Carbon isotopic signatures of super-deep diamonds mediated by iron redox chemistry
Affiliations | Corresponding Author | Cite as | Funding information- Share this article
Article views:3,138Cumulative count of HTML views and PDF downloads.
- Download Citation
- Rights & Permissions
top
Abstract

Figures and Tables
![]() Figure 1 Partial phonon density of states (PDOS) of 57Fe (a, b) and 12C (c, d) in FeCO3 at high pressures. In (a) and (b), the open cycles are PDOS of Fe measured by NRIXS; the blue curves are calculated PDOS of Fe by DFT +U. The red curves in (c) and (d) are calculated PDOS of C. The lowest energy peak at 20-40 meV can be attributed to the acoustic phonons. According to previous high pressure Raman and infrared studies of FeCO3 (Santillán and Williams, 2004; Lin et al., 2012), the other peaks can be assigned to librational mode (L), translational mode (T) and in-plane bending mode (v4), out of plane bending vibration (v2) and asymmetric stretch (v3) of CO32- (marked as dashed vertical lines). The energies of v2 and v3 modes (marked as dotted vertical lines) at 60 GPa are linearly extrapolated from those measured up to 50 GPa for FeCO3 (Santillán and Williams, 2004). In the PDOS of Fe at 60 GPa (b), the splitting of v4 mode at approximately 100-120 meV has also been observed in a previous Raman study (Lin et al., 2012), which is explained as a result of the enhanced interaction between low-spin Fe2+ and neighbouring CO32- units. | ![]() Figure 2 Comparison of the histogram for δ13C of super-deep diamonds (left axis) and the probability density functions (PDFs, right axis) of δ13CDia derived from metallic melt for different P-T conditions. The red, green and black solid curves are calculated PDFs by using Δ13CDia-FeC melt at 250 km, 660 km and 1500 km depths along a cold slab geotherm (Yang et al., 2017), respectively. The yellow dotted curve is calculated by using Δ13CDia-FeC melt at 250 km along the modern mantle geotherm (Yang et al., 2017), which may be similar to the Archean mantle geotherm (Santosh et al., 2010). The pink dashed curve is for diamonds forming from C-H-O fluids, which is calculated using the largest reported value of Δ13CDia-COH (-2.9 ‰) (Cartigny et al., 2014). The inset figure shows the negative tailings of these three PDFs. δ13C data of super-deep diamonds are from Cartigny et al. (2014). |
Figure 1 | Figure 2 |
top
Introduction
Diamonds are prime recorders of the carbon isotopic compositions of the Earth because some of them are sourced deeply from the longest, isolated regions of Earth’s mantle (Cartigny et al., 2014
Cartigny, P., Palot, M., Thomassot, E., Harris, J.W. (2014) Diamond Formation: A Stable Isotope Perspective. Annual Review of Earth and Planetary Sciences 42, 699–732.
). The δ13C values (deviations in per mille of 13C/12C ratios relative to V-PDB) of natural diamonds show a broad range of variations from -41 ‰ to +3 ‰ with a mode at -5 ± 3 ‰ (Cartigny et al., 2014Cartigny, P., Palot, M., Thomassot, E., Harris, J.W. (2014) Diamond Formation: A Stable Isotope Perspective. Annual Review of Earth and Planetary Sciences 42, 699–732.
). Of particular interests are very low δ13C values of -26 ‰ to -41 ‰ found in some eclogitic and super-deep diamonds (e.g., De Stefano et al., 2009De Stefano, A., Kopylova, M.G., Cartigny, P., Afanasiev, V. (2009) Diamonds and eclogites of the Jericho kimberlite (Northern Canada). Contributions to Mineralogy and Petrology 158, 295–315.
; Smart et al., 2011Smart, K.A., Chacko, T., Stachel, T., Muehlenbachs, K., Stern, R.A., Heaman, L.M. (2011) Diamond growth from oxidized carbon sources beneath the Northern Slave Craton, Canada: A δ13C–N study of eclogite-hosted diamonds from the Jericho kimberlite. Geochimica et Cosmochimica Acta 75, 6027–6047.
; Smith et al., 2016Smith, E.M., Shirey, S.B., Nestola, F., Bullock, E.S., Wang, J.H., Richardson, S.H., Wang, W.Y. (2016) Large gem diamonds from metallic liquid in Earth’s deep mantle. Science 354, 1403–1405.
