in the source play the first order control on the Mg isotopic composition (δ26Mg) of resulting melts. The δ26Mg values of global granites range from -0.45 to +0.44 ‰ (Shen et al., 2009
Shen, B., Jacobsen, B., Lee, C.-T.A., Yin, Q.-Z., Morton, D.M. (2009) The Mg isotopic systematics of granitoids in continental arcs and implications for the role of chemical weathering in crust formation. Proceedings of the National Academy of Sciences 106, 20652-20657.
; Li et al., 2010Li, W.-Y., Teng, F.-Z., Ke, S., Rudnick, R.L., Gao, S., Wu, F.-Y., Chappell, B.W. (2010) Heterogeneous magnesium isotopic composition of the upper continental crust. Geochimica et Cosmochimica Acta 74, 6867-6884.
; Liu et al., 2010Liu, S.-A., Teng, F.-Z., He, Y., Ke, S., Li, S. (2010) Investigation of magnesium isotope fractionation during granite differentiation: implication for Mg isotopic composition of the continental crust. Earth and Planetary Science Letters 297, 646-654.
; Telus et al., 2012Telus, M., Dauphas, N., Moynier, F., Tissot, F.L.H., Teng, F.-Z., Nabelek, P.I., Craddock, P.R., Groat, L.A. (2012) Iron, zinc, magnesium and uranium isotopic fractionation during continental crust differentiation: The tale from migmatites, granitoids, and pegmatites. Geochimica et Cosmochimica Acta 97, 247-265.
; Ling et al., 2013Ling, M.-X., Li, Y., Ding, X., Teng, F.-Z., Fan, W., Xu, Y., Sun, W.-D. (2013) Destruction of the North China Craton induced by ridge subductions. The Journal of Geology 121, 197-213.
; Wang et al., 2014bWang, S.-J., Teng, F.-Z., Li, S.-G., Hong, J.-A. (2014b) Magnesium isotopic systematics of mafic rocks during continental subduction. Geochimica et Cosmochimica Acta 143, 34-48.
), far exceeding that of normal mantle rocks (-0.25 ± 0.07 ‰; Teng et al., 2010aTeng, F.-Z., Li, W.-Y., Ke, S., Marty, B., Dauphas, N., Huang, S.-C., Wu, F.-Y., Pourmand, A. (2010a) Magnesium isotopic composition of the Earth and chondrites. Geochimica et Cosmochimica Acta 74, 4150-4166.
). While protolith heterogeneity (i.e. sedimentary vs. igneous protoliths) partially explains this Mg isotopic variation (Shen et al., 2009Shen, B., Jacobsen, B., Lee, C.-T.A., Yin, Q.-Z., Morton, D.M. (2009) The Mg isotopic systematics of granitoids in continental arcs and implications for the role of chemical weathering in crust formation. Proceedings of the National Academy of Sciences 106, 20652-20657.
), metamorphic overprints on potential granitoid sources, with concomitant changes of source mineralogy, may play an important, but poorly understood, role as well.Here, we present Mg isotope data for 12 well-characterised metapelites and 13 mineral separates (biotite and garnet) therein from the Ivrea Zone, NW Italy (Bea and Montero, 1999
Bea, F., Montero, P. (1999) Behavior of accessory phases and redistribution of Zr, REE, Y, Th, and U during metamorphism and partial melting of metapelites in the lower crust: an example from the Kinzigite Formation of Ivrea-Verbano, NW Italy. Geochimica et Cosmochimica Acta 63, 1133-1153.
; Qiu et al., 2011Qiu, L., Rudnick, R.L., McDonough, W.F., Bea, F. (2011) The behavior of lithium in amphibolite-to granulite-facies rocks of the Ivrea–Verbano Zone, NW Italy. Chemical Geology 289, 76-85.
). These metapelites represent a typical prograde metamorphic sequence from middle amphibolite- to granulite-facies, and thus are ideal samples for studying the behaviour of Mg isotopes under the middle-lower crustal conditions. Our results indicate that the bulk metapelites display a considerable Mg isotopic variation (δ26Mg = -0.23 to +0.20 ‰) that does not correlate with metamorphic grade. By contrast, the δ26Mg values of biotite (-0.08 to +1.10 ‰) and garnet (-1.22 to +0.10 ‰) vary more significantly and increase as metamorphism proceeds, which makes Mg isotopes a potential tracer for crustal melting and granite petrogenesis.top
Ivrea Zone Metapelites
The Ivrea Zone, NW Italy, represents an exposed section of the middle to lower crust and preserves a transition from amphibolite- to granulite-facies metapelites (Zingg, 1984
Zingg, A. (1984) Development Stages of Ivrea Paragneiss (Southern Alps, Northern Italy). Fortschritte Der Mineralogie 62, 266-268.
). The metamorphic condition for these metapelites ranges from ca. 4 kbar at 500 ºC to ca.10 ~ 12 kbar at >900 ºC (Henk et al., 1997Henk, A., Franz, L., Teufel, S., Oncken, O. (1997) Magmatic underplating, extension, and crustal reequilibration: insights from a cross-section through the Ivrea Zone and Strona-Ceneri Zone, Northern Italy. The Journal of Geology 105, 367-378.
; Bea and Montero, 1999Bea, F., Montero, P. (1999) Behavior of accessory phases and redistribution of Zr, REE, Y, Th, and U during metamorphism and partial melting of metapelites in the lower crust: an example from the Kinzigite Formation of Ivrea-Verbano, NW Italy. Geochimica et Cosmochimica Acta 63, 1133-1153.
; Luvizotto and Zack, 2009Luvizotto, G., Zack, T. (2009) Nb and Zr behavior in rutile during high-grade metamorphism and retrogression: an example from the Ivrea-Verbano Zone. Chemical Geology 261, 303-317.
; Redler et al., 2011Redler, C., Johnson, T., White, R., Kunz, B. (2011) Phase equilibrium constraints on a deep crustal metamorphic field gradient: metapelitic rocks from the Ivrea Zone (NW Italy). Journal of Metamorphic Geology 30, 235-254.
). The modal abundance of garnet in metapelites increases at the expense of biotite with increasing metamorphic grade following the metamorphic reaction: biotite + sillimanite + plagioclase + quartz → garnet + K-feldspar + rutile + melt. This reaction proceeds until the biotite is almost entirely consumed in granulite-facies metapelites (Bertolani and Garuti, 1970Bertolani, M., Garuti, G. (1970) Aspetti petrografici della formazione basica Ivrea-Verbano in Val Sessera (Vercelli). Rendiconti Societa Italiana di Mineralogia e Petrografia 32, 434-474.
; Schmid and Wood, 1976Schmid, R., Wood, B. (1976) Phase relationships in granulitic metapelites from the Ivrea-Verbano Zone (Northern Italy). Contributions to Mineralogy and Petrology 54, 255-279.
). The metapelites in the Ivrea Zone are divided into three zones from low to high metamorphic grade based on the biotite/garnet ratio (Bea and Montero, 1999Bea, F., Montero, P. (1999) Behavior of accessory phases and redistribution of Zr, REE, Y, Th, and U during metamorphism and partial melting of metapelites in the lower crust: an example from the Kinzigite Formation of Ivrea-Verbano, NW Italy. Geochimica et Cosmochimica Acta 63, 1133-1153.
): the kinzigite zone that corresponds to metapelites with modal biotite/garnet >2, the transition zone in which the modal biotite/garnet is between 0.5 and 2, and the stronalite zone where modal biotite/garnet is <0.5 (Fig. S-1). Twelve representative samples of metapelites and garnet-biotite mineral pairs from the three zones were studied for Mg isotopes (Fig. S-1).top
Results
The Mg isotopic data, along with details of the analytical methods, are presented in the Supplementary Information and summarised in Figure 1. The bulk metapelites display a considerable variation in Mg isotopic composition, with δ26Mg ranging from -0.23 to +0.20 ‰ for the kinzigite zone, from -0.10 to +0.14 ‰ for the transition zone, and from -0.10 to +0.12 ‰ for the stronalite zone. No correlation between bulk δ26Mg and metamorphic grade is observed (Fig. 1). By contrast, δ26Mg values of the associated mineral separates display wider ranges and increase systematically with metamorphic grade (Fig. 1). The Mg isotopic compositions of garnet become progressively heavy from the kinzigite zone (δ26Mg = -1.10 to -1.22 ‰) to the transition zone (δ26Mg = -0.34 ‰) and finally to the stronalite zone (δ26Mg = -0.03 to +0.10 ‰; Fig. 1). The biotite is isotopically heavier than coexisting garnet, with δ26Mg increasing from the kinzigite zone (-0.08 to +0.30 ‰) through the transition zone (+0.58 ‰) to the stronalite zone (+1.00 to +1.10 ‰; Fig. 1).

