CO2-fluxing collapses metal mobility in magmatic vapour
Affiliations | Corresponding Author | Cite as- Share this article
-
Article views:11,749Cumulative count of HTML views and PDF downloads.
- Download Citation
- Rights & Permissions
Abstract
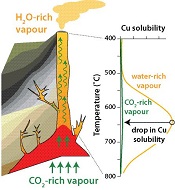
Figures and Tables
![]() Figure 1 Impact of CO2-fluxing on Cu solubility in a volcanic conduit filled with vapour. Fluxing deep-sourced CO2-rich vapour through shallower, water-dominated vapour reduces Cu solubility as the water partial pressure is reduced. Cu contents are significant for the water-dominated vapour. Assuming upward flow, Cu is dissolved from wall rocks to 2.2 km, and subsequently deposited. In contrast, contents in the CO2-rich vapour are negligible. Therefore, a single pulse of CO2–rich vapour will deposit essentially all Cu in the water-vapour, with deposition at all depths and all temperatures. | ![]() Table 1 Predicted Cu solubility in vapours of varying pH2O and pHCl with fO2 and Cu buffered by NiO-Ni and CuFeS2-FeS2, respectively. | ![]() Figure 2 Progressive Cu enrichment in a fracture network intermittently pulsed with CO2-rich vapour in its centre. (a) Schematic section showing Cu deposition on fracture walls followed by partial re-dissolution after each pulse, except for fractures sealed by precipitation. (b) Progressive enrichment of Cu assuming partial Cu re-dissolution, and fluxing of 5 vol. % of the fracture network. Cu is sourced from the 95 vol. % of the network filled with water-rich vapour. |
Figure 1 | Table 1 | Figure 2 |
Supplementary Figures and Tables
![]() Table S-1 Gas compositions used in the calculations and calculated Cu solubility. |
Table S-1 |
top
Introduction
Aqueous vapour is increasingly recognised as an important agent of element mobility in the crust (Hedenquist and Lowenstern, 1994
Hedenquist, J.W., Lowenstern, J.B. (1994) The role of magmas in the formation of hydrothermal ore deposits. Nature 370, 519–527.
; Pokrovski et al., 2005Pokrovski, G.S., Roux, J., Harrichoury, J.C. (2005) Fluid density control on vapour-liquid partitioning of metals in hydrothermal systems. Geology 33, 657–660.
; Williams-Jones and Heinrich, 2005Williams-Jones, A., Heinrich, C. (2005) 100th Anniversary special paper: Vapour transport of metals and the formation of magmatic-hydrothermal ore deposits. Economic Geology 100, 1287–1312.
; Migdisov et al., 2013Migdisov, A.A., Bychkov, A.Y., Williams-Jones, A.E., van Hinsberg, V.J. (2013) A predictive model for the transport of copper by HCl-bearing water vapour in ore-forming magmatic-hydrothermal systems: Implications for copper porphyry ore formation. Geochimica et Cosmochimica Acta 129, 33-53.
). Vapour is particularly common in magmatic environments, and can be sampled directly at fumaroles and volcanic plumes. Compared to the source magma, vapours can be strongly enriched in metals (e.g., Allard et al., 2000Allard, P., Aiuppa, A., Loyer, H., Carrot, F., Gaudry, A., Pinte, G., Michel, A., Dongarrà, G. (2000) Acid gas and metal emission rates during long‐lived basalt degassing at Stromboli Volcano. Geophysical Research Letters 27, 1207–1210.
; Williams-Jones and Heinrich, 2005Williams-Jones, A., Heinrich, C. (2005) 100th Anniversary special paper: Vapour transport of metals and the formation of magmatic-hydrothermal ore deposits. Economic Geology 100, 1287–1312.
; van Hinsberg et al., 2016van Hinsberg, V., Vigouroux, N., Palmer, S., Berlo, K., Mauri, G., Williams-Jones, A., McKenzie, J., Williams-Jones, G., Fischer, T. (2016) Element flux to the environment of the passively degassing crater lake-hosting Kawah Ijen volcano, Indonesia, and implications for estimates of the global volcanic flux. In: Ohba, T., Capaccioni, B., Caudron, C. (Eds.) Geochemistry and Geophysics of Active Volcanic Lakes. Geological Society of London Special Publication, 437 pp.
), and metal mobility in shallow magmatic conduits is well-established (e.g., Berlo et al., 2013Berlo, K., Tuffen, H., Smith, V., Castro, J.M., Pyle, D.M., Mather, T.A., Geraki, K. (2013) Element variations in rhyolitic magma resulting from gas transport. Geochimica et Cosmochimica Acta 121, 436–451.
; Plail et al., 2014Plail, M., Edmonds, M, Humphreys, M.C.S., Barclay, J., Herd, R.A. (2014) Geochemical evidence for relict degassing pathways preserved in andesite. Earth and Planetary Science Letters 386, 21-33.
). Although evidence for high metal solubility in vapour inclusions in rocks is debated (Lerchbaumer and Audétat, 2012Lerchbaumer, L., Audétat, A. (2012) High Cu concentrations in vapour-type fluid inclusions: An artifact? Geochimica et Cosmochimica Acta 88, 255–274.
), experiments reproduce these high solubilities (Migdisov and Williams-Jones, 2013Migdisov, A.A., Williams-Jones, A.E. (2013) A predictive model for metal transport of silver chloride by aqueous vapour in ore-forming magmatic-hydrothermal systems. Geochimica et Cosmochimica Acta 104, 123–135.
; Migdisov et al., 2013Migdisov, A.A., Bychkov, A.Y., Williams-Jones, A.E., van Hinsberg, V.J. (2013) A predictive model for the transport of copper by HCl-bearing water vapour in ore-forming magmatic-hydrothermal systems: Implications for copper porphyry ore formation. Geochimica et Cosmochimica Acta 129, 33-53.
), thereby conclusively confirming that vapours are capable of transporting metals in high concentrations.The ligands available to elements in aqueous vapour and liquid are similar, although charged species are unimportant in vapour and, instead, uncharged molecules dominate element transport. However, the concentrations of metals in vapour inferred from fumarole emissions and fluid inclusions are commonly orders of magnitude higher than is predicted from metal volatility. The reason for this is the ability of water molecules to form hydrogen-bonded clusters that incorporate metal-bearing molecules, thereby significantly increasing stability, and hence solubility of the latter in the vapour phase. Recent experiments (Migdisov and Williams-Jones, 2013
Migdisov, A.A., Williams-Jones, A.E. (2013) A predictive model for metal transport of silver chloride by aqueous vapour in ore-forming magmatic-hydrothermal systems. Geochimica et Cosmochimica Acta 104, 123–135.
; Migdisov et al., 2013Migdisov, A.A., Bychkov, A.Y., Williams-Jones, A.E., van Hinsberg, V.J. (2013) A predictive model for the transport of copper by HCl-bearing water vapour in ore-forming magmatic-hydrothermal systems: Implications for copper porphyry ore formation. Geochimica et Cosmochimica Acta 129, 33-53.
; Hurtig and Williams-Jones, 2014Hurtig, N.C., Williams-Jones, A.E. (2014) An experimental study of the transport of gold through hydration of AuCl in aqueous vapour and vapour-like fluids. Geochimica et Cosmochimica Acta 127, 305–325.
) have demonstrated this effect for Cu, Ag and Au, and show that the complexity of these hydration clusters, and with them the stability of metal-bearing clusters, increases exponentially with increasing water vapour pressure. At the highest densities, the vapour can no longer be considered a low-density fluid as the previously isolated water clusters merge into the 3D hydrogen-bonded network typical of dense aqueous solutions.The density-, or pressure-dependence of metal solubility has been used to explain metal deposition during earthquake-induced depressurisation (Weatherley and Henley, 2013
Weatherley, D.K., Henley, R.W. (2013) Flash vapourization during earthquakes evidenced by gold deposits. Nature Geoscience 6, 294–298.
) and fluid ascent in volcanic-hydrothermal systems (Henley and Berger, 2013Henley, R.W., Berger, B.R. (2013) Nature’s refineries – metals and metalloids in arc volcanoes. Earth Science Reviews 125, 146-170.
). Depressurisation, however, is not the only mechanism that destabilises these clusters. Reducing the partial pressure of water, i.e. changing the vapour’s composition, produces the same effect. For example, dilution of water-rich vapour with a non-polar gas such as CO2, results in a drop in the partial pressure of water (pH2O), and hence destabilisation of hydrated metal-bearing clusters, comparable to that occurring during depressurisation. This effect is entirely independent of the total pressure or density of the system, and can be large even when the density (or Ptotal) of the mixed fluid rises during this dilution.In the following, we will show that fluxing of CO2-dominated vapour, released during degassing of deep-seated magma and known to transit magmatic-hydrothermal conduits, reduces pH2O significantly, leading to near-total collapse of the metal transporting capacity of the vapour. CO2-fluxing is thereby an efficient metal-deposition mechanism in these systems, although the required conditions are unlikely to be encountered in porphyry-Cu systems.