). These low δ13C values are most commonly found in eclogitic diamonds (e.g., Walter et al., 2011Walter, M.J., Kohn, S.C., Araujo, D., Bulanova, G.P., Smith, C.B., Gaillou, E., Wang, J., Steele, A., Shirey, S.B. (2011) Deep Mantle Cycling of Oceanic Crust: Evidence from Diamonds and Their Mineral Inclusions. Science 334, 54–57.
), which presumably incorporated a recycled oceanic crust component. It is thus unlikely that these δ13C values were inherited from Earth’s primordial materials. Although eclogitic diamonds with lowest δ13C values may originate from organic matter at 2.0-2.7 Ga (δ13C -40 ‰ to -60 ‰) (Smart et al., 2011Smart, K.A., Chacko, T., Stachel, T., Muehlenbachs, K., Stern, R.A., Heaman, L.M. (2011) Diamond growth from oxidized carbon sources beneath the Northern Slave Craton, Canada: A δ13C–N study of eclogite-hosted diamonds from the Jericho kimberlite. Geochimica et Cosmochimica Acta 75, 6027–6047.
), such organic matter unlikely survives at the depths (300-1000 km) where super-deep diamonds form (e.g., Anzolini et al., 2019Anzolini, C. et al. (2019) Depth of diamond formation obtained from single periclase inclusions. Geology 47, 219–222.
). Isotopic fractionation associated with diamond precipitation from either CH4 or CO2-bearing fluids (Galimov, 1991Galimov, E.M. (1991) Isotope fractionation related to kimberlite magmatism and diamond formation. Geochimica et Cosmochimica Acta 55, 1697–1708.
) is also an unlikely explanation for the most negative δ13C values measured in these diamonds. The reasons are that: (1) the equilibrium fractionation between diamond and CH4 at mantle temperatures (~+1 ‰) is too low to drive the residual fluid to very negative δ13C values by Rayleigh distillation; (2) the fractionation between diamond and CO2 of ~-3 ‰ at mantle temperatures could only produce diamonds whose δ13C values are ~-8 ‰ or higher. Overall, the question of how some super-deep diamonds acquired highly negative δ13C values is still open.Through plate tectonics, relatively oxidised iron and carbon species at the Earth’s surface are transported to the deep mantle by subducted slabs, where Fe2+ can disproportionate into Fe3+ and metallic Fe (Equation 1) below ~250 km due to stabilisation of Fe3+ in garnet, pyroxene and bridgmanite (Frost et al., 2004
Frost, D.J., Liebske, C., Langenhorst, F., McCammon, C.A., Tronnes, R.G., Rubie, D.C. (2004) Experimental evidence for the existence of iron-rich metal in the Earth’s lower mantle. Nature 428, 409–412.
; Rohrbach et al., 2007Rohrbach, A., Ballhaus, C., Golla–Schindler, U., Ulmer, P., Kamenetsky, V.S., Kuzmin, D.V. (2007) Metal saturation in the upper mantle. Nature 449, 456–458.
):Eq. 1
3FeO = Fe + Fe2O3
The resulting metallic Fe would react with carbonates to form either diamond (Equation 2) or iron carbide (Equation 3), depending on the local Fe:C ratio and thus redox state (Palyanov et al., 2013
Palyanov, Y.N., Bataleva, Y.V., Sokol, A.G., Borzdov, Y.M., Kupriyanov, I.N., Reutsky, V.N., Sobolev, N.V. (2013) Mantle-slab interaction and redox mechanism of diamond formation. Proceedings of the National Academy of Sciences of the United States of America 110, 20408–20413.
):Eq. 2
FeCO3 + 2Fe = 3FeO + C
Eq. 3
FeCO3 + 5Fe = 3FeO + Fe3C
Fe3C can also serve as a reduced C source to form diamonds through the following redox reaction (Bataleva et al., 2016
Bataleva, Y.V., Palyanov, Y.N., Borzdov, Y.M., Bayukov, O.A., Sobolev, N.V. (2016) Conditions for diamond and graphite formation from iron carbide at the P-T parameters of lithospheric mantle. Russian Geology and Geophysics 57, 176–189.
):Eq. 4
Fe3C + 3Fe2O3 = 9FeO + C
Moreover, Fe-C alloys/mixtures may melt under the pressure and temperature (P-T) conditions of the mantle because of their relatively low melting temperatures, especially in the presence of Ni, as compared to other mantle minerals (e.g., Rohrbach et al., 2014
Rohrbach, A., Ghosh, S., Schmidt, M.W., Wijbrans, C.H., Klemme, S. (2014) The stability of Fe–Ni carbides in the Earth’s mantle: Evidence for a low Fe–Ni–C melt fraction in the deep mantle. Earth and Planetary Science Letters 388, 211–221.