Figure 1 Magnesium isotopic compositions versus metamorphic grade for the bulk metapelites, biotites and garnets. The average value of bulk upper continental crust (δ26Mg = -0.22 ‰) is represented by the blue line (Li et al., 2010
Li, W.-Y., Teng, F.-Z., Ke, S., Rudnick, R.L., Gao, S., Wu, F.-Y., Chappell, B.W. (2010) Heterogeneous magnesium isotopic composition of the upper continental crust. Geochimica et Cosmochimica Acta 74, 6867-6884.
). Data are reported in Table S-1.top
Discussion and Conclusion
Minerals with low Mg coordination numbers (CN) and thus strong Mg-O bonds preferentially incorporate heavy Mg isotopes. Given the difference in Mg coordination geometry between biotite (CN = 6) and garnet (CN = 8), substantial inter-mineral isotope fractionation is expected, but the exact fractionation factor has not been determined. Our study shows that the Mg isotope fractionation between biotite and garnet is significant, in the range of 0.92 ~ 1.48 ‰ (Fig. 2). Different mineral fragments from the same metapelite yield identical Mg isotopic compositions (Table S-1), suggesting negligible intra-mineral Mg isotopic variations and thus implying the equilibrium Mg isotope fractionation. The degree of equilibrium isotope fractionation is a function of temperature, with 103 lnαbiotite-garnet = 0.96 × 106/T2 (R2 = 0.7; Fig. 2). This fractionation equation is very similar to those previously reported for garnet-clinopyroxene pairs from eclogites (Fig. 2) (Li et al., 2011
Li, W.-Y., Teng, F.-Z., Xiao, Y., Huang, J. (2011) High-temperature inter-mineral magnesium isotope fractionation in eclogite from the Dabie orogen, China. Earth and Planetary Science Letters 304, 224-230.
; Wang et al., 2012Wang, S.-J., Teng, F.-Z., Williams, H.M., Li, S.-G. (2012) Magnesium isotopic variations in cratonic eclogites: Origins and implications. Earth and Planetary Science Letters 359, 219-226.
, 2014aWang, S.-J., Teng, F.-Z., Li, S.-G. (2014a) Tracing carbonate-silicate interaction during subduction using magnesium and oxygen isotopes. Nature Communications 5, doi:10.1038/ncomms6328.
, bWang, S.-J., Teng, F.-Z., Li, S.-G., Hong, J.-A. (2014b) Magnesium isotopic systematics of mafic rocks during continental subduction. Geochimica et Cosmochimica Acta 143, 34-48.
; Huang et al., 2013Huang, F., Chen, L., Wu, Z., Wang, W. (2013) First-principles calculations of equilibrium Mg isotope fractionations between garnet, clinopyroxene, orthopyroxene, and olivine: Implications for Mg isotope thermometry. Earth and Planetary Science Letters 367, 61-70.
), which is reasonable since the Mg coordination geometry in biotite is the same as in clinopyroxene. The relatively high scatter of data may reflect an additional but small effect from pressure, as first-principles calculations suggest that the equilibrium inter-mineral Mg isotope fractionation is also pressure-dependent; i.e. the degree of fractionation is suppressed as pressure decreases (Huang et al., 2013Huang, F., Chen, L., Wu, Z., Wang, W. (2013) First-principles calculations of equilibrium Mg isotope fractionations between garnet, clinopyroxene, orthopyroxene, and olivine: Implications for Mg isotope thermometry. Earth and Planetary Science Letters 367, 61-70.
). Nevertheless, our results indicate for the first time that Mg isotopes might be used as a novel geothermometer for garnet and biotite-bearing rocks.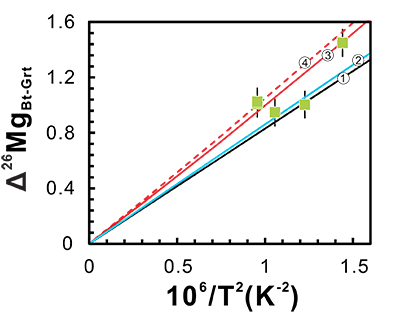
Figure 2 Magnesium isotope fractionation between biotite and garnet (Δ26MgBt-Grt = δ26MgBt - δ26MgGrt) as a function of 1/T2. Empirically and theoretically determined equilibrium equations of clinopyroxene-garnet Mg isotope fractionation are also shown for comparison. ① is an empirical equilibrium fractionation equation of Δ26Mgclinopyroxene–garnet = 0.83 × 106/T2 from Li et al. (2011)
Li, W.-Y., Teng, F.-Z., Xiao, Y., Huang, J. (2011) High-temperature inter-mineral magnesium isotope fractionation in eclogite from the Dabie orogen, China. Earth and Planetary Science Letters 304, 224-230.
; ② is also an empirical equilibrium fractionation equation of Δ26Mgclinopyroxene–garnet = 0.86 × 106/T2 from Wang et al. (2012)Wang, S.-J., Teng, F.-Z., Williams, H.M., Li, S.-G. (2012) Magnesium isotopic variations in cratonic eclogites: Origins and implications. Earth and Planetary Science Letters 359, 219-226.
; ③ and ④ represent the theoretically determined equilibrium fractionation equations at pressures of 0 and 10 kbar (Huang et al., 2013Huang, F., Chen, L., Wu, Z., Wang, W. (2013) First-principles calculations of equilibrium Mg isotope fractionations between garnet, clinopyroxene, orthopyroxene, and olivine: Implications for Mg isotope thermometry. Earth and Planetary Science Letters 367, 61-70.
), respectively. The temperatures for the studied metapelites, estimated using biotite-garnet Mg-Fe exchange geothermometer, are from Bea and Montero (1999)Bea, F., Montero, P. (1999) Behavior of accessory phases and redistribution of Zr, REE, Y, Th, and U during metamorphism and partial melting of metapelites in the lower crust: an example from the Kinzigite Formation of Ivrea-Verbano, NW Italy. Geochimica et Cosmochimica Acta 63, 1133-1153.
. Data are reported in Table S-1.The δ26Mg values of the bulk-rock do not correlate with metamorphic grade (Fig. 1), suggesting that metamorphic dehydration/partial melting during the transition from amphibolite- to granulite-facies caused negligible change of Mg isotopes of metapelites. This conclusion is consistent with previous studies that show limited Mg isotope fractionation by either low- or high-temperature metamorphic dehydration (Teng et al., 2013
Teng, F.Z., Yang, W., Rudnick, R.L., Hu, Y. (2013) Heterogeneous magnesium isotopic composition of the lower continental crust: A xenolith perspective. Geochemistry, Geophysics, Geosystems 14, 3844-3856.
; Li et al., 2014Li, W.Y., Teng, F.Z., Wing, B.A., Xiao, Y. (2014) Limited magnesium isotope fractionation during metamorphic dehydration in metapelites from the Onawa contact aureole, Maine. Geochemistry, Geophysics, Geosystems 15, 408-415.
; Wang et al., 2014bWang, S.-J., Teng, F.-Z., Li, S.-G., Hong, J.-A. (2014b) Magnesium isotopic systematics of mafic rocks during continental subduction. Geochimica et Cosmochimica Acta 143, 34-48.
). During metamorphism, the majority of Mg in rocks is inherited by newly formed minerals without significant loss, hence Mg isotope variations in the bulk metamorphic rocks are not directly related to metamorphic grade. Therefore, the heavy Mg isotopic compositions of the studied metapelites indicate that their protoliths were the residues of chemical weathering, from which light Mg isotopes had been progressively leached to the hydrosphere (Tipper et al., 2006Tipper, E.T., Galy, A., Gaillardet, J., Bickle, M.J., Elderfield, H., Carder, E.A. (2006) The magnesium isotope budget of the modern ocean: Constraints from riverine magnesium isotope ratios. Earth and Planetary Science Letters 250, 241-253.
; Teng et al., 2010bTeng, F.-Z., Li, W.-Y., Rudnick, R.L., Gardner, L.R. (2010b) Contrasting lithium and magnesium isotope fractionation during continental weathering. Earth and Planetary Science Letters 300, 63-71.
).The Mg budget in metapelites is exclusively controlled by biotite and garnet, which is opposite to other isotopic systems (such as Sr, Nd, Li, and O) that are hosted in other minerals in addition to biotite and garnet. Due to the prograde reaction of biotite + sillimanite + plagioclase + quartz → garnet + K-feldspar + rutile + melt (Schnetger, 1994
Schnetger, B. (1994) Partial melting during the evolution of the amphibolite-to granulite-facies gneisses of the Ivrea Zone, northern Italy. Chemical Geology 113, 71-101.
; Luvizotto and Zack, 2009Luvizotto, G., Zack, T. (2009) Nb and Zr behavior in rutile during high-grade metamorphism and retrogression: an example from the Ivrea-Verbano Zone. Chemical Geology 261, 303-317.
), the mineralogy of metapelites changes from biotite-dominated at amphibolite-facies to garnet-dominated at granulite-facies (Fig. S-2). At the same time, both biotite and garnet δ26Mg values become more positive (Fig. S-2). The coherent garnet - biotite Mg isotopic variations mainly result from the fact that the partitioning of Mg and its isotopes between garnet and biotite proceeds in accordance with their modal abundance within a nearly closed system. For example, biotites dominate the Mg budget in amphibolite-facies pelites, and consequently biotite δ26Mg values are, as expected from mass balance, similar to the bulk-rock values, whereas garnet δ26Mg values are significantly lighter because of the coordination geometry controlled inter-mineral fractionation (Fig. 1). During prograde metamorphism, isotopically heavy Mg released from the breakdown of biotite is progressively incorporated into the garnet, driving garnets towards high δ26Mg, close to the bulk-rock values (Fig. 1), and simultaneously shifting residual biotites to more 26Mg enriched.The systematic variation of mineral Mg isotopic compositions during middle-lower crustal metamorphism indicates the possibility of Mg isotope fractionation during crustal melting. As temperature rises, metapelites in the deep continental crust undergo two stages of dehydration melting: first as muscovite, and second as biotite (e.g., Le Breton and Thompson, 1988
Le Breton, N., Thompson, A.B. (1988) Fluid-absent (dehydration) melting of biotite in metapelites in the early stages of crustal anatexis. Contributions to Mineralogy and Petrology 99, 226-237.
; Clemens, 2003Clemens, J. (2003) S-type granitic magmas—petrogenetic issues, models and evidence. Earth-Science Reviews 61, 1-18.
). Muscovite dehydration melting (e.g., muscovite + plagioclase + quartz → melt + K-feldspar + sillimanite + biotite) would not produce significant Mg isotope fractionation between melts and residues because minerals participating in the melting reactions have the same Mg coordination geometry and hence would display limited inter-mineral Mg isotope fractionations. By contrast, dehydration melting of biotite at higher temperatures as discussed above (e.g., biotite + sillimanite + plagioclase + quartz → garnet + K-feldspar + rutile + melt) is able to generate highly variable δ26Mg for the melt. Melting reaction consistent with the biotite dehydration melting experiment of Patiño Douce and Johnston, 1991Patiño Douce, A., Johnston, A.D. (1991) Phase equilibria and melt productivity in the pelitic system: implications for the origin of peraluminous granitoids and aluminous granulites. Contributions to Mineralogy and Petrology 107, 202-218.
is modelled to investigate the behaviour of Mg isotopes during crustal melting (Fig. S-3). With progressive melting, Mg isotopic compositions of residual biotite become heavier accompanied by the growth of peritectic garnet. Correspondingly, the resulting melts become isotopically heavier (Fig. S-3). Therefore, multiple pulses of granitic magmas generated from the same protolith could have δ26Mg values that vary by up to ~1 ‰ (Fig. S-3).Our results also have important implications on tracing intra-crustal differentiation by using Mg isotopes. The granulite-facies lower crustal rocks that have undergone a previous melt extraction would contain only small amounts of hydrous minerals (e.g., biotite and amphibole) coexisting with a garnet-dominated solid peritectic mineral assemblage (Fig. 3). As expected from the closed-system Mg isotopic evolution during metamorphism, these biotites and amphiboles would have heavier Mg isotopic compositions than those in the amphibolite-facies middle crust. Hence, dehydration melting of a granulite-facies source in the lower crust at high temperatures (>850 ºC) would generate melts that have higher δ26Mg values than those derived from dehydration melting of an amphibolite-facies source in the middle crust. Further studies of Mg isotopes in granites may help to fingerprint their source and melting mechanism in the deep continental crust.
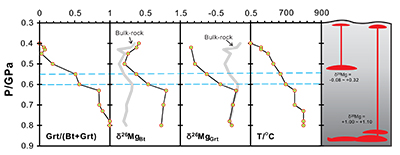
Figure 3 The variation of Grt/(Bt+Grt) ratio, δ26MgBt, δ26MgGrt and temperature as a function of pressure (crustal depth). Also shown is a cartoon illustrating the generation of granitic melts at different crustal depths. For those samples whose mineral separates are unavailable, Mg isotopic compositions of biotite and garnet are calculated based on the mineral modes and chemistries, assuming an inter-mineral Mg isotope fractionation of ~ 1.00 ‰. The mineral modes, compositions and temperatures are from Bea and Montero (1999)
Bea, F., Montero, P. (1999) Behavior of accessory phases and redistribution of Zr, REE, Y, Th, and U during metamorphism and partial melting of metapelites in the lower crust: an example from the Kinzigite Formation of Ivrea-Verbano, NW Italy. Geochimica et Cosmochimica Acta 63, 1133-1153.
.top
Acknowledgements
We would like to thank Roberta Rudnick for sharing samples, Aaron Brewer and Kwan-Nang Pang for thoughtful discussion, Ethan Baxter, Cin-Ty Lee and Bruce Watson for insightful comments. This work was financially supported by the national Science Foundation EAR-0838227, EAR-1056713, and EAR-1340160.
Editor: Bruce Watson
top
References
Barbero, L., Villaseca, C., Rogers, G., Brown, P. (1995) Geochemical and isotopic disequilibrium in crustal melting: an insight from the anatectic granitoids from Toledo, Spain. Journal of Geophysical Research: Solid Earth 100, 15745-15765.