top
CO2-Fluxing in Magmatic Conduits
The solubility of volatiles in magma is controlled dominantly by pressure (Dixon et al., 1995
Dixon, J.E., Stolper, E.M., Holloway, J.R. (1995) An Experimental Study of Water and Carbon Dioxide Solubilities in Mid-Ocean Ridge Basaltic Liquids. Part I: Calibration and Solubility Models. Journal of Petrology 36, 1607–1631.
; Spilliaert et al., 2006aSpilliaert, N., Metrich, N., Allard, P. (2006a) S–Cl–F degassing pattern of water-rich alkali basalt: Modelling and relationship with eruption styles on Mount Etna volcano. Earth and Planetary Science Letters 248, 772–786.
) as are, therefore, the resulting exsolved fluid compositions. CO2 is the first major volatile to exsolve, and deep-released magmatic fluids are CO2-dominated, with H2O becoming more important as pressure decreases, followed by S, Cl, and F (Spilliaert et al., 2006aSpilliaert, N., Metrich, N., Allard, P. (2006a) S–Cl–F degassing pattern of water-rich alkali basalt: Modelling and relationship with eruption styles on Mount Etna volcano. Earth and Planetary Science Letters 248, 772–786.
).Magma ponds and exsolves volatiles at different levels in the crust, and can experience stages of closed- and open-system degassing. These volatiles are buoyant and flux through the overlying magmatic conduit, intermediate ponding levels, and the system’s host rocks. Fluxing of CO2-rich gas through shallower magma results in CO2 enrichment and melt dehydration (Spilliaert et al., 2006b
Spilliaert, N., Allard, P., Metrich, N. (2006b) Melt inclusion record of the conditions of ascent, degassing, and extrusion of volatile-rich alkali basalt during the powerful 2002 flank eruption of Mount Etna (Italy). Journal of Geophysical Research 111, B04203.
). High CO2, low H2O melt inclusions are now widely recognised, and generally attributed to CO2-fluxing (Spilliaert et al., 2006bSpilliaert, N., Allard, P., Metrich, N. (2006b) Melt inclusion record of the conditions of ascent, degassing, and extrusion of volatile-rich alkali basalt during the powerful 2002 flank eruption of Mount Etna (Italy). Journal of Geophysical Research 111, B04203.
; Blundy et al., 2010Blundy, J., Cashman, K.V., Rust, A., Witham, F. (2010) A case for CO2-rich arc magmas. Earth and Planetary Science Letters 290, 289–301.
). Gas fluxing has also been linked to enrichments in Cl, alkalis, Ti, Fe, and P (e.g., Ferlito et al., 2007Ferlito, C., Viccaro, M., Cristofolini, R. (2007) Volatile-induced magma differentiation in the plumbing system of Mt. Etna volcano (Italy): evidence from glass in tephra of the 2001 eruption. Bulletin of Volcanology 70, 455–473.
), Cu (Collins et al., 2009Collins, S.J., Pyle, D.M., Maclennan, J. (2009) Melt inclusions track pre-eruption storage and dehydration of magmas at Etna. Geology 37, 571–574.
), and Li (e.g., Berlo et al., 2004Berlo, K., Blundy, J., Turner, S., Cashman, K.V., Hawkesworth, C., Black, S. (2004) Geochemical Precursors to Volcanic Activity at Mount St. Helens, USA. Science 306, 1167–1169.
). These element enrichments are generally interpreted in terms of repressurisation, fluid boiling or crystallisation, but we argue here that changes in metal-complex stability resulting from CO2-fluxing are an attractive alternative.The pressure dependence of volatile-element solubility in magma leads to sharply different compositions of the exsolved fluid at different depths. A fluid in equilibrium with a typical MOR basalt at 1300 ˚C will contain 83 mole % CO2 and 4 % H2O at 1000 bar, but 17 mole % CO2 and 61 % H2O at the surface (Gaillard et al., 2012
Gaillard, F., Scaillet, B., Arndt, N.T. (2012) Atmospheric oxygenation caused by a change in volcanic degassing pressure. Nature 478, 229–232.
). To evaluate the impact of such drastic changes in composition, in particular in pH2O, on the ability of magmatic vapour to carry metals, and the potential of CO2-fluxing as a metal deposition mechanism, we modelled a vapour-filled fracture network in a magmatic-hydrothermal system subjected to a pulse of CO2-rich vapour (Fig. 1). Given that our purpose is to highlight the effects of CO2, we will explore endmember scenarios, rather than a specific geological case.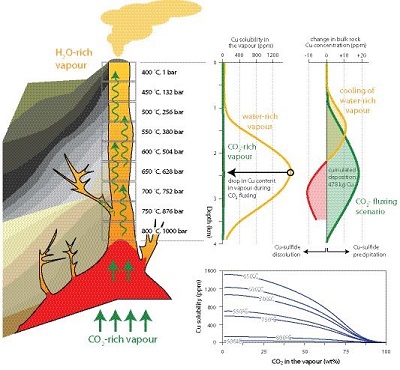
Figure 1 Impact of CO2-fluxing on Cu solubility in a volcanic conduit filled with vapour. Fluxing deep-sourced CO2-rich vapour through shallower, water-dominated vapour reduces Cu solubility as the water partial pressure is reduced. Cu contents are significant for the water-dominated vapour. Assuming upward flow, Cu is dissolved from wall rocks to 2.2 km, and subsequently deposited. In contrast, contents in the CO2-rich vapour are negligible. Therefore, a single pulse of CO2–rich vapour will deposit essentially all Cu in the water-vapour, with deposition at all depths and all temperatures.
top
Impact of CO2-Fluxing on Metal Solubility
In the simplest scenario, a CO2-rich vapour pulse, released from mafic magma at 1000 bar, passes through shallower, water-dominated vapour at 800 ˚C. To model this, we used gas compositions in equilibrium with mafic magma at various depths (Gaillard et al., 2012
Gaillard, F., Scaillet, B., Arndt, N.T. (2012) Atmospheric oxygenation caused by a change in volcanic degassing pressure. Nature 478, 229–232.
) at constant pHCl = 0.005 (Migdisov et al., 2013Migdisov, A.A., Bychkov, A.Y., Williams-Jones, A.E., van Hinsberg, V.J. (2013) A predictive model for the transport of copper by HCl-bearing water vapour in ore-forming magmatic-hydrothermal systems: Implications for copper porphyry ore formation. Geochimica et Cosmochimica Acta 129, 33-53.
), a Cu content buffered by chalcopyrite-pyrite coexistence (thermodynamic data sources as in Migdisov et al., 2013Migdisov, A.A., Bychkov, A.Y., Williams-Jones, A.E., van Hinsberg, V.J. (2013) A predictive model for the transport of copper by HCl-bearing water vapour in ore-forming magmatic-hydrothermal systems: Implications for copper porphyry ore formation. Geochimica et Cosmochimica Acta 129, 33-53.
) and fO2 at Ni-NiO. Speciation for the gas compositions taken from Gaillard et al. (2012)Gaillard, F., Scaillet, B., Arndt, N.T. (2012) Atmospheric oxygenation caused by a change in volcanic degassing pressure. Nature 478, 229–232.
was recalculated for our model conditions.Fluxing the CO2-rich gas through H2O-dominated vapour at 100 and 10 bar reduced Cu solubility by a factor of 50 and 100, respectively (Table 1). Decreasing the temperature to 650 ˚C, where Cu solubility is highest (Migdisov et al., 2013
Migdisov, A.A., Bychkov, A.Y., Williams-Jones, A.E., van Hinsberg, V.J. (2013) A predictive model for the transport of copper by HCl-bearing water vapour in ore-forming magmatic-hydrothermal systems: Implications for copper porphyry ore formation. Geochimica et Cosmochimica Acta 129, 33-53.
), and reducing the pHCl for the deeply-exsolved vapour tenfold to include the low volatility of Cl at high pressure (Spilliaert et al., 2006aSpilliaert, N., Metrich, N., Allard, P. (2006a) S–Cl–F degassing pattern of water-rich alkali basalt: Modelling and relationship with eruption styles on Mount Etna volcano. Earth and Planetary Science Letters 248, 772–786.
), had an even greater effect. Copper solubility at 100 bar dropped by four orders of magnitude (725 to 0.08 ppb). In essence, CO2 reduces the transporting capacity of the vapour to almost nothing, and Cu is deposited or reabsorbed by the melt. Per bar of pressure change, the drop in Cu solubility is 2.6 bar-1 for the 100 to 10 bar change in Ptotal at 650 ˚C, whereas it is 2.9 bar-1 and 82 bar-1 for ∆pH2O at 100 and 10 bar Ptotal, respectively (Table 1). When the accompanying reduction in pHCl is taken into account, CO2-fluxing is 100 to 600 times more effective in lowering Cu solubility per bar than decompression.Table 1 Predicted Cu solubility in vapours of varying pH2O and pHCl with fO2 and Cu buffered by NiO-Ni and CuFeS2-FeS2, respectively.
Pressure (bar) | Cu reduction factor | |||||||||
Vapour | T (˚C) | Ptotal | pH2O | pHCl | Cu (ppb) | pair | absolute | per bar | ||
1 | H2O-rich | 800 | 100 | 24.42 | 0.005 | 1.2 | ||||
2 | CO2-rich | 800 | 100 | 5.76 | 0.005 | 0.02 | 1 - 2 | 53 | 2.9 | |