; Liu et al., 2016Liu, J., Li, J., Hrubiak, R., Smith, J.S. (2016) Origins of ultralow velocity zones through slab-derived metallic melt. Proceedings of the National Academy of Sciences 113, 5547–5551.
). The resultant Fe-C melt can form diamonds through the reaction mediated by iron redox chemistry:Eq. 5
Fe-C melt + Fe2O3 = 3FeO + C
The presence of S or other light elements can significantly lower C solubility in metallic melt and therefore promote diamond formation (Bataleva et al., 2015
Bataleva, Y.V., Palyanov, Y.N., Borzdov, Y.M., Bayukov, O.A., Sobolev, N.V. (2015) Interaction of iron carbide and sulfur under P–T conditions of the lithospheric mantle. Doklady Earth Sciences 463, 707–711.
). For example, Fe-Ni-S-C inclusions have been found in super-deep diamonds (e.g., Kaminsky and Wirth, 2011Kaminsky, F.V., Wirth, R. (2011) Iron Carbide Inclusions in Lower-Mantle Diamond from Juina, Brazil. Canadian Mineralogist 49, 555–572.
; Smith et al., 2016Smith, E.M., Shirey, S.B., Nestola, F., Bullock, E.S., Wang, J.H., Richardson, S.H., Wang, W.Y. (2016) Large gem diamonds from metallic liquid in Earth’s deep mantle. Science 354, 1403–1405.
). Finding such metallic inclusions requires careful examination as these inclusions are small in size (µm to nm scale) and can be mistaken for graphite (Kaminsky and Wirth, 2011Kaminsky, F.V., Wirth, R. (2011) Iron Carbide Inclusions in Lower-Mantle Diamond from Juina, Brazil. Canadian Mineralogist 49, 555–572.
; Smith et al., 2016Smith, E.M., Shirey, S.B., Nestola, F., Bullock, E.S., Wang, J.H., Richardson, S.H., Wang, W.Y. (2016) Large gem diamonds from metallic liquid in Earth’s deep mantle. Science 354, 1403–1405.
). The presence of metallic inclusions supports the view that C-bearing metallic melt could serve as a carbon source for some super-deep diamonds below ~250 km.Horita and Polyakov (2015)
Horita, J., Polyakov, V.B. (2015) Carbon-bearing iron phases and the carbon isotope composition of the deep Earth. Proceedings of the National Academy of Sciences 112, 31–36.
have attempted to address the aforementioned question through calculations of the reduced partition function ratio (β-factor) of C in Fe3C using the heat capacity and the iron phonon density of states (PDOS) at 1 bar. They combined this β-factor with previously published β-factors of diamond and carbonates to calculate the carbon equilibrium isotopic fractionation Δ13C between these phases,Eq. 6
Δ13CB-A = 1000(lnβB - lnβA)
where A and B are two phases in isotopic equilibrium. An important assumption that Horita and Polyakov (2015)
Horita, J., Polyakov, V.B. (2015) Carbon-bearing iron phases and the carbon isotope composition of the deep Earth. Proceedings of the National Academy of Sciences 112, 31–36.
made is that pressure has no effect on this fractionation. However, super-deep diamonds form under high P-T conditions in the mantle below 250 km depth, and applied pressure has undoubtedly been shown to stiffen lattice bonds and induce structural and electronic transitions, which in turn can affect β-factors of C in host phases (e.g., Lin et al., 2004Lin, J.-F., Struzhkin, V.V., Mao, H., Hemley, R.J., Chow, P., Hu, M.Y., Li, J. (2004) Magnetic transition in compressed Fe3C from x-ray emission spectroscopy. Physical Review B 70, 212405.
, 2012Lin, J.-F., Liu, J., Jacobs, C., Prakapenka, V.B. (2012) Vibrational and elastic properties of ferromagnesite across the electronic spin-pairing transition of iron. American Mineralogist 97, 583–591.
). In order to constrain reliably the extent of C isotopic fractionation during super-deep diamond formation, we used DFT augmented by a Hubbard U correction method (Giannozzi et al., 2009Giannozzi, P., Baroni, S., Bonini, N., Calandra, M. , Car, R., et al. (2009) QUANTUM ESPRESSO: a modular and open-source software project for quantum simulations of materials. Journal of Physics: Condensed Matter 21, 395502.