Consequently, the elemental and isotopic diversity of granitoids results from a combination of protolith heterogeneities, melting reaction patterns, melt extraction and ascending processes (Barbero et al., 1995; Bea, 1996; Patiño Douce, 1999; Brown, 2007; Stevens et al., 2007; Clemens and Stevens, 2012; Lee and Morton, 2015).
View in article
Bea, F. (1996) Controls on the trace element composition of crustal melts. Geological Society of America Special Papers 315, 33-41.

Consequently, the elemental and isotopic diversity of granitoids results from a combination of protolith heterogeneities, melting reaction patterns, melt extraction and ascending processes (Barbero et al., 1995; Bea, 1996; Patiño Douce, 1999; Brown, 2007; Stevens et al., 2007; Clemens and Stevens, 2012; Lee and Morton, 2015).
View in article
Bea, F., Montero, P. (1999) Behavior of accessory phases and redistribution of Zr, REE, Y, Th, and U during metamorphism and partial melting of metapelites in the lower crust: an example from the Kinzigite Formation of Ivrea-Verbano, NW Italy. Geochimica et Cosmochimica Acta 63, 1133-1153.

Here, we present Mg isotope data for 12 well-characterised metapelites and 13 mineral separates (biotite and garnet) therein from the Ivrea Zone, NW Italy (Bea and Montero, 1999; Qiu et al., 2011).
View in article
The metamorphic condition for these metapelites ranges from ca. 4 kbar at 500 ºC to ca.10 ~ 12 kbar at >900 ºC (Henk et al., 1997; Bea and Montero, 1999; Luvizotto and Zack, 2009; Redler et al., 2011).
View in article
The metapelites in the Ivrea Zone are divided into three zones from low to high metamorphic grade based on the biotite/garnet ratio (Bea and Montero, 1999): the kinzigite zone that corresponds to metapelites with modal biotite/garnet >2, the transition zone in which the modal biotite/garnet is between 0.5 and 2, and the stronalite zone where modal biotite/garnet is <0.5 (Fig. S-1).
View in article
Figure 2 [...] The temperatures for the studied metapelites, estimated using biotite-garnet Mg-Fe exchange geothermometer, are from Bea and Montero (1999).
View in article
Figure 3 [...] The mineral modes, compositions and temperatures are from Bea and Montero (1999).
View in article
Bertolani, M., Garuti, G. (1970) Aspetti petrografici della formazione basica Ivrea-Verbano in Val Sessera (Vercelli). Rendiconti Societa Italiana di Mineralogia e Petrografia 32, 434-474.

This reaction proceeds until the biotite is almost entirely consumed in granulite-facies metapelites (Bertolani and Garuti, 1970; Schmid and Wood, 1976).
View in article
Brown, M. (2007) Crustal melting and melt extraction, ascent and emplacement in orogens: mechanisms and consequences. Journal of the Geological Society 164, 709-730.

Consequently, the elemental and isotopic diversity of granitoids results from a combination of protolith heterogeneities, melting reaction patterns, melt extraction and ascending processes (Barbero et al., 1995; Bea, 1996; Patiño Douce, 1999; Brown, 2007; Stevens et al., 2007; Clemens and Stevens, 2012; Lee and Morton, 2015).
View in article
Clemens, J. (2003) S-type granitic magmas—petrogenetic issues, models and evidence. Earth-Science Reviews 61, 1-18.

As temperature rises, metapelites in the deep continental crust undergo two stages of dehydration melting: first as muscovite, and second as biotite (e.g., Le Breton and Thompson, 1988; Clemens, 2003).
View in article
Clemens, J., Vielzeuf, D. (1987) Constraints on melting and magma production in the crust. Earth and Planetary Science Letters 86, 287-306.

Fluid-absent incongruent melting of hydrous minerals such as muscovite, biotite, and amphibole that takes place in the continental crust give rise to large volumes of granitoids (Clemens and Vielzeuf, 1987; Clemens and Watkins, 2001).
View in article
Clemens, J., Watkins, J. (2001) The fluid regime of high-temperature metamorphism during granitoid magma genesis. Contributions to Mineralogy and Petrology 140, 600-606.

Fluid-absent incongruent melting of hydrous minerals such as muscovite, biotite, and amphibole that takes place in the continental crust give rise to large volumes of granitoids (Clemens and Vielzeuf, 1987; Clemens and Watkins, 2001).
View in article
Clemens, J., Stevens, G. (2012) What controls chemical variation in granitic magmas? Lithos 134, 317-329.

Consequently, the elemental and isotopic diversity of granitoids results from a combination of protolith heterogeneities, melting reaction patterns, melt extraction and ascending processes (Barbero et al., 1995; Bea, 1996; Patiño Douce, 1999; Brown, 2007; Stevens et al., 2007; Clemens and Stevens, 2012; Lee and Morton, 2015).
View in article
Henk, A., Franz, L., Teufel, S., Oncken, O. (1997) Magmatic underplating, extension, and crustal reequilibration: insights from a cross-section through the Ivrea Zone and Strona-Ceneri Zone, Northern Italy. The Journal of Geology 105, 367-378.