3 | CO2-rich | 800 | 100 | 5.76 | 0.00005 | 0.0002 | 1 - 3 | 5232 | 280 | |
4 | H2O-rich | 800 | 10 | 1.41 | 0.005 | 0.004 | ||||
5 | CO2-rich | 800 | 10 | 0.21 | 0.005 | 0.00005 | 4 - 5 | 99 | 82 | |
6 | CO2-rich | 800 | 10 | 0.21 | 0.00005 | 0.0000005 | 4 - 6 | 9706 | 8104 | |
7 | H2O-rich | 650 | 100 | 23.54 | 0.005 | 724 | ||||
8 | CO2-rich | 650 | 100 | 5.72 | 0.005 | 8 | 7 - 8 | 91 | 5.1 | |
9 | CO2-rich | 650 | 100 | 5.72 | 0.00005 | 0.08 | 7 - 9 | 8957 | 503 | |
10 | H2O-rich | 650 | 10 | 0.81 | 0.005 | 3.1 | ||||
11 | CO2-rich | 650 | 10 | 0.1 | 0.005 | 0.02 | 10 - 11 | 148 | 208 | |
12 | CO2-rich | 650 | 10 | 0.1 | 0.00005 | 0.0002 | 10 - 12 | 14566 | 20515 | |
H2O-rich | 800 | 100 - 10 | 0.005 | 1 - 4 | 263 | 2.9 | ||||
| H2O-rich | 650 | 100 - 10 | | 0.005 | | | 7 - 10 | 237 | 2.6 |
Collins et al. (2009)
Collins, S.J., Pyle, D.M., Maclennan, J. (2009) Melt inclusions track pre-eruption storage and dehydration of magmas at Etna. Geology 37, 571–574.
report that melt inclusions from Etna volcano record an approximately twofold enrichment in Cu content, which was attributed to CO2-fluxing, with gas CO2 content increasing from 70 to 98 mole %. Unfortunately, the stability constants for the hydrated Cu-chloride species of Migdisov et al. (2013)Migdisov, A.A., Bychkov, A.Y., Williams-Jones, A.E., van Hinsberg, V.J. (2013) A predictive model for the transport of copper by HCl-bearing water vapour in ore-forming magmatic-hydrothermal systems: Implications for copper porphyry ore formation. Geochimica et Cosmochimica Acta 129, 33-53.
cannot be extrapolated to the 1140 ˚C reported for these inclusions, which precludes quantitative modelling of this system. To a first approximation, a 270 times decrease in Cu solubility is predicted from the change in pH2O, for a Ptotal of 1000 bar and fayalite-magnetite-quartz buffered fO2. The Etna melt is unlikely to be saturated in chalcopyrite, and this drop therefore represents a maximum concentration change. Nonetheless, it shows that CO2-fluxing introduces a sharp reduction in the Cu transporting capability of the gas that can indeed explain the observed correlation between fluxing and Cu contents in Etna melt inclusions.In our simple model, re-establishing a water-rich vapour composition following CO2-fluxing re-solvates the Cu, and the mechanism is fully reversible. However, kinetic barriers, collapse of a bubble foam as a result of CO2-fluxing, or choking of vapour pathways by fluxing-induced mineral deposition may preserve these deposits. Moreover, deposition of these elements on conduit walls will lead to their diffusion into the host rock or melt, as a local concentration gradient develops. Transient CO2-fluxing should thus be best preserved by the fastest diffusing elements, e.g., Li. Melt inclusions with elevated Li content and derived from a magma ponding horizon affected by CO2-fluxing have indeed been observed (e.g., Berlo et al., 2004
Berlo, K., Blundy, J., Turner, S., Cashman, K.V., Hawkesworth, C., Black, S. (2004) Geochemical Precursors to Volcanic Activity at Mount St. Helens, USA. Science 306, 1167–1169.
; Blundy et al., 2010Blundy, J., Cashman, K.V., Rust, A., Witham, F. (2010) A case for CO2-rich arc magmas. Earth and Planetary Science Letters 290, 289–301.
). However, the lack of stability constants for hydrated Li species in vapour prevents us from evaluating whether CO2-fluxing is capable of inducing a sufficient drop in Li solubility to generate the observed enrichments.top
Metal Enrichment by CO2-Fluxing
Magmatic-hydrothermal systems can be vertically extensive (e.g., Hedenquist and Lowenstern, 1994
Hedenquist, J.W., Lowenstern, J.B. (1994) The role of magmas in the formation of hydrothermal ore deposits. Nature 370, 519–527.
). To better represent these, we added a pressure-temperature gradient (Fig. 1). We assumed that the host rock had reacted to an equilibrium alteration assemblage prior to CO2-fluxing and therefore did not participate in the reactions. The initial, H2O-dominated vapour (XCO2 = 0.04) was Cu-saturated by equilibrating with chalcopyrite, and Cu solubility was tracked along the conduit length. Subsequently, the conduit was fluxed with CO2-rich vapour (XCO2 = 0.91) as in the simplified model above. In natural systems, Cu concentrations are unlikely to be at Cu-phase saturation throughout the system and throughout their lifespan (Landtwing et al., 2005Landtwing, M.R., Pettke, T., Halter, W.E., Heinrich, C.A., Redmond, P.B., Einaudi, M.T., Kunze, K. (2005) Copper deposition during quartz dissolution by cooling magmatic-hydrothermal fluids: The Bingham porphyry. Earth and Planetary Science Letters 235, 229–243.
), and these calculations thus provide maximum estimates. However, this is also true for other depositional mechanisms, and saturation therefore provides an appropriate benchmark for comparison.Copper solubility in the water-rich vapour reaches a maximum of 1575 ppm at 650 ˚C, resulting from the hydrated Cu-chloride species being most stable at this temperature (Migdisov et al., 2013
Migdisov, A.A., Bychkov, A.Y., Williams-Jones, A.E., van Hinsberg, V.J. (2013) A predictive model for the transport of copper by HCl-bearing water vapour in ore-forming magmatic-hydrothermal systems: Implications for copper porphyry ore formation. Geochimica et Cosmochimica Acta 129, 33-53.
; Fig. 1). In contrast, only 0.2 ppm Cu dissolves in the CO2-rich vapour at these conditions. Copper solubility decreases with increasing XCO2 for all temperatures (Fig. 1). Any vapour passing through the conduit will dissolve Cu at temperatures up to 650 ˚C, and only precipitate it after cooling below this temperature (Fig. 1). In contrast, CO2-fluxing will result in Cu deposition at any temperature, because of the drastic reduction in solubility. In addition, the total amount of Cu deposited in a fluxing scenario is higher than by simple cooling, because of the greater overall reduction in solubility.Given that water is a more abundant volatile than CO2, permeable gas networks such as bubble foams in magma and interconnected fracture networks will develop dominantly during release of water-rich fluid. However, once established, such networks allow volumetrically minor CO2-rich gases to flux through. CO2 is not consumed by fluxing-induced metal deposition, and a single pulse of CO2-rich gas can therefore lead to deposition of a significant mass of metal. Using the values calculated in the model above, a single pulse of CO2–rich vapour would deposit 478 kg of Cu along this 4 km long conduit for a 1 m2 conduit cross-section. Assuming a host-rock percolation threshold of 15 vol. %, this single pulse would enrich the bulk host rock in Cu by 8 ppm; at 650 ˚C the maximum pervasive enrichment would be 20 ppm. Depositing the Cu in veins, rather than pervasively, leads to locally higher concentrations, e.g., if these account for 5 % of rock volume, their Cu content peaks at 400 ppm. Although still small, this is for a single batch of fluid, and a single CO2-pulse, whereas many ore-forming systems are characterised by large fluid volumes in extensive hydrothermal circulation systems (e.g., Sillitoe, 2010
Sillitoe, R.H. (2010) Porphyry Copper Systems. Economic Geology 105, 3–41.
).Enrichment of a fracture network to wt. %-level Cu concentrations, i.e. economic levels, necessitates involvement of a water-rich vapour that transports Cu. Metal solubilities in CO2-rich vapour are negligible, preventing it from acting as a metal carrier, and its role is restricted to providing a highly efficient depositional mechanism. To explore a system where water- and CO2-rich vapours interact, we limited fluxing to 5 vol. % of the conduit network (Fig. 2a). After each CO2-pulse, H2O-rich vapour re-establishes itself in the fluxed part of the fracture network and mixes with the H2O-rich vapour in the 95 vol. % of the fracture network. Repeatedly fluxing this 5 vol. % section leads to a rapid increase in Cu content (Fig. 2b). Effectively, this redistributes Cu from the full system and concentrates it in the CO2-fluxed part. We included a re-dissolution fraction of 5 wt. % of total Cu in each calculation step, which can be interpreted as slow kinetics of re-dissolution, or short lifespans for individual fractures. Choosing a higher fraction necessitates more CO2-pulses to reach these Cu concentrations, but otherwise does not affect the model. Cu contents in the fluxed part of the fracture network readily reach wt. % concentrations (Fig. 2b).