) to calculate the β-factors of C in MgCO3, FeCO3, Fe3C and diamond (Tables S-1, S-2) at the P-T conditions of subducted slabs in the mantle. We also measured the PDOS of Fe2+ in FeCO3 by nuclear resonant inelastic X-ray scattering (NRIXS) spectroscopy (Dauphas et al., 2018Dauphas, N., Hu, M.Y., Baker, E.M., Hu, J., Tissot, F.L.H., Alp, E.E., Roskosz, M., Zhao, J., Bi, W., Liu, J., J.-F., Niea, N.X., Heard, A. (2018) SciPhon: a data analysis software for nuclear resonant inelastic X-ray scattering with applications to Fe, Kr, Sn, Eu and Dy. Journal of Synchrotron Radiation 25, 1581–1599.
) to evaluate the accuracy of the theoretical calculations.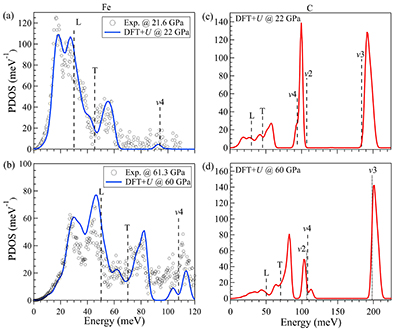
Figure 1 Partial phonon density of states (PDOS) of 57Fe (a, b) and 12C (c, d) in FeCO3 at high pressures. In (a) and (b), the open cycles are PDOS of Fe measured by NRIXS; the blue curves are calculated PDOS of Fe by DFT +U. The red curves in (c) and (d) are calculated PDOS of C. The lowest energy peak at 20-40 meV can be attributed to the acoustic phonons. According to previous high pressure Raman and infrared studies of FeCO3 (Santillán and Williams, 2004
Santillán, J., Williams, Q. (2004) A high-pressure infrared and X-ray study of FeCO3 and MnCO3: comparison with CaMg(CO3)2-dolomite. Physics of the Earth and Planetary Interiors 143–144, 291–304.
; Lin et al., 2012Lin, J.-F., Liu, J., Jacobs, C., Prakapenka, V.B. (2012) Vibrational and elastic properties of ferromagnesite across the electronic spin-pairing transition of iron. American Mineralogist 97, 583–591.
), the other peaks can be assigned to librational mode (L), translational mode (T) and in-plane bending mode (v4), out of plane bending vibration (v2) and asymmetric stretch (v3) of CO32- (marked as dashed vertical lines). The energies of v2 and v3 modes (marked as dotted vertical lines) at 60 GPa are linearly extrapolated from those measured up to 50 GPa for FeCO3 (Santillán and Williams, 2004Santillán, J., Williams, Q. (2004) A high-pressure infrared and X-ray study of FeCO3 and MnCO3: comparison with CaMg(CO3)2-dolomite. Physics of the Earth and Planetary Interiors 143–144, 291–304.
). In the PDOS of Fe at 60 GPa (b), the splitting of v4 mode at approximately 100-120 meV has also been observed in a previous Raman study (Lin et al., 2012Lin, J.-F., Liu, J., Jacobs, C., Prakapenka, V.B. (2012) Vibrational and elastic properties of ferromagnesite across the electronic spin-pairing transition of iron. American Mineralogist 97, 583–591.
), which is explained as a result of the enhanced interaction between low-spin Fe2+ and neighbouring CO32- units.top
PDOS of Fe and C in Minerals Relevant to Diamond Formation
The DFT + U calculation was verified by comparing the theoretical PDOS of Fe2+ in FeCO3 with the one measured by NRIXS (Fig .1). The PDOS results in theory and experiment match well with each other, which could also support the validity of the calculated β-factors of C in carbonates, diamond and nonmagnetic Fe3C (Fig. S-1). Synchrotron Mössbauer spectra (Fig. S-2) and optical images (Fig. S-3) show that the spin transition of Fe2+ in FeCO3 occurs between 44-46 GPa at 300 K. Across the spin transition, the unit cell volume collapses by 9.4 %, the Fe-O bond length is shortened by 4.8 % (Fig. S-4). Meanwhile, the spin transition of iron results in ~5 % decrease of the β-factor of C in LS FeCO3 compared to its HS state (Fig. S-5) as the C-O bound length is lengthened by 2.1 % (Fig. S-4). The magnetic state of Fe3C changes from ferromagnetic at ambient condition to paramagnetic and finally nonmagnetic at pressures higher than ~22-60 GPa (Lin et al., 2004
Lin, J.-F., Struzhkin, V.V., Mao, H., Hemley, R.J., Chow, P., Hu, M.Y., Li, J. (2004) Magnetic transition in compressed Fe3C from x-ray emission spectroscopy. Physical Review B 70, 212405.