The metamorphic condition for these metapelites ranges from ca. 4 kbar at 500 ºC to ca.10 ~ 12 kbar at >900 ºC (Henk et al., 1997; Bea and Montero, 1999; Luvizotto and Zack, 2009; Redler et al., 2011).
View in article
Huang, F., Chen, L., Wu, Z., Wang, W. (2013) First-principles calculations of equilibrium Mg isotope fractionations between garnet, clinopyroxene, orthopyroxene, and olivine: Implications for Mg isotope thermometry. Earth and Planetary Science Letters 367, 61-70.

This fractionation equation is very similar to those previously reported for garnet-clinopyroxene pairs from eclogites (Fig. 2) (Li et al., 2011; Wang et al., 2012, 2014a,b; Huang et al., 2013), which is reasonable since the Mg coordination geometry in biotite is the same as in clinopyroxene.
View in article
The relatively high scatter of data may reflect an additional but small effect from pressure, as first-principles calculations suggest that the equilibrium inter-mineral Mg isotope fractionation is also pressure-dependent; i.e. the degree of fractionation is suppressed as pressure decreases (Huang et al., 2013).
View in article
Figure 2 [...] Empirically and theoretically determined equilibrium equations of clinopyroxene-garnet Mg isotope fractionation are also shown for comparison. ① is an empirical equilibrium fractionation equation of Δ26Mgclinpyroxene–garnet = 0.83 × 106/T2 from Li et al. (2011); ② is also an empirical equilibrium fractionation equation of Δ26Mgclinpyroxene–garnet = 0.86 × 106/T2 from Wang et al. (2012); ③ and ④ represent the theoretically determined equilibrium fractionation equations at pressures of 0 and 10 kbar (Huang et al., 2013 respectively.
View in article
Le Breton, N., Thompson, A.B. (1988) Fluid-absent (dehydration) melting of biotite in metapelites in the early stages of crustal anatexis. Contributions to Mineralogy and Petrology 99, 226-237.

Pelitic rocks containing abundant mica minerals are the most fertile lithologies in the deep (middle and lower) continental crust, and can produce as much as 50 vol.% melt as the temperature rises above 700 ºC (Le Breton and Thompson, 1988; Vielzeuf and Holloway, 1988).
View in article
As temperature rises, metapelites in the deep continental crust undergo two stages of dehydration melting: first as muscovite, and second as biotite (e.g., Le Breton and Thompson, 1988; Clemens, 2003).
View in article
Lee, C.-T.A., Morton, D.M. (2015) High silica granites: Terminal porosity and crystal settling in shallow magma chambers. Earth and Planetary Science Letters 409, 23-31.

Consequently, the elemental and isotopic diversity of granitoids results from a combination of protolith heterogeneities, melting reaction patterns, melt extraction and ascending processes (Barbero et al., 1995; Bea, 1996; Patiño Douce, 1999; Brown, 2007; Stevens et al., 2007; Clemens and Stevens, 2012; Lee and Morton, 2015).
View in article
Li, W.-Y., Teng, F.-Z., Ke, S., Rudnick, R.L., Gao, S., Wu, F.-Y., Chappell, B.W. (2010) Heterogeneous magnesium isotopic composition of the upper continental crust. Geochimica et Cosmochimica Acta 74, 6867-6884.

The δ26Mg values of global granites range from -0.45 to +0.44 ‰ (Shen et al., 2009; Li et al., 2010; Liu et al., 2010; Telus et al., 2012; Ling et al., 2013; Wang et al., 2014b), far exceeding that of normal mantle rocks (-0.25 ± 0.07 ‰; Teng et al., 2010a).
View in article
Figure 1 [...] The average value of bulk upper continental crust (δ26Mg = -0.22 ‰) is represented by the blue line (Li et al., 2010).
View in article
Li, W.-Y., Teng, F.-Z., Xiao, Y., Huang, J. (2011) High-temperature inter-mineral magnesium isotope fractionation in eclogite from the Dabie orogen, China. Earth and Planetary Science Letters 304, 224-230.

This fractionation equation is very similar to those previously reported for garnet-clinopyroxene pairs from eclogites (Fig. 2) (Li et al., 2011; Wang et al., 2012, 2014a,b; Huang et al., 2013), which is reasonable since the Mg coordination geometry in biotite is the same as in clinopyroxene.
View in article
Figure 2 [...] Empirically and theoretically determined equilibrium equations of clinopyroxene-garnet Mg isotope fractionation are also shown for comparison. ① is an empirical equilibrium fractionation equation of Δ26Mgclinpyroxene–garnet = 0.83 × 106/T2 from Li et al. (2011); ② is also an empirical equilibrium fractionation equation of Δ26Mgclinpyroxene–garnet = 0.86 × 106/T2 from Wang et al. (2012); ③ and ④ represent the theoretically determined equilibrium fractionation equations at pressures of 0 and 10 kbar (Huang et al., 2013 respectively.
View in article
Li, W.Y., Teng, F.Z., Wing, B.A., Xiao, Y. (2014) Limited magnesium isotope fractionation during metamorphic dehydration in metapelites from the Onawa contact aureole, Maine. Geochemistry, Geophysics, Geosystems 15, 408-415.

This conclusion is consistent with previous studies that show limited Mg isotope fractionation by either low- or high-temperature metamorphic dehydration (Teng et al., 2013; Li et al., 2014; Wang et al., 2014b).
View in article
Ling, M.-X., Li, Y., Ding, X., Teng, F.-Z., Fan, W., Xu, Y., Sun, W.-D. (2013) Destruction of the North China Craton induced by ridge subductions. The Journal of Geology 121, 197-213.

The δ26Mg values of global granites range from -0.45 to +0.44 ‰ (Shen et al., 2009; Li et al., 2010; Liu et al., 2010; Telus et al., 2012; Ling et al., 2013; Wang et al., 2014b), far exceeding that of normal mantle rocks (-0.25 ± 0.07 ‰; Teng et al., 2010a).
View in article
Liu, S.-A., Teng, F.-Z., He, Y., Ke, S., Li, S. (2010) Investigation of magnesium isotope fractionation during granite differentiation: implication for Mg isotopic composition of the continental crust. Earth and Planetary Science Letters 297, 646-654.

The δ26Mg values of global granites range from -0.45 to +0.44 ‰ (Shen et al., 2009; Li et al., 2010; Liu et al., 2010; Telus et al., 2012; Ling et al., 2013; Wang et al., 2014b), far exceeding that of normal mantle rocks (-0.25 ± 0.07 ‰; Teng et al., 2010a).
View in article
Luvizotto, G., Zack, T. (2009) Nb and Zr behavior in rutile during high-grade metamorphism and retrogression: an example from the Ivrea-Verbano Zone. Chemical Geology 261, 303-317.

The metamorphic condition for these metapelites ranges from ca. 4 kbar at 500 ºC to ca.10 ~ 12 kbar at >900 ºC (Henk et al., 1997; Bea and Montero, 1999; Luvizotto and Zack, 2009; Redler et al., 2011).
View in article
Due to the prograde reaction of biotite + sillimanite + plagioclase + quartz → garnet + K-feldspar + rutile + melt (Schnetger, 1994; Luvizotto and Zack, 2009), the mineralogy of metapelites changes from biotite-dominated at amphibolite-facies to garnet-dominated at granulite-facies (Fig. S-2).
View in article
Patiño Douce, A. (1999) What do experiments tell us about the relative contributions of crust and mantle to the origin of granitic magmas? Geological Society Special Publications 168, 55-75.

Consequently, the elemental and isotopic diversity of granitoids results from a combination of protolith heterogeneities, melting reaction patterns, melt extraction and ascending processes (Barbero et al., 1995; Bea, 1996; Patiño Douce, 1999; Brown, 2007; Stevens et al., 2007; Clemens and Stevens, 2012; Lee and Morton, 2015).
View in article
Patiño Douce, A., Johnston, A.D. (1991) Phase equilibria and melt productivity in the pelitic system: implications for the origin of peraluminous granitoids and aluminous granulites. Contributions to Mineralogy and Petrology 107, 202-218.

Melting reaction consistent with the biotite dehydration melting experiment of Patiño et al. (1991) is modelled to investigate the behaviour of Mg isotopes during crustal melting (Fig. S-3).
View in article
Qiu, L., Rudnick, R.L., McDonough, W.F., Bea, F. (2011) The behavior of lithium in amphibolite-to granulite-facies rocks of the Ivrea–Verbano Zone, NW Italy. Chemical Geology 289, 76-85.

Here, we present Mg isotope data for 12 well-characterised metapelites and 13 mineral separates (biotite and garnet) therein from the Ivrea Zone, NW Italy (Bea and Montero, 1999; Qiu et al., 2011).
View in article
Redler, C., Johnson, T., White, R., Kunz, B. (2011) Phase equilibrium constraints on a deep crustal metamorphic field gradient: metapelitic rocks from the Ivrea Zone (NW Italy). Journal of Metamorphic Geology 30, 235-254.