Figure 2 Progressive Cu enrichment in a fracture network intermittently pulsed with CO2-rich vapour in its centre. (a) Schematic section showing Cu deposition on fracture walls followed by partial re-dissolution after each pulse, except for fractures sealed by precipitation. (b) Progressive enrichment of Cu assuming partial Cu re-dissolution, and fluxing of 5 vol. % of the fracture network. Cu is sourced from the 95 vol. % of the network filled with water-rich vapour.
top
Conclusions
We propose that CO2-fluxing is a highly efficient metal deposition mechanism that rivals established ore-depositing mechanisms. It can concentrate Cu to levels that are economic to mine in porphyry-Cu ore deposits. However, porphyry-Cu systems provide a benchmark only, and we do not advocate their formation by CO2-fluxing, because the combination of invoked geological conditions is unlikely to be present in these systems. Rather, CO2-fluxing has the potential to control metal contents in gas-fluxed magmas and on a local scale in associated hydrothermal systems. In the models presented here, metal deposition results from lowering water partial pressure and this can be brought about not only by fluxing with CO2, but also by fluxing with other gases (e.g., N2). It is moreover not restricted to vapours, but will also impact solubility for hydrated species in aqueous solutions (e.g., Akinfiev and Diamond, 2009
Akinfiev, N.N., Diamond, L.W. (2009) A simple predictive model of quartz solubility in water–salt–CO. Geochimica et Cosmochimica Acta 73, 1597–1608.
), albeit less drastically.top
Acknowledgements
The research leading to these results received funding from the NSERC Discovery Grant Program and the European Research Council under the European Union's Seventh Framework Program (FP/2007-2013) / ERC Grant Agreement number 307356.
Editor: Liane G. Benning
top
References
Akinfiev, N.N., Diamond, L.W. (2009) A simple predictive model of quartz solubility in water–salt–CO. Geochimica et Cosmochimica Acta 73, 1597–1608.