; Gao et al., 2008Gao, L., Chen, B., Wang, J., Alp, E.E., Zhao, J., Lerche, M., Sturhahn, W., Scott, H.P., Huang, F., Ding, Y., Sinogeikin, S.V., Lundstrom, C.C., Bass, J.D., Li, J. (2008) Pressure-induced magnetic transition and sound velocities of Fe3C: Implications for carbon in the Earth’s inner core. Geophysical Research Letters 35, L17306.
). Therefore, nonmagnetic Fe3C is the relevant phase for most mantle depths. Similar to previous theoretical calculations (Horita and Polyakov, 2015Horita, J., Polyakov, V.B. (2015) Carbon-bearing iron phases and the carbon isotope composition of the deep Earth. Proceedings of the National Academy of Sciences 112, 31–36.
) and C isotopic measurements on natural diamonds and iron carbide inclusions (Mikhail et al., 2014Mikhail, S., Guillermier, C., Franchi, I.A., Beard, A.D., Crispin, K., Verchovsky, A.B., Jones, A.P., Milledge, H.J. (2014) Empirical evidence for the fractionation of carbon isotopes between diamond and iron carbide from the Earth’s mantle. Geochemistry, Geophysics, Geosystems 15, 855–866.
), the magnitude of ΔCDia-Fe3C is larger than other inter-mineral fractionations involving diamond, such as ΔCDia-Carbonates (Fig. S-6). Our calculated ΔCDia-Fe3C values along the representative P-T conditions of modern mantle and cold slab (Fig. S-6) are as much as 27 % lower than the 1 bar value of ΔCDia-Fe3C reported by Horita and Polyakov (2015)Horita, J., Polyakov, V.B. (2015) Carbon-bearing iron phases and the carbon isotope composition of the deep Earth. Proceedings of the National Academy of Sciences 112, 31–36.
. Therefore the 1 bar data would overestimate the C isotopic fractionation during diamond formation from a Fe3C source under representative mantle P-T conditions.top
Carbon Isotopic Fractionation in Diamonds through Redox Reactions
As discussed by Horita and Polyakov (2015)
Horita, J., Polyakov, V.B. (2015) Carbon-bearing iron phases and the carbon isotope composition of the deep Earth. Proceedings of the National Academy of Sciences 112, 31–36.
, the most significant reaction that can impart C isotopic fractionation to diamonds is one involving the oxidation of C alloyed with metallic melt to release C to form diamonds (Equation 5). To assess how redox reactions involving Fe-C melt below ~250 km can influence the δ13C values of super-deep diamonds, we modelled the isotopic fractionation of C between diamond and Fe-C melt. Our calculations accounting for various P-T and compositional factors allow us to test whether this diamond formation pathway can account for their δ13C values.The C isotopic fractionation can be modelled by using a Rayleigh distillation if the diamonds produced do not back-react with the C source, which is reasonable if the Fe-C source is a melt and the reaction product is a solid characterised by a low self-diffusivity (Koga et al., 2005
Koga, K.T., Walter, M.J., Nakamura, E., Kobayashi, K. (2005) Carbon self-diffusion in a natural diamond. Physical Review B 72, 024108.
). As it is challenging to calculate the β-factor of C in Fe-C melt directly, Δ13CDia-FeC melt is calculated by the sum of Δ13CDia-Fe3C (Fig. S-6) and the equilibrium fractionation Δ13CFe3C-FeC melt = -5.6 × 106/T2 anchored to the experimentally determined value of ~-2 ‰ at 6.3 GPa and 1673 K (Reutsky et al., 2015Reutsky, V.N., Borzdov, Y.M., Palyanov, Y.N. (2015) Carbon isotope fractionation during high pressure and high temperature crystallization of Fe–C melt. Chemical Geology 406, 18–24.