The metamorphic condition for these metapelites ranges from ca. 4 kbar at 500 ºC to ca.10 ~ 12 kbar at >900 ºC (Henk et al., 1997; Bea and Montero, 1999; Luvizotto and Zack, 2009; Redler et al., 2011).
View in article
Schmid, R., Wood, B. (1976) Phase relationships in granulitic metapelites from the Ivrea-Verbano Zone (Northern Italy). Contributions to Mineralogy and Petrology 54, 255-279.

This reaction proceeds until the biotite is almost entirely consumed in granulite-facies metapelites (Bertolani and Garuti, 1970; Schmid and Wood, 1976).
View in article
Schnetger, B. (1994) Partial melting during the evolution of the amphibolite-to granulite-facies gneisses of the Ivrea Zone, northern Italy. Chemical Geology 113, 71-101.

Due to the prograde reaction of biotite + sillimanite + plagioclase + quartz → garnet + K-feldspar + rutile + melt (Schnetger, 1994; Luvizotto and Zack, 2009), the mineralogy of metapelites changes from biotite-dominated at amphibolite-facies to garnet-dominated at granulite-facies (Fig. S-2).
View in article
Shen, B., Jacobsen, B., Lee, C.-T.A., Yin, Q.-Z., Morton, D.M. (2009) The Mg isotopic systematics of granitoids in continental arcs and implications for the role of chemical weathering in crust formation. Proceedings of the National Academy of Sciences 106, 20652-20657.

The δ26Mg values of global granites range from -0.45 to +0.44 ‰ (Shen et al., 2009; Li et al., 2010; Liu et al., 2010; Telus et al., 2012; Ling et al., 2013; Wang et al., 2014b), far exceeding that of normal mantle rocks (-0.25 ± 0.07 ‰; Teng et al., 2010a).
View in article
While protolith heterogeneity (i.e. sedimentary vs. igneous protoliths) partially explains this Mg isotopic variation (Shen et al., 2009), metamorphic overprints on potential granitoid sources, with concomitant changes of source mineralogy, may play an important, but poorly understood, role as well.
View in article
Stevens, G., Villaros, A., Moyen, J.-F. (2007) Selective peritectic garnet entrainment as the origin of geochemical diversity in S-type granites. Geology 35, 9-12.

Consequently, the elemental and isotopic diversity of granitoids results from a combination of protolith heterogeneities, melting reaction patterns, melt extraction and ascending processes (Barbero et al., 1995; Bea, 1996; Patiño Douce, 1999; Brown, 2007; Stevens et al., 2007; Clemens and Stevens, 2012; Lee and Morton, 2015).
View in article
Telus, M., Dauphas, N., Moynier, F., Tissot, F.L.H., Teng, F.-Z., Nabelek, P.I., Craddock, P.R., Groat, L.A. (2012) Iron, zinc, magnesium and uranium isotopic fractionation during continental crust differentiation: The tale from migmatites, granitoids, and pegmatites. Geochimica et Cosmochimica Acta 97, 247-265.

The δ26Mg values of global granites range from -0.45 to +0.44 ‰ (Shen et al., 2009; Li et al., 2010; Liu et al., 2010; Telus et al., 2012; Ling et al., 2013; Wang et al., 2014b), far exceeding that of normal mantle rocks (-0.25 ± 0.07 ‰; Teng et al., 2010a).
View in article
Teng, F.-Z., Li, W.-Y., Ke, S., Marty, B., Dauphas, N., Huang, S.-C., Wu, F.-Y., Pourmand, A. (2010a) Magnesium isotopic composition of the Earth and chondrites. Geochimica et Cosmochimica Acta 74, 4150-4166.

The δ26Mg values of global granites range from -0.45 to +0.44 ‰ (Shen et al., 2009; Li et al., 2010; Liu et al., 2010; Telus et al., 2012; Ling et al., 2013; Wang et al., 2014b), far exceeding that of normal mantle rocks (-0.25 ± 0.07 ‰; Teng et al., 2010a).
View in article
Teng, F.-Z., Li, W.-Y., Rudnick, R.L., Gardner, L.R. (2010b) Contrasting lithium and magnesium isotope fractionation during continental weathering. Earth and Planetary Science Letters 300, 63-71.

Therefore, the heavy Mg isotopic compositions of the studied metapelites indicate that their protoliths were the residues of chemical weathering, from which light Mg isotopes had been progressively leached to the hydrosphere (e.g., Tipper et al., 2006; Teng et al., 2010b).
View in article
Teng, F.Z., Yang, W., Rudnick, R.L., Hu, Y. (2013) Heterogeneous magnesium isotopic composition of the lower continental crust: A xenolith perspective. Geochemistry, Geophysics, Geosystems 14, 3844-3856.

This conclusion is consistent with previous studies that show limited Mg isotope fractionation by either low- or high-temperature metamorphic dehydration (Teng et al., 2013; Li et al., 2014; Wang et al., 2014b).
View in article
Tipper, E.T., Galy, A., Gaillardet, J., Bickle, M.J., Elderfield, H., Carder, E.A. (2006) The magnesium isotope budget of the modern ocean: Constraints from riverine magnesium isotope ratios. Earth and Planetary Science Letters 250, 241-253.

Therefore, the heavy Mg isotopic compositions of the studied metapelites indicate that their protoliths were the residues of chemical weathering, from which light Mg isotopes had been progressively leached to the hydrosphere (e.g., Tipper et al., 2006; Teng et al., 2010b).
View in article
Vielzeuf, D., Holloway, J.R. (1988) Experimental determination of the fluid-absent melting relations in the pelitic system. Contributions to Mineralogy and Petrology 98, 257-276.

Pelitic rocks containing abundant mica minerals are the most fertile lithologies in the deep (middle and lower) continental crust, and can produce as much as 50 vol.% melt as the temperature rises above 700 ºC (Le Breton and Thompson, 1988; Vielzeuf and Holloway, 1988).
View in article
Wang, S.-J., Teng, F.-Z., Williams, H.M., Li, S.-G. (2012) Magnesium isotopic variations in cratonic eclogites: Origins and implications. Earth and Planetary Science Letters 359, 219-226.

This fractionation equation is very similar to those previously reported for garnet-clinopyroxene pairs from eclogites (Fig. 2) (Li et al., 2011; Wang et al., 2012, 2014a,b; Huang et al., 2013), which is reasonable since the Mg coordination geometry in biotite is the same as in clinopyroxene.
View in article
Figure 2 [...] Empirically and theoretically determined equilibrium equations of clinopyroxene-garnet Mg isotope fractionation are also shown for comparison. ① is an empirical equilibrium fractionation equation of Δ26Mgclinpyroxene–garnet = 0.83 × 106/T2 from Li et al. (2011); ② is also an empirical equilibrium fractionation equation of Δ26Mgclinpyroxene–garnet = 0.86 × 106/T2 from Wang et al. (2012); ③ and ④ represent the theoretically determined equilibrium fractionation equations at pressures of 0 and 10 kbar (Huang et al., 2013 respectively.
View in article
Wang, S.-J., Teng, F.-Z., Li, S.-G. (2014a) Tracing carbonate-silicate interaction during subduction using magnesium and oxygen isotopes. Nature Communications 5, doi:10.1038/ncomms6328.

This fractionation equation is very similar to those previously reported for garnet-clinopyroxene pairs from eclogites (Fig. 2) (Li et al., 2011; Wang et al., 2012, 2014a,b; Huang et al., 2013), which is reasonable since the Mg coordination geometry in biotite is the same as in clinopyroxene.
View in article
Wang, S.-J., Teng, F.-Z., Li, S.-G., Hong, J.-A. (2014b) Magnesium isotopic systematics of mafic rocks during continental subduction. Geochimica et Cosmochimica Acta 143, 34-48.

The δ26Mg values of global granites range from -0.45 to +0.44 ‰ (Shen et al., 2009; Li et al., 2010; Liu et al., 2010; Telus et al., 2012; Ling et al., 2013; Wang et al., 2014b), far exceeding that of normal mantle rocks (-0.25 ± 0.07 ‰; Teng et al., 2010a).
View in article
This fractionation equation is very similar to those previously reported for garnet-clinopyroxene pairs from eclogites (Fig. 2) (Li et al., 2011; Wang et al., 2012, 2014a,b; Huang et al., 2013), which is reasonable since the Mg coordination geometry in biotite is the same as in clinopyroxene.
View in article
This conclusion is consistent with previous studies that show limited Mg isotope fractionation by either low- or high-temperature metamorphic dehydration (Teng et al., 2013; Li et al., 2014; Wang et al., 2014b).
View in article
Whalen, J.B., Currie, K.L., Chappell, B.W. (1987) A-type granites: geochemical characteristics, discrimination and petrogenesis. Contributions to Mineralogy and Petrology 95, 407-419.

Dry, granulitic residues after an early melt extraction are relatively refractory but may still be melted at higher temperatures (e.g., >830 ºC; Whalen et al., 1987). Consequently
View in article
Zingg, A. (1984) Development Stages of Ivrea Paragneiss (Southern Alps, Northern Italy). Fortschritte Der Mineralogie 62, 266-268.