It is moreover not restricted to vapours, but will also impact solubility for hydrated species in aqueous solutions (e.g., Akinfiev and Diamond, 2009), albeit less drastically.
View in article
Allard, P., Aiuppa, A., Loyer, H., Carrot, F., Gaudry, A., Pinte, G., Michel, A., Dongarrà, G. (2000) Acid gas and metal emission rates during long‐lived basalt degassing at Stromboli Volcano. Geophysical Research Letters 27, 1207–1210.

Compared to the source magma, vapours can be strongly enriched in metals (e.g., Allard et al., 2000; Williams-Jones and Heinrich, 2005; van Hinsberg et al., 2016), and metal mobility in shallow magmatic conduits is well-established (e.g., Berlo et al., 2013; Plail et al., 2014).
View in article
Berlo, K., Blundy, J., Turner, S., Cashman, K.V., Hawkesworth, C., Black, S. (2004) Geochemical Precursors to Volcanic Activity at Mount St. Helens, USA. Science 306, 1167–1169.

Gas fluxing has also been linked to enrichments in Cl, alkalis, Ti, Fe, and P (e.g., Ferlito et al., 2007), Cu (Collins et al., 2009), and Li (e.g., Berlo et al., 2004).
View in article
Transient CO2-fluxing should thus be best preserved by the fastest diffusing elements, e.g., Li. Melt inclusions with elevated Li content and derived from a magma ponding horizon affected by CO2-fluxing have indeed been observed (e.g., Berlo et al., 2004; Blundy et al., 2010).
View in article
Berlo, K., Tuffen, H., Smith, V., Castro, J.M., Pyle, D.M., Mather, T.A., Geraki, K. (2013) Element variations in rhyolitic magma resulting from gas transport. Geochimica et Cosmochimica Acta 121, 436–451.

Compared to the source magma, vapours can be strongly enriched in metals (e.g., Allard et al., 2000; Williams-Jones and Heinrich, 2005; van Hinsberg et al., 2016), and metal mobility in shallow magmatic conduits is well-established (e.g., Berlo et al., 2013; Plail et al., 2014).
View in article
Blundy, J., Cashman, K.V., Rust, A., Witham, F. (2010) A case for CO2-rich arc magmas. Earth and Planetary Science Letters 290, 289–301.

High CO2, low H2O melt inclusions are now widely recognised, and generally attributed to CO2-fluxing (Spilliaert et al., 2006b; Blundy et al., 2010).
View in article
Transient CO2-fluxing should thus be best preserved by the fastest diffusing elements, e.g., Li. Melt inclusions with elevated Li content and derived from a magma ponding horizon affected by CO2-fluxing have indeed been observed (e.g., Berlo et al., 2004; Blundy et al., 2010).
View in article
Collins, S.J., Pyle, D.M., Maclennan, J. (2009) Melt inclusions track pre-eruption storage and dehydration of magmas at Etna. Geology 37, 571–574.

Gas fluxing has also been linked to enrichments in Cl, alkalis, Ti, Fe, and P (e.g., Ferlito et al., 2007), Cu (Collins et al., 2009), and Li (e.g., Berlo et al., 2004).
View in article
Collins et al. (2009) report that melt inclusions from Etna volcano record an approximately twofold enrichment in Cu content, which was attributed to CO2-fluxing, with gas CO2 content increasing from 70 to 98 mole %.
View in article
Dixon, J.E., Stolper, E.M., Holloway, J.R. (1995) An Experimental Study of Water and Carbon Dioxide Solubilities in Mid-Ocean Ridge Basaltic Liquids. Part I: Calibration and Solubility Models. Journal of Petrology 36, 1607–1631.

The solubility of volatiles in magma is controlled dominantly by pressure (Dixon et al., 1995; Spilliaert et al., 2006a) as are, therefore, the resulting exsolved fluid compositions.
View in article
Ferlito, C., Viccaro, M., Cristofolini, R. (2007) Volatile-induced magma differentiation in the plumbing system of Mt. Etna volcano (Italy): evidence from glass in tephra of the 2001 eruption. Bulletin of Volcanology 70, 455–473.

Gas fluxing has also been linked to enrichments in Cl, alkalis, Ti, Fe, and P (e.g., Ferlito et al., 2007), Cu (Collins et al., 2009), and Li (e.g., Berlo et al., 2004).
View in article
Gaillard, F., Scaillet, B., Arndt, N.T. (2012) Atmospheric oxygenation caused by a change in volcanic degassing pressure. Nature 478, 229–232.

A fluid in equilibrium with a typical MOR basalt at 1300 ˚C will contain 83 mole % CO2 and 4 % H2O at 1000 bar, but 17 % CO2 and 61 % H2O at the surface (Gaillard et al., 2012).
View in article
To model this, we used gas compositions in equilibrium with mafic magma at various depths (Gaillard et al., 2012) at constant pHCl = 0.005 (Migdisov et al., 2013), a Cu content buffered by chalcopyrite-pyrite coexistence (thermodynamic data sources as in Migdisov et al., 2013) and ƒO2 at Ni-NiO.
View in article
Speciation for the gas compositions taken from Gaillard et al. (2012) was recalculated for our model conditions.
View in article
Hedenquist, J.W., Lowenstern, J.B. (1994) The role of magmas in the formation of hydrothermal ore deposits. Nature 370, 519–527.

Aqueous vapour is increasingly recognised as an important agent of element mobility in the crust (Hedenquist and Lowenstern, 1994; Pokrovski et al., 2005; Williams-Jones and Heinrich, 2005; Migdisov et al., 2013).
View in article
Magmatic-hydrothermal systems can be vertically extensive (e.g., Hedenquist and Lowenstern, 1994).
View in article
Henley, R.W., Berger, B.R. (2013) Nature’s refineries – metals and metalloids in arc volcanoes. Earth Science Reviews 125, 146-170.