). By using this calculated Δ13CDia-FeC melt, δ13C values of diamonds forming from metallic melt can be calculated using a Rayleigh distillation model. Relative to the Fe-C melt source, the diamonds are enriched in the heavy isotope of C. Removal of isotopically heavy diamonds would have driven the Fe-C melt reservoir towards lower δ13C values and diamonds formed from this low δ13C reservoir progressively acquired more negative δ13C values.
Figure 2 Comparison of the histogram for δ13C of super-deep diamonds (left axis) and the probability density functions (PDFs, right axis) of δ13CDia derived from metallic melt for different P-T conditions. The red, green and black solid curves are calculated PDFs by using Δ13CDia-FeC melt at 250 km, 660 km and 1500 km depths along a cold slab geotherm (Yang et al., 2017
Yang, D., Wang, W., Wu, Z. (2017) Elasticity of superhydrous phase B at the mantle temperatures and pressures: Implications for 800 km discontinuity and water flow into the lower mantle. Journal of Geophysical Research: Solid Earth 122, 5026–5037.
), respectively. The yellow dotted curve is calculated by using Δ13CDia-FeC melt at 250 km along the modern mantle geotherm (Yang et al., 2017Yang, D., Wang, W., Wu, Z. (2017) Elasticity of superhydrous phase B at the mantle temperatures and pressures: Implications for 800 km discontinuity and water flow into the lower mantle. Journal of Geophysical Research: Solid Earth 122, 5026–5037.
), which may be similar to the Archean mantle geotherm (Santosh et al., 2010Santosh, M., Maruyama, S., Komiya, T., Yamamoto, S. (2010) Orogens in the evolving Earth: from surface continents to ‘lost continents’ at the core–mantle boundary. Geological Society, London, Special Publications 338, 77–116.
). The pink dashed curve is for diamonds forming from C-H-O fluids, which is calculated using the largest reported value of Δ13CDia-COH (-2.9 ‰) (Cartigny et al., 2014Cartigny, P., Palot, M., Thomassot, E., Harris, J.W. (2014) Diamond Formation: A Stable Isotope Perspective. Annual Review of Earth and Planetary Sciences 42, 699–732.
). The inset figure shows the negative tailings of these three PDFs. δ13C data of super-deep diamonds are from Cartigny et al. (2014)Cartigny, P., Palot, M., Thomassot, E., Harris, J.W. (2014) Diamond Formation: A Stable Isotope Perspective. Annual Review of Earth and Planetary Sciences 42, 699–732.
.The probability density function (PDF) of δ13CDia values of diamond formed from metallic melt, which we note as g(δ13CDia), is simply given as below (see detailed derivation in Supplementary Information),
Eq. 7
The PDFs for Δ13CDia-Fe3C at representative upper mantle, transition zone, and lower mantle depths are shown in Fig. 2. For each PDF, δ13C0Source is set to locate the maximum probability of δ13CDia at -5 ‰, which is the mode of δ13C of worldwide diamonds (Cartigny et al., 2014
Cartigny, P., Palot, M., Thomassot, E., Harris, J.W. (2014) Diamond Formation: A Stable Isotope Perspective. Annual Review of Earth and Planetary Sciences 42, 699–732.
). Because of the decrease of Δ13CDia-FeC melt with increasing depth and temperature, the extent of the negative tail of each PDF decreases with depth where diamonds form (Fig. 2). For example, the cumulative probability of δ13CDia values lower than -26 ‰ is ~0.17 at 250 km, and it decreases to 0.10 and 0.05 at 660 and 1500 km depths, respectively (Fig. S-7). This shows that super-deep diamonds with very light C isotope could have formed from C-bearing metallic melt in the reduced part (>250 km) of the upper mantle.top
Conclusion
By using the β-factors of carbon in relevant minerals/melt, the carbon isotopic fractionations between carbon-bearing phases possibly involved in super-deep diamond formation are calculated. The corresponding PDFs for δ13C of super-deep diamonds from different carbon sources are derived. Based on the comparison between the histogram for δ13C of super-deep diamonds and the derived PDFs, super-deep diamonds could crystallise from C-saturated metallic melt, thus supporting the existence of a deep mantle saturated in metallic iron.
top
Acknowledgements
W.Z. Wang and Z.Q. Wu acknowledge the Strategic Priority Research Program of the Chinese Academy of Sciences (XDB18000000), the Natural Science Foundation of China (41721002). J.F.L. and N.D. acknowledge support from CSEDI of the NSF Geophysics Program (EAR-1502594). NRIXS experiments used resources of the Advanced Photon Source, a U.S. Department of Energy (DOE) Office of Science User Facility operated for the DOE Office of Science by Argonne National Laboratory under Contract No. DE-AC02-06CH11357. W. Liang acknowledges the National Science Foundation for Young Scientists of China (41802044). W.L. Bi acknowledges COMPRES, the Consortium for Materials Properties Research in Earth Sciences under NSF Cooperative Agreement EAR 1606856. B. Chen acknowledges support from NSF grants EAR-1555388 and EAR-1565708.