The Ivrea Zone, NW Italy, represents an exposed section of the middle to lower crust and preserves a transition from amphibolite- to granulite-facies metapelites (Zingg, 1984).
View in article
top
Supplementary Information
Methods
Prior to sample dissolution, biotite and garnet grains were handpicked under a binocular microscope with extra care taken to avoid any alteration products during separation. The mineral separates were then ultrasonicated for three times with each in Milli-Q Water for 10 minutes.
Chemical procedures including sample dissolution, column chemistry, and instrumental analyses were carried out at the Isotope Laboratory of University of Washington, Seattle, USA. These mineral separates together with the rock powders were digested using a mixture of Optima-grade HF-HNO3-HCl acids. After complete dissolution, dried residues were taken up in 1N HNO3 ready for column chemistry. Detail procedures have been reported elsewhere (Teng et al., 2007
Teng, F.-Z., Wadhwa, M., Helz, R.T. (2007) Investigation of magnesium isotope fractionation during basalt differentiation: implications for a chondritic composition of the terrestrial mantle. Earth and Planetary Science Letters 261, 84-92.
, 2010Teng, F.-Z., Li, W.-Y., Ke, S., Marty, B., Dauphas, N., Huang, S.-C., Wu, F.-Y., Pourmand, A. (2010) Magnesium isotopic composition of the Earth and chondrites. Geochimica et Cosmochimica Acta 74, 4150-4166.
, 2015Teng, F.Z., Li, W.Y., Ke, S., Yang, W., Liu, S.A., Sedaghatpour, F., Wang, S.J., Huang, K.J., Hu, Y., Ling, M.X., Xiao, Y., Liu, X.M., Li, X.W., Gu, H.O., Sio, C., Wallace, D., Su, B.X., Zhao, L., Harrington, M., Brewer, A. (2015) Magnesium isotopic compositions of international geological reference materials. Geostandards and Geoanalytical Research doi: 10.1111/j.1751-908X.2014.00326.x.
; Yang et al., 2009Yang, W., Teng, F.-Z., Zhang, H.-F. (2009) Chondritic magnesium isotopic composition of the terrestrial mantle: a case study of peridotite xenoliths from the North China craton. Earth and Planetary Science Letters 288, 475-482.
; Teng and Yang, 2014Teng, F.Z., Yang, W. (2014) Comparison of factors affecting the accuracy of high‐precision magnesium isotope analysis by multi‐collector inductively coupled plasma mass spectrometry. Rapid Communications in Mass Spectrometry 28, 19-24.
), and only a brief description is given below.Chemical separation and purification of Mg were achieved by cation exchange chromatography with Bio-Rad AG50W-X8 resin in 1N HNO3 media. The same column procedure was performed twice in order to obtain the pure Mg recovery. The pure Mg solutions were then dried down, and re-dissolved in 3 % HNO3 ready for mass spectrometry. An additional chromatographic step was processed for some garnets containing high Mn concentrations, using BioRad AG50W-X8 200-400 mesh resin in 0.5N HCl-95 % acetone media (Bizzarro et al., 2011
Bizzarro, M., Paton, C., Larsen, K., Schiller, M., Trinquier, A., Ulfbeck, D. (2011) High-precision Mg-isotope measurements of terrestrial and extraterrestrial material by HR-MC-ICPMS-implications for the relative and absolute Mg-isotope composition of the bulk silicate Earth. Journal of Analytical Atomic Spectrometry 26, 565-577.
).The Mg isotopic compositions were analysed by the sample-standard bracketing method using a Nu Plasma MC-ICPMS at low resolution mode (Teng and Yang, 2014
Teng, F.Z., Yang, W. (2014) Comparison of factors affecting the accuracy of high‐precision magnesium isotope analysis by multi‐collector inductively coupled plasma mass spectrometry. Rapid Communications in Mass Spectrometry 28, 19-24.
). Each batch of sample analysis contains at least one well-characterised standard. Sample solution was repeated on ratio measurements for >4 times within a session. The long-term precision is better than ±0.07 ‰ (2SD) for the 26Mg/24Mg ratio. Magnesium isotopic results are reported in the conventional δ notation in per mille relative to DSM-3:Eq. S-1

Figure S-1 Sketch map showing the location of Ivrea Zone, NW Italy (modified from Bea and Montero, 1999
Bea, F., Montero, P. (1999) Behavior of accessory phases and redistribution of Zr, REE, Y, Th, and U during metamorphism and partial melting of metapelites in the lower crust: an example from the Kinzigite Formation of Ivrea-Verbano, NW Italy. Geochimica et Cosmochimica Acta 63, 1133-1153.
). The labels indicate the samples listed in Table S-1.
Figure S-2 Plots of biotite δ26Mg versus biotite mode (a) and garnet δ26Mg versus garnet mode (b) in metapelites. The modal abundances of biotite and garnet are from Bea and Montero (1999)
Bea, F., Montero, P. (1999) Behavior of accessory phases and redistribution of Zr, REE, Y, Th, and U during metamorphism and partial melting of metapelites in the lower crust: an example from the Kinzigite Formation of Ivrea-Verbano, NW Italy. Geochimica et Cosmochimica Acta 63, 1133-1153.
. The Mg isotopic data are reported in Table S-1.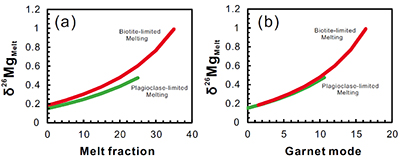
Figure S-3 Modelling showing the evolution of melt δ26Mg values as a function of melt fraction (a) and peritectic garnet mode (b) during progressive biotite dehydration melting. The stoichiometry of biotite dehydration melting reaction is: 1 biotite + 0.55 plagioclase + 0.14 aluminosilicate + 0.76 quartz = 1.72 melt + 0.73 garnet (Patiño Douce and Johnston, 1991
Patiño Douce, A., Johnston, A.D. (1991) Phase equilibria and melt productivity in the pelitic system: implications for the origin of peraluminous granitoids and aluminous granulites. Contributions to Mineralogy and Petrology 107, 202-218.
). Biotite-limited melting and plagioclase-limited melting indicate that melting terminates when biotite and plagioclase in the source is exhausted, respectively. In the modelling, the protoliths are assumed to have δ26Mg value of +0.15 ‰ and MgO of 3 wt.%; the partition coefficient of Mg between biotite and garnet (Dbiotite/garnet) is roughly assumed to be 2 based on the mineral compositions (Bea and Montero, 1999Bea, F., Montero, P. (1999) Behavior of accessory phases and redistribution of Zr, REE, Y, Th, and U during metamorphism and partial melting of metapelites in the lower crust: an example from the Kinzigite Formation of Ivrea-Verbano, NW Italy. Geochimica et Cosmochimica Acta 63, 1133-1153.
). The mineral modes in P1 (biotite : plagioclase : aluminosilicate : quartz : others = 0.26 : 0.09 : 0.11 : 0.49 : 0.05) and P2 (biotite : plagioclase : aluminosilicate : quartz : garnet: others = 0.22 : 0.17 : 0.04 : 0.52 : 0.015 : 0.035) are taken as examples to model plagioclase-limited melting and biotite-limited melting, respectively. The inter-mineral Mg isotope fractionation between biotite and garnet is assumed to be ~1.00 ‰.Table S-1 Magnesium isotopic compositions (‰) of bulk rocks and mineral separates for the metapelites from the Ivrea Zone, NW Italy.
Sample | Bulk/Mineral | δ26Mg | 2SD | δ25Mg | 2SD | Grt/(Bt+Grt)* | P (GPa)* | T(ºC)* |
Kinzigite Zone | ||||||||
P1 | Bulk rock | +0.205 | 0.070 | +0.100 | 0.068 | 0 | 0.40 | 500 |
Replicate a | +0.201 | 0.048 | +0.141 | 0.044 | ||||
average | +0.202 | 0.040 | +0.129 | 0.037 | ||||
Biotite | +0.329 | 0.076 | +0.189 | 0.052 | ||||
Replicate | +0.275 | 0.076 | +0.156 | 0.052 | ||||
average | +0.302 | 0.054 | +0.173 | 0.037 | ||||
P2 | Bulk rock | +0.110 | 0.048 | +0.075 | 0.044 | 0.064 | 0.42 | 560 |
Biotite | +0.255 | 0.073 | +0.132 | 0.074 | ||||
Garnet | -1.194 | 0.093 | -0.631 | 0.094 | ||||
Duplicate b | -1.258 | 0.064 | -0.614 | 0.049 | ||||
Replicate | -1.197 | 0.064 | -0.615 | 0.050 | ||||
Duplicate | -1.225 | 0.098 | -0.598 | 0.098 | ||||
average | -1.221 | 0.039 | -0.620 | 0.031 | ||||
P3 | Bulk rock | -0.265 | 0.048 | -0.155 | 0.044 | 0.078 | 0.43 | 560 |
P4 | Bulk rock | -0.073 | 0.048 | -0.048 | 0.044 | 0.029 | 0.45 | 600 |
Biotite | +0.017 | 0.073 | +0.049 | 0.074 | ||||
P5 | Bulk rock | -0.234 | 0.048 | -0.124 | 0.044 | 0.187 | 0.50 | 630 |
Biotite | -0.082 | 0.073 | -0.054 | 0.074 | ||||
Duplicate | -0.072 | 0.064 | -0.078 | 0.049 | ||||
average | -0.076 | 0.048 | -0.071 | 0.041 | ||||
Garnet | -1.086 | 0.073 | -0.624 | 0.074 | ||||
Duplicate | -1.108 | 0.064 | -0.605 | 0.049 | ||||
average | -1.099 | 0.048 | -0.611 | 0.041 | ||||
Transition Zone | ||||||||
P6 | Bulk rock | -0.099 | 0.048 | -0.046 | 0.044 | 0.511 | 0.55 | 670 |
Replicate | -0.105 | 0.064 | -0.036 | 0.054 | ||||
average | -0.101 | 0.038 | -0.042 | 0.034 | ||||
P7 | Bulk rock | +0.138 | 0.048 | +0.090 | 0.044 | 0.566 | 0.60 | 700 |
Biotite | +0.608 | 0.073 | +0.323 | 0.074 | ||||
Duplicate | +0.567 | 0.076 | +0.316 | 0.052 | ||||
Duplicate | +0.555 | 0.064 | +0.290 | 0.049 | ||||
average | +0.575 | 0.041 | +0.306 | 0.032 | ||||
Garnet | -0.360 | 0.076 | -0.170 | 0.052 | ||||
Duplicate | -0.330 | 0.064 | -0.171 | 0.049 | ||||
average | -0.343 | 0.049 | -0.170 | 0.036 | ||||
Stronalite Zone | ||||||||
P8 | Bulk rock | +0.118 | 0.048 | +0.048 | 0.044 | 0.845 | 0.63 | 750 |
Biotite | +1.100 | 0.073 | +0.579 | 0.074 | ||||
Garnet | +0.090 | 0.073 | +0.077 | 0.074 | ||||
Duplicate | +0.098 | 0.064 | +0.026 | 0.049 | ||||
average | +0.095 | 0.048 | +0.042 | 0.041 | ||||
P9 | Bulk rock | -0.031 | 0.052 | +0.014 | 0.048 | 0.850 | 0.70 | 750 |
Biotite | +1.020 | 0.073 | +0.560 | 0.074 | ||||
Duplicate | +0.983 | 0.064 | +0.518 | 0.041 | ||||
average | +0.999 | 0.048 | +0.531 | 0.048 | ||||
Garnet | -0.005 | 0.073 | +0.009 | 0.074 | ||||
Duplicate | -0.043 | 0.064 | +0.001 | 0.049 | ||||
average | -0.026 | 0.048 | +0.003 | 0.041 | ||||
P10 | Bulk rock | -0.022 | 0.052 | +0.025 | 0.048 | 0.895 | 0.73 | 800 |
P11 | Bulk rock | -0.105 | 0.052 | -0.049 | 0.048 | 1.000 | 0.78 | 800 |
P12 | Bulk rock | -0.035 | 0.052 | -0.002 | 0.048 | 0.994 | 0.80 | 800 |
* Data are from Bea and Montero (1999) Bea, F., Montero, P. (1999) Behavior of accessory phases and redistribution of Zr, REE, Y, Th, and U during metamorphism and partial melting of metapelites in the lower crust: an example from the Kinzigite Formation of Ivrea-Verbano, NW Italy. Geochimica et Cosmochimica Acta 63, 1133-1153.
a Repeat sample dissolution, column chemistry and instrumental analysis.
b Repeat measurement of Mg isotopic ratios on the same solution.
2SD 2 times the standard deviation of the population of n (n > 20) repeated measurements of the standards during an analytical session.
Supplementary Information References
Bea, F., Montero, P. (1999) Behavior of accessory phases and redistribution of Zr, REE, Y, Th, and U during metamorphism and partial melting of metapelites in the lower crust: an example from the Kinzigite Formation of Ivrea-Verbano, NW Italy. Geochimica et Cosmochimica Acta 63, 1133-1153.