The density-, or pressure-dependence of metal solubility has been used to explain metal deposition during earthquake-induced depressurisation (Weatherley and Henley, 2013) and fluid ascent in volcanic-hydrothermal systems (Henley and Berger, 2013).
View in article
Hurtig, N.C., Williams-Jones, A.E. (2014) An experimental study of the transport of gold through hydration of AuCl in aqueous vapour and vapour-like fluids. Geochimica et Cosmochimica Acta 127, 305–325.

Recent experiments (Migdisov and Williams-Jones, 2013; Migdisov et al., 2013; Hurtig and Williams-Jones, 2014) have demonstrated this effect for Cu, Ag and Au, and show that the complexity of these hydration clusters, and with them the stability of metal-bearing clusters, increases exponentially with increasing water vapour pressure.
View in article
Landtwing, M.R., Pettke, T., Halter, W.E., Heinrich, C.A., Redmond, P.B., Einaudi, M.T., Kunze, K. (2005) Copper deposition during quartz dissolution by cooling magmatic-hydrothermal fluids: The Bingham porphyry. Earth and Planetary Science Letters 235, 229–243.

Subsequently, the conduit was fluxed with CO2-rich vapour (XCO2 = 0.91) as in the simplified model above. In natural systems, Cu concentrations are unlikely to be at Cu-phase saturation throughout the system and throughout their lifespan (Landtwing et al., 2005), and these calculations thus provide maximum estimates.
View in article
Lerchbaumer, L., Audétat, A. (2012) High Cu concentrations in vapour-type fluid inclusions: An artifact? Geochimica et Cosmochimica Acta 88, 255–274.

Although evidence for high metal solubility in vapour inclusions in rocks is debated (Lerchbaumer and Audétat, 2012), experiments reproduce these high solubilities (Migdisov and Williams-Jones, 2013; Migdisov et al., 2013), thereby conclusively confirming that vapours are capable of transporting metals in high concentrations.
View in article
Migdisov, A.A., Williams-Jones, A.E. (2013) A predictive model for metal transport of silver chloride by aqueous vapour in ore-forming magmatic-hydrothermal systems. Geochimica et Cosmochimica Acta 104, 123–135.

Although evidence for high metal solubility in vapour inclusions in rocks is debated (Lerchbaumer and Audétat, 2012), experiments reproduce these high solubilities (Migdisov and Williams-Jones, 2013; Migdisov et al., 2013), thereby conclusively confirming that vapours are capable of transporting metals in high concentrations.
View in article
Recent experiments (Migdisov and Williams-Jones, 2013; Migdisov et al., 2013; Hurtig and Williams-Jones, 2014) have demonstrated this effect for Cu, Ag and Au, and show that the complexity of these hydration clusters, and with them the stability of metal-bearing clusters, increases exponentially with increasing water vapour pressure.
View in article
Migdisov, A.A., Bychkov, A.Y., Williams-Jones, A.E., van Hinsberg, V.J. (2013) A predictive model for the transport of copper by HCl-bearing water vapour in ore-forming magmatic-hydrothermal systems: Implications for copper porphyry ore formation. Geochimica et Cosmochimica Acta 129, 33-53.

Aqueous vapour is increasingly recognised as an important agent of element mobility in the crust (Hedenquist and Lowenstern, 1994; Pokrovski et al., 2005; Williams-Jones and Heinrich, 2005; Migdisov et al., 2013).
View in article
Although evidence for high metal solubility in vapour inclusions in rocks is debated (Lerchbaumer and Audétat, 2012), experiments reproduce these high solubilities (Migdisov and Williams-Jones, 2013; Migdisov et al., 2013), thereby conclusively confirming that vapours are capable of transporting metals in high concentrations.
View in article
Recent experiments (Migdisov and Williams-Jones, 2013; Migdisov et al., 2013; Hurtig and Williams-Jones, 2014) have demonstrated this effect for Cu, Ag and Au, and show that the complexity of these hydration clusters, and with them the stability of metal-bearing clusters, increases exponentially with increasing water vapour pressure.
View in article
To model this, we used gas compositions in equilibrium with mafic magma at various depths (Gaillard et al., 2012) at constant pHCl = 0.005 (Migdisov et al., 2013), a Cu content buffered by chalcopyrite-pyrite coexistence (thermodynamic data sources as in Migdisov et al., 2013) and ƒO2 at Ni-NiO.
View in article
Decreasing the temperature to 650 ˚C, where Cu solubility is highest (Migdisov et al., 2013), and reducing the pHCl for the deeply-exsolved vapour tenfold to include the low volatility of Cl at high pressure (Spilliaert et al., 2006a), had an even greater effect.
View in article
Unfortunately, the stability constants for the hydrated Cu-chloride species of Migdisov et al. (2013) cannot be extrapolated to the 1140 ˚C reported for these inclusions, which precludes quantitative modelling of this system.
View in article
Copper solubility in the water-rich vapour reaches a maximum of 1575 ppm at 650 ˚C, resulting from the hydrated Cu-chloride species being most stable at this temperature (Migdisov et al., 2013; Fig. 1).
View in article
Plail, M., Edmonds, M, Humphreys, M.C.S., Barclay, J., Herd, R.A. (2014) Geochemical evidence for relict degassing pathways preserved in andesite. Earth and Planetary Science Letters 386, 21-33.

Compared to the source magma, vapours can be strongly enriched in metals (e.g., Allard et al., 2000; Williams-Jones and Heinrich, 2005; van Hinsberg et al., 2016), and metal mobility in shallow magmatic conduits is well-established (e.g., Berlo et al., 2013; Plail et al., 2014).
View in article
Pokrovski, G.S., Roux, J., Harrichoury, J.C. (2005) Fluid density control on vapour-liquid partitioning of metals in hydrothermal systems. Geology 33, 657–660.

Aqueous vapour is increasingly recognised as an important agent of element mobility in the crust (Hedenquist and Lowenstern, 1994; Pokrovski et al., 2005; Williams-Jones and Heinrich, 2005; Migdisov et al., 2013).
View in article
Sillitoe, R.H. (2010) Porphyry Copper Systems. Economic Geology 105, 3–41.

Although still small, this is for a single batch of fluid, and a single CO2-pulse, whereas many ore-forming systems are characterised by large fluid volumes in extensive hydrothermal circulation systems (e.g., Sillitoe, 2010).
View in article
Spilliaert, N., Metrich, N., Allard, P. (2006a) S–Cl–F degassing pattern of water-rich alkali basalt: Modelling and relationship with eruption styles on Mount Etna volcano. Earth and Planetary Science Letters 248, 772–786.

The solubility of volatiles in magma is controlled dominantly by pressure (Dixon et al., 1995; Spilliaert et al., 2006a) as are, therefore, the resulting exsolved fluid compositions.
View in article
CO2 is the first major volatile to exsolve, and deep-released magmatic fluids are CO2-dominated, with H2O becoming more important as pressure decreases, followed by S, Cl, and F (Spilliaert et al., 2006a).
View in article
Decreasing the temperature to 650 ˚C, where Cu solubility is highest (Migdisov et al., 2013), and reducing the pHCl for the deeply-exsolved vapour tenfold to include the low volatility of Cl at high pressure (Spilliaert et al., 2006a), had an even greater effect.
View in article
Spilliaert, N., Allard, P., Metrich, N. (2006b) Melt inclusion record of the conditions of ascent, degassing, and extrusion of volatile-rich alkali basalt during the powerful 2002 flank eruption of Mount Etna (Italy). Journal of Geophysical Research 111, B04203.

Fluxing of CO2-rich gas through shallower magma results in CO2 enrichment and melt dehydration (Spilliaert et al., 2006b).
View in article
High CO2, low H2O melt inclusions are now widely recognised, and generally attributed to CO2-fluxing (Spilliaert et al., 2006b; Blundy et al., 2010).
View in article
van Hinsberg, V., Vigouroux, N., Palmer, S., Berlo, K., Mauri, G., Williams-Jones, A., McKenzie, J., Williams-Jones, G., Fischer, T. (2016) Element flux to the environment of the passively degassing crater lake-hosting Kawah Ijen volcano, Indonesia, and implications for estimates of the global volcanic flux. In: Ohba, T., Capaccioni, B., Caudron, C. (Eds.) Geochemistry and Geophysics of Active Volcanic Lakes. Geological Society of London Special Publication, 437 pp.

Compared to the source magma, vapours can be strongly enriched in metals (e.g., Allard et al., 2000; Williams-Jones and Heinrich, 2005; van Hinsberg et al., 2016), and metal mobility in shallow magmatic conduits is well-established (e.g., Berlo et al., 2013; Plail et al., 2014).
View in article
Weatherley, D.K., Henley, R.W. (2013) Flash vapourization during earthquakes evidenced by gold deposits. Nature Geoscience 6, 294–298.