Editor: Wendy Mao
top
Author Contributions
J.C. Liu and W.Z. Wang contributed equally to this work. J.F. Lin, J.C. Liu and W.Z. Wang designed this project. J.C. Liu, H. Yang, N. Dauphas, M.Y. Hu, J.Y. Zhao, W.L, Bi, E.E. Alp, W. Liang and B. Chen performed the experiments and data analysis. W.Z. Wang and Z.Q. Wu performed the calculations. J.C. Liu, W.Z. Wang, N. Dauphas and J.F. Lin wrote the paper.
top
References
Anzolini, C. et al. (2019) Depth of diamond formation obtained from single periclase inclusions. Geology 47, 219–222.

Although eclogitic diamonds with lowest δ13C values may originate from organic matter at 2.0-2.7 Ga (δ13C -40 ‰ to -60 ‰) (Smart et al., 2011), such organic matter unlikely survives at the depths (300-1000 km) where super-deep diamonds form (e.g., Anzolini et al., 2019).
View in article
Bataleva, Y.V., Palyanov, Y.N., Borzdov, Y.M., Bayukov, O.A., Sobolev, N.V. (2015) Interaction of iron carbide and sulfur under P–T conditions of the lithospheric mantle. Doklady Earth Sciences 463, 707–711.

The presence of S or other light elements can significantly lower C solubility in metallic melt and therefore promote diamond formation (Bataleva et al., 2015).
View in article
Bataleva, Y.V., Palyanov, Y.N., Borzdov, Y.M., Bayukov, O.A., Sobolev, N.V. (2016) Conditions for diamond and graphite formation from iron carbide at the P-T parameters of lithospheric mantle. Russian Geology and Geophysics 57, 176–189.

Fe3C can also serve as a reduced C source to form diamonds through the following redox reaction (Bataleva et al., 2016): Fe3C + 3Fe2O3 = 9FeO + C
View in article
Cartigny, P., Palot, M., Thomassot, E., Harris, J.W. (2014) Diamond Formation: A Stable Isotope Perspective. Annual Review of Earth and Planetary Sciences 42, 699–732.

Diamonds are prime recorders of the carbon isotopic compositions of the Earth because some of them are sourced deeply from the longest, isolated regions of Earth’s mantle (Cartigny et al., 2014).
View in article
The δ13C values (deviations in per mille of 13C/12C ratios relative to V-PDB) of natural diamonds show a broad range of variations from -41 ‰ to +3 ‰ with a mode at -5 ± 3 ‰ (Cartigny et al., 2014).
View in article
Figure 2 [...] The pink dashed curve is for diamonds forming from C-H-O fluids, which is calculated using the largest reported value of Δ13CDia-COH (-2.9 ‰) (Cartigny et al., 2014).
View in article
Figure 2 [...] δ13C data of super-deep diamonds are from Cartigny et al. (2014).
View in article
For each PDF, δ13C0Source is set to locate the maximum probability of δ13CDia at -5 ‰, which is the mode of δ13C of worldwide diamonds (Cartigny et al., 2014).
View in article
Dauphas, N., Hu, M.Y., Baker, E.M., Hu, J., Tissot, F.L.H., Alp, E.E., Roskosz, M., Zhao, J., Bi, W., Liu, J., J.-F., Niea, N.X., Heard, A. (2018) SciPhon: a data analysis software for nuclear resonant inelastic X-ray scattering with applications to Fe, Kr, Sn, Eu and Dy. Journal of Synchrotron Radiation 25, 1581–1599.

We also measured the PDOS of Fe2+ in FeCO3 by nuclear resonant inelastic X-ray scattering (NRIXS) spectroscopy (Dauphas et al., 2018) to evaluate the accuracy of the theoretical calculations.
View in article
De Stefano, A., Kopylova, M.G., Cartigny, P., Afanasiev, V. (2009) Diamonds and eclogites of the Jericho kimberlite (Northern Canada). Contributions to Mineralogy and Petrology 158, 295–315.