Figure S-1 Sketch map showing the location of Ivrea Zone, NW Italy (modified from Bea and Montero, 1999).
View in supplementary information
Figure S-2 [...] The modal abundances of biotite and garnet are from Bea and Montero (1999).
View in supplementary information
Figure S-3 [...] In the modelling, the protoliths are assumed to have δ26Mg value of +0.15 ‰ and MgO of 3 wt.%; the partition coefficient of Mg between biotite and garnet (Dbiotite/garnet) is roughly assumed to be 2 based on the mineral compositions (Bea and Montero, 1999).
View in supplementary information
Table S-1 [...] Data are from Bea and Montero (1999).
View in supplementary information
Bizzarro, M., Paton, C., Larsen, K., Schiller, M., Trinquier, A., Ulfbeck, D. (2011) High-precision Mg-isotope measurements of terrestrial and extraterrestrial material by HR-MC-ICPMS-implications for the relative and absolute Mg-isotope composition of the bulk silicate Earth. Journal of Analytical Atomic Spectrometry 26, 565-577.

An additional chromatographic step was processed for some garnets containing high Mn concentrations, using BioRad AG50W-X8 200-400 mesh resin in 0.5N HCl-95 % acetone media (Bizzarro et al., 2011).
View in supplementary information
Patiño Douce, A., Johnston, A.D. (1991) Phase equilibria and melt productivity in the pelitic system: implications for the origin of peraluminous granitoids and aluminous granulites. Contributions to Mineralogy and Petrology 107, 202-218.

Figure S-3 [...] The stoichiometry of biotite dehydration melting reaction is: 1 biotite + 0.55 plagioclase + 0.14 aluminosilicate + 0.76 quartz = 1.72 melt + 0.73 garnet (Patiño Douce and Johnston, 1991).
View in supplementary information
Teng, F.Z., Yang, W. (2014) Comparison of factors affecting the accuracy of high‐precision magnesium isotope analysis by multi‐collector inductively coupled plasma mass spectrometry. Rapid Communications in Mass Spectrometry 28, 19-24.

Detail procedures have been reported elsewhere (Teng et al., 2007, 2010, 2015; Yang et al., 2009; Teng and Yang, 2014), and only a brief description is given below.
View in supplementary information
The Mg isotopic compositions were analysed by the sample-standard bracketing method using a Nu Plasma MC-ICPMS at low resolution mode (Teng and Yang, 2014).
View in supplementary information
Teng, F.-Z., Wadhwa, M., Helz, R.T. (2007) Investigation of magnesium isotope fractionation during basalt differentiation: implications for a chondritic composition of the terrestrial mantle. Earth and Planetary Science Letters 261, 84-92.

Detail procedures have been reported elsewhere (Teng et al., 2007, 2010, 2015; Yang et al., 2009; Teng and Yang, 2014), and only a brief description is given below.
View in supplementary information
Teng, F.-Z., Li, W.-Y., Ke, S., Marty, B., Dauphas, N., Huang, S.-C., Wu, F.-Y., Pourmand, A. (2010) Magnesium isotopic composition of the Earth and chondrites. Geochimica et Cosmochimica Acta 74, 4150-4166.

Detail procedures have been reported elsewhere (Teng et al., 2007, 2010, 2015; Yang et al., 2009; Teng and Yang, 2014), and only a brief description is given below.
View in supplementary information
Teng, F.Z., Li, W.Y., Ke, S., Yang, W., Liu, S.A., Sedaghatpour, F., Wang, S.J., Huang, K.J., Hu, Y., Ling, M.X., Xiao, Y., Liu, X.M., Li, X.W., Gu, H.O., Sio, C., Wallace, D., Su, B.X., Zhao, L., Harrington, M., Brewer, A. (2015) Magnesium isotopic compositions of international geological reference materials. Geostandards and Geoanalytical Research doi: 10.1111/j.1751-908X.2014.00326.x.

Detail procedures have been reported elsewhere (Teng et al., 2007, 2010, 2015; Yang et al., 2009; Teng and Yang, 2014), and only a brief description is given below.
View in supplementary information
Yang, W., Teng, F.-Z., Zhang, H.-F. (2009) Chondritic magnesium isotopic composition of the terrestrial mantle: a case study of peridotite xenoliths from the North China craton. Earth and Planetary Science Letters 288, 475-482.

Detail procedures have been reported elsewhere (Teng et al., 2007, 2010, 2015; Yang et al., 2009; Teng and Yang, 2014), and only a brief description is given below.
View in supplementary information
Figures and Tables

Figure 1 Magnesium isotopic compositions versus metamorphic grade for the bulk metapelites, biotites and garnets. The average value of bulk upper continental crust (δ26Mg = -0.22 ‰) is represented by the blue line (Li et al., 2010). Data are reported in Table S-1.