The density-, or pressure-dependence of metal solubility has been used to explain metal deposition during earthquake-induced depressurisation (Weatherley and Henley, 2013) and fluid ascent in volcanic-hydrothermal systems (Henley and Berger, 2013).
View in article
Williams-Jones, A., Heinrich, C. (2005) 100th Anniversary special paper: Vapour transport of metals and the formation of magmatic-hydrothermal ore deposits. Economic Geology 100, 1287–1312.

Aqueous vapour is increasingly recognised as an important agent of element mobility in the crust (Hedenquist and Lowenstern, 1994; Pokrovski et al., 2005; Williams-Jones and Heinrich, 2005; Migdisov et al., 2013).
View in article
Compared to the source magma, vapours can be strongly enriched in metals (e.g., Allard et al., 2000; Williams-Jones and Heinrich, 2005; van Hinsberg et al., 2016), and metal mobility in shallow magmatic conduits is well-established (e.g., Berlo et al., 2013; Plail et al., 2014).
View in article
top
Supplementary Information
Details on Calculations and Methodology
Thermodynamic calculations
The solubility of Cu in the CO2-H2O(-HCl) vapours was calculated from thermodynamic stability constants for the relevant vapour species and the H2O-CO2 medium at the temperatures and pressures of the respective simulations. To set model boundary conditions for the oxygen fugacity and Cu activity, each vapour was equilibrated with a mineral assemblage consisting of chalcopyrite, pyrite, Ni and NiO. Chalcopyrite is the most common Cu-mineral in nature, and likely controls the solubility of copper in a wide variety of environments. Its presence, together with that of pyrite, saturates the vapour in Cu at a level representative for many Cu mineralisations. Ni and NiO buffer the oxygen fugacities of the system, but do not partake in the speciation reactions as no other Ni-species bar Ni(s) and NiO(s) were allowed to form. Calculations for Etna volcano were performed with oxygen fugacity fixed by the assemblage fayalite-magnetite-quartz.
Copper saturation of an HCl-bearing vapour with Cu-bearing species is controlled by the following reactions (Migdisov et al., 2013
Migdisov, A.A., Bychkov, A.Y., Williams-Jones, A.E., van Hinsberg, V.J. (2013) A predictive model for the transport of copper by HCl-bearing water vapour in ore-forming magmatic-hydrothermal systems: Implications for copper porphyry ore formation. Geochimica et Cosmochimica Acta 129, 33-53, doi: 10.1016/j.gca.2013.12.024.
):Eq. S-1
CuFeS2chalcopyrite + HClgas + 0.25O2gas + (n-0.5) H2Ogas = CuCl:(H2O)ngas + FeS2pyrite
Eq. S-2
CuFeS2chalcopyrite + 2HClgas + 0.25O2gas + 2H2Ogas = CuCl:HCl:(H2O)2.5gas + FeS2pyrite
The Cu solubility calculations thus involved the following species: CuClgas, Cu3Cl3gas, CuCl:(H2O)ngas, CuCl:HCl:(H2O)2.5gas, HClgas, O2gas, H2Ogas, and CO2gas. Considering that data on the stability of the hydrated gaseous Fe-bearing chlorides as well as the hydrated Cu-(H)xSy species are not available, these species were not accounted for in the modelling. Reduced sulphur species are known to solvate Cu in aqueous vapour (e.g., Simon et al., 2006
Simon, A.C., Pettke, T., Candela, P.A., Piccoli, P.M., Heinrich, C.A. (2006) Copper partitioning in a melt-vapor-brine-magnetite-pyrrhotite assemblage. Geochimica et Cocmochimica Acta 70, 5583-5600.
; Zajacz et al., 2011Zajacz, Z., Seo, J.H., Candela, P., Piccoli, P.M., Tossell, J.A. (2011) The solubility of copper in high-temperature magmatic vapors: A quest for significance of various chloride and sulfide complexes. Geochimica et Cocmochimica Acta 75, 2811-2827.
), and excluding these from the present calculations will thus underestimate the solubility of Cu. Fugacity and partial pressures of pure water were calculated using the equation of state reported in Kestin et al. (1984)Kestin, J., Sengers, J.V., Kamgar-Parsi, B., Sengers, J.M.H.L. (1984) Thermophysical Properties of Fluid H2O. Journal of Physical and Chemical Reference Data 13, 175, doi: 10.1063/1.555707.
; gaseous mixtures as well as the properties of pure HClgas, O2gas, and CO2gas were modelled using the PRSV equation of state (Stryjek and Vera, 1986Stryjek, R., Vera, J.H. (1986) PRSV: An improved peng-Robinson equation of state for pure compounds and mixtures. The Canadian Journal of Chemical Engineering 64, 323–333, doi: 10.1002/cjce.5450640224.
); hydrated copper chlorides were assumed to obey the Ideal Gas Law. The calculations were performed using the HCh software package for geochemical equilibrium modelling (Shvarov and Bastrakov, 1999Shvarov, Y., Bastrakov, E. (1999) HCh: a software package for geochemical equilibrium modeling - User's guide. Record 1999/025, Australian Geological Survey Organisation, Canberra.
). Thermodynamic properties required for these calculations were taken from Migdisov et al. (2013)Migdisov, A.A., Bychkov, A.Y., Williams-Jones, A.E., van Hinsberg, V.J. (2013) A predictive model for the transport of copper by HCl-bearing water vapour in ore-forming magmatic-hydrothermal systems: Implications for copper porphyry ore formation. Geochimica et Cosmochimica Acta 129, 33-53, doi: 10.1016/j.gca.2013.12.024.
for the Cu/Cl species, and from Robie and Hemingway (1995)Robie, R.A., Hemingway, B.S. (1995) Thermodynamic Properties of Minerals and Related Substances at 298.15 K and 1 Bar (105 Pascals) Pressure and at Higher Temperatures. US Geological Survey Bulletin 2131, 1–470.
and Holland and Powell (1998)Holland, T.J.B., Powell, R. (1998) An internally consistent thermodynamic data set for phases of petrological interest. Journal of Metamorphic Geology 16, 309–343.
for chalcopyrite, pyrite, Ni, NiO, magnetite, fayalite, quartz, HClgas, O2gas, and CO2gas.Single CO2-pulse model
In the simplest scenario, we model a single pulse of CO2-rich vapour passing through a, for simplicity, static water-dominated vapour at 800 ˚C (in principal only relative movement of the CO2 vapour is required and both could be moving up the conduit in absolute terms). The CO2 vapour pulse is assumed to diffuse into the water-rich vapour and change the vapour’s composition to that of the CO2-dominated vapour, i.e. changing the medium without a change in total pressure. In natural systems, introduction of a CO2-rich vapour may lead to a rise in Ptotal, which will reduce the impact of the decrease in pH2O. However, given that the effect of a change in pH2O exceeds that resulting from a change in Ptotal per bar of change (Table 1), it is unlikely able to compensate. Cu solubility was calculated at 10 and 100 bar in both water- and a CO2-dominated vapour. The compositions of the water-dominated vapour at 10 and 100 bar are those in equilibrium with mafic magma at these pressures, as taken from Gaillard et al. (2012)
Gaillard, F., Scaillet, B., Arndt, N.T. (2012) Atmospheric oxygenation caused by a change in volcanic degassing pressure. Nature 478, 229–232, doi: 10.1038/nature10460.
. The CO2-dominated vapour pulse is the composition of the vapour in equilibrium with this same mafic magma at 1000 bar from Gaillard et al. (2012)Gaillard, F., Scaillet, B., Arndt, N.T. (2012) Atmospheric oxygenation caused by a change in volcanic degassing pressure. Nature 478, 229–232, doi: 10.1038/nature10460.
, with its speciation and species fugacities recalculated for 10 or 100 bar using the thermodynamic data and models detailed above. In the first set of calculations, pHCl was kept constant at 0.005 to isolate the effect of CO2 on solubility. However, the deep-degassed CO2-rich vapour pulse will have a lower HCl content, because Cl-solubility in the melt is strongly pressure dependent (Spilliaert et al., 2006Spilliaert, N., Metrich, N., Allard, P. (2006) S–Cl–F degassing pattern of water-rich alkali basalt: Modelling and relationship with eruption styles on Mount Etna volcano. Earth And Planetary Science Letters 248, 772–786.
). To show the combined impact of lowering pH2O and pHCl, we reduced the pHCl by an arbitrary factor of 10. The model of Witham et al. (2012)Witham, F., Blundy, J., Kohn, S.C., Lesne, P., Dixon, J., Churakov, S.V., Botcharnikov, R. (2012) SolEx: A model for mixed COHSCl-volatile solubilities and exsolved gas compositions in basalt. Computers & Geosciences 45, 87–97, doi: 10.1016/j.cageo.2011.09.021.
predicts a more than tenfold decrease for a typical basalt when comparing the exsolved gas at 1000 bar to that at 10 bar, showing that this is a reasonable reduction. Lowering the pHCl further reduces the Cu solubility (Tables 1 and S-1).Table S-1 Gas compositions used in the calculations and calculated Cu solubility.
Conditions | CO2-rich vapour pulse | Receiving vapour | |||||
T (˚C) | 800 | 800 | 800 | 800 | 800 | ||
P (bar) | 1000 | 100* | 10* | 100 | 10 | ||
Input gas composition in bar | |||||||
pCO2 | 1116 | 90 | 8.7 | 62 | 2.9 | ||
pCO | 83 | 6.1 | 0.66 | 0.4 | 0.02 | ||
pH2O | 8.7 | 1.41 | 0.21 | 24 | 5.8 | ||
pH2S | 48 | 3.93 | 0.31 | 12 | 0.99 | ||
pH2 | 0.71 | 0.1 | 0.02 | 0.17 | 0.04 | ||
pS2 | 1 | 0.33 | 0.07 | 1.1 | 0.13 | ||
pSO2 | 0.004 | 0.003 | 0.001 | 0.59 | 0.18 | ||
Calculated Cu solubility in ppb | |||||||
Cu | | - | 0.022 | 4.6 10-5 | | 1.18 | 4.5 10-3 |
* These CO2-rich vapours represent the 1000 bar vapour with partial pressures recal-culated to equilibrium values at 100 and 10 bar using the thermodynamic data listed above.
Download in ExcelOur calculations do not consider all Cu-species that have been proposed to control Cu solubility in vapour for lack of the thermodynamic data to model these. Hydrated Cu-(H)xSy species, in particular, are regarded as important carriers of Cu in vapour (e.g., Simon et al., 2006
Simon, A.C., Pettke, T., Candela, P.A., Piccoli, P.M., Heinrich, C.A. (2006) Copper partitioning in a melt-vapor-brine-magnetite-pyrrhotite assemblage. Geochimica et Cocmochimica Acta 70, 5583-5600.
; Zajacz et al., 2011Zajacz, Z., Seo, J.H., Candela, P., Piccoli, P.M., Tossell, J.A. (2011) The solubility of copper in high-temperature magmatic vapors: A quest for significance of various chloride and sulfide complexes. Geochimica et Cocmochimica Acta 75, 2811-2827.
). The impact of CO2-fluxing on the stability of such species cannot be assessed quantitatively, but qualitatively. Addition of CO2 will result in dilution of the constituent elements of these species, thereby reducing their partial pressures, e.g., pH2S, which will lower the stability of these species and, hence, the solubility of Cu. Including additional Cu-species thus changes the absolute value of the Cu solubility, but these species are similarly affected by CO2-fluxing, and a strong reduction in Cu content in the vapour will hence still ensue.Conduit model
To evaluate the impact of changes in metal solubility resulting from CO2 fluxing in a geological environment, we modelled a vapour filled magmatic-hydrothermal conduit or fracture network (Fig. 1). Temperature decreased linearly in this conduit from 800 to 400 ˚C and the system was subjected to a lithostatic pressure gradient using a wall-rock density of 2700 kgm-3 (i.e. dacite) from 1000 to 1 bar. Solubilities were calculated for a typical, water-dominated arc-volcanic gas emitted from an andesitic-dacitic volcanic system (XH2O = 0.953; XCO2 = 0.035; XCO = 2∙10-8; XH2S = 6.9∙10-3; XSO2 = 4.9∙10-3; XH2 = 2∙10-7; XHCl = 1.13∙10-3), progressively fluxed by the CO2-rich vapour listed in Table S-1. Major and trace element vapour speciation was recalculated for each set of P-T conditions using the thermodynamic data and models listed above. This system mimics a dacite-hosted magmatic-hydrothermal system fluxed by CO2-rich vapours released from a deeper mafic magma. The system is assumed to be in equilibrium and no exchange between host rock and vapour takes place except for that involving Cu solubility (see above), i.e. the host rock has been altered to a mature secondary silicate mineral paragenesis in equilibrium with the vapour and therefore acts as a passive boundary. There is no vertical movement of the water-dominated vapour through the conduit.
To calculate the amount of Cu deposited by fluxing, we integrated the area under the solubility curves for the most water-rich and most CO2-rich vapours to arrive at the total mass of Cu in the conduit for each vapour, the difference of which represents the mass deposited. An interconnected fracture network reaches permeability at ~15 vol. % and we took this number as the fraction of the conduit or fracture network occupied by vapour (fluxing requires a permeable network). The mass of depositing Cu was hence distributed over 85 vol. % of dacite at a density of 2700 kgm-3 to determine the increase in Cu content in the host dacite for a single pulse of CO2-rich vapour assuming pervasive deposition of Cu and a cylindrical conduit. Deposition of Cu per unit conduit length was similarly calculated by splitting the conduit into 9 sections at 50 ˚C spacing (Fig. 1) and integrating the solubility curve area for each section.
Pulsed conduit model
Vapours rich in CO2 have negligible transporting capacity for Cu. This means that fluxing by such vapours does not add metal to the system and only represents a highly efficient depositional mechanism. To form a mineralisation thus requires involvement of a water-dominated vapour to act as the metal-carrying medium. In our final model, we represent such a system by assuming that only 5 % of the conduit or fracture network is fluxed by CO2-rich vapour for any given pulse, and pulses are repeated. Multiple pulses of vapour with varying X(CO2) are known from a number of volcanoes. Etna volcano, in particular, has displayed rapid and persistent fluctuations in X(CO2) on timescales from minutes to weeks (Allard et al., 2005
Allard, P., Burton, M., Murè, F. (2005) Spectroscopic evidence for a lava fountain driven by previously accumulated magmatic gases. Nature 433, 407-410, doi: 10.1038/nature03246.
; Aiuppa et al., 2007Aiuppa, A., Moretti, R., Federico, C., Giudice, G., Gurrieri, S., Liuzzo, M., Papale, P., Shinohara, H., Valenza, M. (2007) Forecasting Etna eruptions by real-time observation of volcanic gas composition. Geology 35, 1115–1118.
). The compositions of the water-rich and CO2-rich vapours and, hence, their Cu solubility, are as in the preceding model. As above, the fracture network has a 15 vol. % permeability and is hosted by dacite with a 2700 kgm-3 density.Each model step consists of:
1. A pulse of CO2-rich vapour passing through 5 vol. % of the fracture network filled with water-dominated vapour saturated in chalcopyrite thereby leading to Cu deposition. This step corresponds to the conduit model described in the preceding section.
2. Subsequently, the vapour in this 5 vol. % section is mixed with the water-rich vapour remaining in the rest of the fracture network, and 5 wt. % of the chalcopyrite deposited is allowed to re-dissolve into this vapour as long as this does not exceed Cu solubility (alternatively, Cu re-dissolves up to this solubility limit). This step represents the re-establishment of the water vapour, after the CO2-pulse has passed, by an influx of water-rich vapour from the rest of the fracture network. The 5 wt. % re-dissolution limit is arbitrary and was set to simulate kinetic hindrance of chalcopyrite re-dissolution and sealing off of fractures by mineral precipitation during the deposition event. Such sealed sections will no longer participate and are a well-established feature of many mineralisations, including porphyry Cu deposits as evidenced by multi-stage and crosscutting vein relationships (Sillitoe, 2010
Sillitoe, R.H. (2010) Porphyry Copper Systems. Economic Geology 105, 3–41.
).Pulses were subsequently repeated, leading to a progressive enrichment of Cu in the fluxed part of the fracture network. This Cu is sourced from the 95 vol. % of the fracture network not being fluxed, and this model thus represents a redistribution of Cu with the water-rich vapour acting as the transporting agent, and local enrichment as a result of Cu deposition by CO2-fluxing. Enrichment slows with pulse number because the relative contribution from the water vapour mixing back in decreases over time compared to the residual Cu resulting from incomplete re-dissolution. Increasing the re-dissolution fraction slows the build-up of Cu, but it does reach the same concentrations (except when the re-dissolution fraction = 1, because in this case all Cu is re-dissolved and thus reaches its solubility limit and no Cu is hence redistributed into the fluxed part of the fracture network).
Deposition of metals in many ore-deposits, for example Cu-porphyry systems, is not pervasive, but concentrated in discrete veins (Sillitoe, 2010
Sillitoe, R.H. (2010) Porphyry Copper Systems. Economic Geology 105, 3–41.
). To simulate this, a “volume fraction of receiving wall rock” was introduced into the model. A value of 1 represents complete pervasive deposition, whereas lower values represent progressive concentration of Cu on fracture walls (Fig. 2a), i.e. forming chalcopyrite veins. From integration of the solubility curve area following the approach described above for the conduit model we calculate the concentrations of Cu in these fracture walls for a number of volume fractions (Fig. 2b) and show that these concentrations correspond to levels found in economically viable Cu deposits for volume fractions of less than ~5 %. This would represent a mineralised system where chalcopyrite veins make up less than 5 vol. % of the total hydrothermal fracture network.Supplementary Information References
Aiuppa, A., Moretti, R., Federico, C., Giudice, G., Gurrieri, S., Liuzzo, M., Papale, P., Shinohara, H., Valenza, M. (2007) Forecasting Etna eruptions by real-time observation of volcanic gas composition. Geology 35, 1115–1118.