Figure 2 Magnesium isotope fractionation between biotite and garnet (Δ26MgBt-Grt = δ26MgBt - δ26MgGrt) as a function of 1/T2. Empirically and theoretically determined equilibrium equations of clinopyroxene-garnet Mg isotope fractionation are also shown for comparison. ① is an empirical equilibrium fractionation equation of Δ26Mgclinopyroxene–garnet = 0.83 × 106/T2 from Li et al. (2011); ② is also an empirical equilibrium fractionation equation of Δ26Mgclinopyroxene–garnet = 0.86 × 106/T2 from Wang et al. (2012); ③ and ④ represent the theoretically determined equilibrium fractionation equations at pressures of 0 and 10 kbar (Huang et al., 2013), respectively. The temperatures for the studied metapelites, estimated using biotite-garnet Mg-Fe exchange geothermometer, are from Bea and Montero (1999). Data are reported in Table S-1.
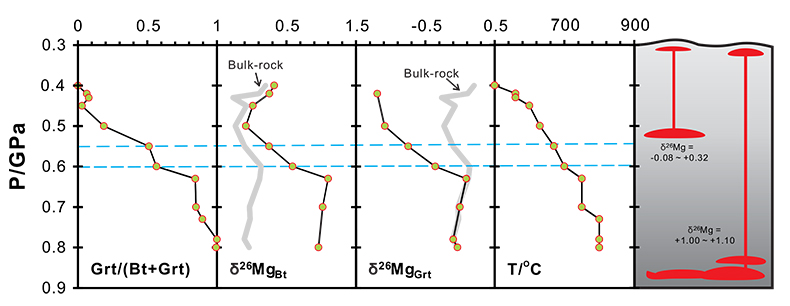
Figure 3 The variation of Grt/(Bt+Grt) ratio, δ26MgBt, δ26MgGrt and temperature as a function of pressure (crustal depth). Also shown is a cartoon illustrating the generation of granitic melts at different crustal depths. For those samples whose mineral separates are unavailable, Mg isotopic compositions of biotite and garnet are calculated based on the mineral modes and chemistries, assuming an inter-mineral Mg isotope fractionation of ~ 1.00 ‰. The mineral modes, compositions and temperatures are from Bea and Montero (1999).
Back to article
Supplementary Figures and Tables

Figure S-1 Sketch map showing the location of Ivrea Zone, NW Italy (modified from Bea and Montero, 1999). The labels indicate the samples listed in Table S-1.
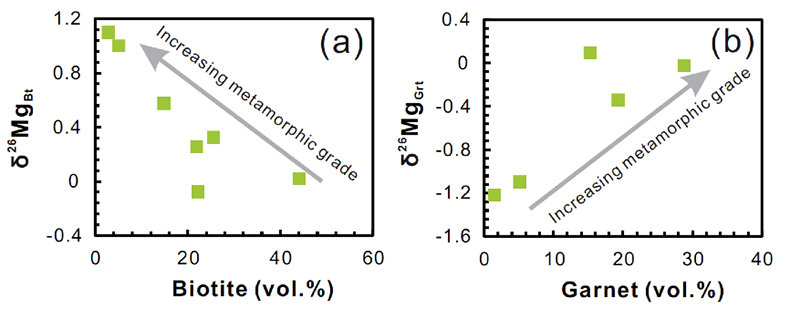
Figure S-2 Plots of biotite δ26Mg versus biotite mode (a) and garnet δ26Mg versus garnet mode (b) in metapelites. The modal abundances of biotite and garnet are from Bea and Montero (1999). The Mg isotopic data are reported in Table S-1.

Figure S-3 Modelling showing the evolution of melt δ26Mg values as a function of melt fraction (a) and peritectic garnet mode (b) during progressive biotite dehydration melting. The stoichiometry of biotite dehydration melting reaction is: 1 biotite + 0.55 plagioclase + 0.14 aluminosilicate + 0.76 quartz = 1.72 melt + 0.73 garnet (Patiño Douce and Johnston, 1991). Biotite-limited melting and plagioclase-limited melting indicate that melting terminates when biotite and plagioclase in the source is exhausted, respectively. In the modelling, the protoliths are assumed to have δ26Mg value of +0.15 ‰ and MgO of 3 wt.%; the partition coefficient of Mg between biotite and garnet (Dbiotite/garnet) is roughly assumed to be 2 based on the mineral compositions (Bea and Montero, 1999). The mineral modes in P1 (biotite : plagioclase : aluminosilicate : quartz : others = 0.26 : 0.09 : 0.11 : 0.49 : 0.05) and P2 (biotite : plagioclase : aluminosilicate : quartz : garnet: others = 0.22 : 0.17 : 0.04 : 0.52 : 0.015 : 0.035) are taken as examples to model plagioclase-limited melting and biotite-limited melting, respectively. The inter-mineral Mg isotope fractionation between biotite and garnet is assumed to be ~1.00 ‰.
Table S-1 Magnesium isotopic compositions (‰) of bulk rocks and mineral separates for the metapelites from the Ivrea Zone, NW Italy.
Sample | Bulk/Mineral | δ26Mg | 2SD | δ25Mg | 2SD | Grt/(Bt+Grt)* | P (GPa)* | T(ºC)* |
Kinzigite Zone | ||||||||
P1 | Bulk rock | +0.205 | 0.070 | +0.100 | 0.068 | 0 | 0.40 | 500 |
Replicate a | +0.201 | 0.048 | +0.141 | 0.044 | ||||
average | +0.202 | 0.040 | +0.129 | 0.037 | ||||
Biotite | +0.329 | 0.076 | +0.189 | 0.052 | ||||
Replicate | +0.275 | 0.076 | +0.156 | 0.052 | ||||
average | +0.302 | 0.054 | +0.173 | 0.037 | ||||
P2 | Bulk rock | +0.110 | 0.048 | +0.075 | 0.044 | 0.064 | 0.42 | 560 |
Biotite | +0.255 | 0.073 | +0.132 | 0.074 | ||||
Garnet | -1.194 | 0.093 | -0.631 | 0.094 | ||||
Duplicate b | -1.258 | 0.064 | -0.614 | 0.049 | ||||
Replicate | -1.197 | 0.064 | -0.615 | 0.050 | ||||
Duplicate | -1.225 | 0.098 | -0.598 | 0.098 | ||||
average | -1.221 | 0.039 | -0.620 | 0.031 | ||||
P3 | Bulk rock | -0.265 | 0.048 | -0.155 | 0.044 | 0.078 | 0.43 | 560 |
P4 | Bulk rock | -0.073 | 0.048 | -0.048 | 0.044 | 0.029 | 0.45 | 600 |
Biotite | +0.017 | 0.073 | +0.049 | 0.074 | ||||
P5 | Bulk rock | -0.234 | 0.048 | -0.124 | 0.044 | 0.187 | 0.50 | 630 |
Biotite | -0.082 | 0.073 | -0.054 | 0.074 | ||||
Duplicate | -0.072 | 0.064 | -0.078 | 0.049 | ||||
average | -0.076 | 0.048 | -0.071 | 0.041 | ||||
Garnet | -1.086 | 0.073 | -0.624 | 0.074 | ||||
Duplicate | -1.108 | 0.064 | -0.605 | 0.049 | ||||
average | -1.099 | 0.048 | -0.611 | 0.041 | ||||
Transition Zone | ||||||||
P6 | Bulk rock | -0.099 | 0.048 | -0.046 | 0.044 | 0.511 | 0.55 | 670 |
Replicate | -0.105 | 0.064 | -0.036 | 0.054 | ||||
average | -0.101 | 0.038 | -0.042 | 0.034 | ||||
P7 | Bulk rock | +0.138 | 0.048 | +0.090 | 0.044 | 0.566 | 0.60 | 700 |
Biotite | +0.608 | 0.073 | +0.323 | 0.074 | ||||
Duplicate | +0.567 | 0.076 | +0.316 | 0.052 | ||||
Duplicate | +0.555 | 0.064 | +0.290 | 0.049 | ||||
average | +0.575 | 0.041 | +0.306 | 0.032 | ||||
Garnet | -0.360 | 0.076 | -0.170 | 0.052 | ||||
Duplicate | -0.330 | 0.064 | -0.171 | 0.049 | ||||
average | -0.343 | 0.049 | -0.170 | 0.036 | ||||
Stronalite Zone | ||||||||
P8 | Bulk rock | +0.118 | 0.048 | +0.048 | 0.044 | 0.845 | 0.63 | 750 |
Biotite | +1.100 | 0.073 | +0.579 | 0.074 | ||||
Garnet | +0.090 | 0.073 | +0.077 | 0.074 | ||||
Duplicate | +0.098 | 0.064 | +0.026 | 0.049 | ||||
average | +0.095 | 0.048 | +0.042 | 0.041 | ||||
P9 | Bulk rock | -0.031 | 0.052 | +0.014 | 0.048 | 0.850 | 0.70 | 750 |
Biotite | +1.020 | 0.073 | +0.560 | 0.074 | ||||
Duplicate | +0.983 | 0.064 | +0.518 | 0.041 | ||||
average | +0.999 | 0.048 | +0.531 | 0.048 | ||||
Garnet | -0.005 | 0.073 | +0.009 | 0.074 | ||||
Duplicate | -0.043 | 0.064 | +0.001 | 0.049 | ||||
average | -0.026 | 0.048 | +0.003 | 0.041 | ||||
P10 | Bulk rock | -0.022 | 0.052 | +0.025 | 0.048 | 0.895 | 0.73 | 800 |
P11 | Bulk rock | -0.105 | 0.052 | -0.049 | 0.048 | 1.000 | 0.78 | 800 |
P12 | Bulk rock | -0.035 | 0.052 | -0.002 | 0.048 | 0.994 | 0.80 | 800 |
* Data are from Bea and Montero (1999).
a Repeat sample dissolution, column chemistry and instrumental analysis.
b Repeat measurement of Mg isotopic ratios on the same solution.
2SD 2 times the standard deviation of the population of n (n > 20) repeated measurements of the standards during an analytical session.