Etna volcano, in particular, has displayed rapid and persistent fluctuations in X(CO2) on timescales from minutes to weeks (Allard et al., 2005; Aiuppa et al., 2007).
View in Supplementary Information
Allard, P., Burton, M., Murè, F. (2005) Spectroscopic evidence for a lava fountain driven by previously accumulated magmatic gases. Nature 433, 407-410, doi: 10.1038/nature03246.

Etna volcano, in particular, has displayed rapid and persistent fluctuations in X(CO2) on timescales from minutes to weeks (Allard et al., 2005; Aiuppa et al., 2007).
View in Supplementary Information
Gaillard, F., Scaillet, B., Arndt, N.T. (2012) Atmospheric oxygenation caused by a change in volcanic degassing pressure. Nature 478, 229–232, doi: 10.1038/nature10460.

The compositions of the water-dominated vapour at 10 and 100 bar are those in equilibrium with mafic magma at these pressures, as taken from Gaillard et al. (2012).
View in Supplementary Information
The CO2-dominated vapour pulse is the composition of the vapour in equilibrium with this same mafic magma at 1000 bar from Gaillard et al. (2012), with its speciation and species fugacities recalculated for 10 or 100 bar using the thermodynamic data and models detailed above.
View in Supplementary Information
Holland, T.J.B., Powell, R. (1998) An internally consistent thermodynamic data set for phases of petrological interest. Journal of Metamorphic Geology 16, 309–343.

Thermodynamic properties required for these calculations were taken from Migdisov et al. (2013) for the Cu/Cl species, and from Robie and Hemingway (1995) and Holland and Powell (1998) for chalcopyrite, pyrite, Ni, NiO, magnetite, fayalite, quartz, HClgas, O2gas, and CO2gas.
View in Supplementary Information
Kestin, J., Sengers, J.V., Kamgar-Parsi, B., Sengers, J.M.H.L. (1984) Thermophysical Properties of Fluid H2O. Journal of Physical and Chemical Reference Data 13, 175, doi: 10.1063/1.555707.

Fugacity and partial pressures of pure water were calculated using the equation of state reported in Kestin et al. (1984); gaseous mixtures as well as the properties of pure HClgas, O2gas, and CO2gas were modelled using the PRSV equation of state (Stryjek and Vera, 1986); hydrated copper chlorides were assumed to obey the Ideal Gas Law.
View in Supplementary Information
Migdisov, A.A., Bychkov, A.Y., Williams-Jones, A.E., van Hinsberg, V.J. (2013) A predictive model for the transport of copper by HCl-bearing water vapour in ore-forming magmatic-hydrothermal systems: Implications for copper porphyry ore formation. Geochimica et Cosmochimica Acta 129, 33-53, doi: 10.1016/j.gca.2013.12.024.

Copper saturation of an HCl-bearing vapour with Cu-bearing species is controlled by the following reactions (Migdisov et al., 2013):
CuFeS2chalcopyrite + HClgas + 0.25O2gas + (n-0.5) H2Ogas = CuCl:(H2O)ngas + FeS2pyrite
CuFeS2chalcopyrite + 2HClgas + 0.25O2gas + 2H2Ogas = CuCl:HCl:(H2O)2.5gas + FeS2pyrite
View in Supplementary Information
Thermodynamic properties required for these calculations were taken from Migdisov et al. (2013) for the Cu/Cl species, and from Robie and Hemingway (1995) and Holland and Powell (1998) for chalcopyrite, pyrite, Ni, NiO, magnetite, fayalite, quartz, HClgas, O2gas, and CO2gas.
View in Supplementary Information
Robie, R.A., Hemingway, B.S. (1995) Thermodynamic Properties of Minerals and Related Substances at 298.15 K and 1 Bar (105 Pascals) Pressure and at Higher Temperatures. US Geological Survey Bulletin 2131, 1–470.

Thermodynamic properties required for these calculations were taken from Migdisov et al. (2013) for the Cu/Cl species, and from Robie and Hemingway (1995) and Holland and Powell (1998) for chalcopyrite, pyrite, Ni, NiO, magnetite, fayalite, quartz, HClgas, O2gas, and CO2gas.
View in Supplementary Information
Shvarov, Y., Bastrakov, E. (1999) HCh: a software package for geochemical equilibrium modeling - User's guide. Record 1999/025, Australian Geological Survey Organisation, Canberra.

The calculations were performed using the HCh software package for geochemical equilibrium modelling (Shvarov and Bastrakov, 1999).
View in Supplementary Information
Sillitoe, R.H. (2010) Porphyry Copper Systems. Economic Geology 105, 3–41.

Such sealed sections will no longer participate and are a well-established feature of many mineralisations, including porphyry Cu deposits as evidenced by multi-stage and crosscutting vein relationships (Sillitoe, 2010).
View in Supplementary Information
Deposition of metals in many ore-deposits, for example Cu-porphyry systems, is not pervasive, but concentrated in discrete veins (Sillitoe, 2010).
View in Supplementary Information
Simon, A.C., Pettke, T., Candela, P.A., Piccoli, P.M., Heinrich, C.A. (2006) Copper partitioning in a melt-vapor-brine-magnetite-pyrrhotite assemblage. Geochimica et Cocmochimica Acta 70, 5583-5600.

Reduced sulphur species are known to solvate Cu in aqueous vapour (e.g., Simon et al., 2006; Zajacz et al., 2011), and excluding these from the present calculations will thus underestimate the solubility of Cu.
View in Supplementary Information
Hydrated Cu-(H)xSy species, in particular, are regarded as important carriers of Cu in vapour (e.g., Simon et al., 2006; Zajacz et al., 2011).
View in Supplementary Information
Spilliaert, N., Metrich, N., Allard, P. (2006) S–Cl–F degassing pattern of water-rich alkali basalt: Modelling and relationship with eruption styles on Mount Etna volcano. Earth And Planetary Science Letters 248, 772–786.

However, the deep-degassed CO2-rich vapour pulse will have a lower HCl content, because Cl-solubility in the melt is strongly pressure dependent (Spilliaert et al., 2006).
View in Supplementary Information
Stryjek, R., Vera, J.H. (1986) PRSV: An improved peng-Robinson equation of state for pure compounds and mixtures. The Canadian Journal of Chemical Engineering 64, 323–333, doi: 10.1002/cjce.5450640224.

Fugacity and partial pressures of pure water were calculated using the equation of state reported in Kestin et al. (1984); gaseous mixtures as well as the properties of pure HClgas, O2gas, and CO2gas were modelled using the PRSV equation of state (Stryjek and Vera, 1986); hydrated copper chlorides were assumed to obey the Ideal Gas Law.
View in Supplementary Information
Witham, F., Blundy, J., Kohn, S.C., Lesne, P., Dixon, J., Churakov, S.V., Botcharnikov, R. (2012) SolEx: A model for mixed COHSCl-volatile solubilities and exsolved gas compositions in basalt. Computers & Geosciences 45, 87–97, doi: 10.1016/j.cageo.2011.09.021.

The model of Witham et al. (2012) predicts a more than tenfold decrease for a typical basalt when comparing the exsolved gas at 1000 bar to that at 10 bar, showing that this is a reasonable reduction.
View in Supplementary Information
Zajacz, Z., Seo, J.H., Candela, P., Piccoli, P.M., Tossell, J.A. (2011) The solubility of copper in high-temperature magmatic vapors: A quest for significance of various chloride and sulfide complexes. Geochimica et Cocmochimica Acta 75, 2811-2827.

Reduced sulphur species are known to solvate Cu in aqueous vapour (e.g., Simon et al., 2006; Zajacz et al., 2011), and excluding these from the present calculations will thus underestimate the solubility of Cu.
View in Supplementary Information
Hydrated Cu-(H)xSy species, in particular, are regarded as important carriers of Cu in vapour (e.g., Simon et al., 2006; Zajacz et al., 2011).
View in Supplementary Information