Of particular interests are very low δ13C values of -26 ‰ to -41 ‰ found in some eclogitic and super-deep diamonds (e.g., De Stefano et al., 2009; Smart et al., 2011; Smith et al., 2016).
View in article
Frost, D.J., Liebske, C., Langenhorst, F., McCammon, C.A., Tronnes, R.G., Rubie, D.C. (2004) Experimental evidence for the existence of iron-rich metal in the Earth’s lower mantle. Nature 428, 409–412.

Through plate tectonics, relatively oxidised iron and carbon species at the Earth’s surface are transported to the deep mantle by subducted slabs, where Fe2+ can disproportionate into Fe3+ and metallic Fe (Equation 1) below ~250 km due to stabilisation of Fe3+ in garnet, pyroxene and bridgmanite (Frost et al., 2004; Rohrbach et al., 2007): 3FeO = Fe + Fe2O3
View in article
Galimov, E.M. (1991) Isotope fractionation related to kimberlite magmatism and diamond formation. Geochimica et Cosmochimica Acta 55, 1697–1708.

Isotopic fractionation associated with diamond precipitation from either CH4 or CO2-bearing fluids (Galimov, 1991) is also an unlikely explanation for the most negative δ13C values measured in these diamonds.
View in article
Gao, L., Chen, B., Wang, J., Alp, E.E., Zhao, J., Lerche, M., Sturhahn, W., Scott, H.P., Huang, F., Ding, Y., Sinogeikin, S.V., Lundstrom, C.C., Bass, J.D., Li, J. (2008) Pressure-induced magnetic transition and sound velocities of Fe3C: Implications for carbon in the Earth’s inner core. Geophysical Research Letters 35, L17306.

The magnetic state of Fe3C changes from ferromagnetic at ambient condition to paramagnetic and finally nonmagnetic at pressures higher than ~22-60 GPa (Lin et al., 2004; Gao et al., 2008).
View in article
Giannozzi, P., Baroni, S., Bonini, N., Calandra, M. , Car, R., et al. (2009) QUANTUM ESPRESSO: a modular and open-source software project for quantum simulations of materials. Journal of Physics: Condensed Matter 21, 395502.

In order to constrain reliably the extent of C isotopic fractionation during super-deep diamond formation, we used DFT augmented by a Hubbard U correction method (Giannozzi et al., 2009) to calculate the β-factors of C in MgCO3, FeCO3, Fe3C and diamond (Tables S-1, S-2) at the P-T conditions of subducted slabs in the mantle.
View in article
Horita, J., Polyakov, V.B. (2015) Carbon-bearing iron phases and the carbon isotope composition of the deep Earth. Proceedings of the National Academy of Sciences 112, 31–36.

Horita and Polyakov (2015) have attempted to address the aforementioned question through calculations of the reduced partition function ratio (β-factor) of C in Fe3C using the heat capacity and the iron phonon density of states (PDOS) at 1 bar.
View in article
An important assumption that Horita and Polyakov (2015) made is that pressure has no effect on this fractionation.
View in article
Similar to previous theoretical calculations (Horita and Polyakov, 2015) and C isotopic measurements on natural diamonds and iron carbide inclusions (Mikhail et al., 2014), the magnitude of ΔCDia-Fe3C is larger than other inter-mineral fractionations involving diamond, such as ΔCDia-Carbonates (Fig. S-6).
View in article
Our calculated ΔCDia-Fe3C values along the representative P-T conditions of modern mantle and cold slab (Fig. S-6) are as much as 27 % lower than the 1 bar value of ΔCDia-Fe3C reported by Horita and Polyakov (2015).
View in article
As discussed by Horita and Polyakov (2015), the most significant reaction that can impart C isotopic fractionation to diamonds is one involving the oxidation of C alloyed with metallic melt to release C to form diamonds (Equation 5).
View in article
Kaminsky, F.V., Wirth, R. (2011) Iron Carbide Inclusions in Lower-Mantle Diamond from Juina, Brazil. Canadian Mineralogist 49, 555–572.

For example, Fe-Ni-S-C inclusions have been found in super-deep diamonds (e.g., Kaminsky and Wirth, 2011; Smith et al., 2016).
View in article
Finding such metallic inclusions requires careful examination as these inclusions are small in size (µm to nm scale) and can be mistaken for graphite (Kaminsky and Wirth, 2011; Smith et al., 2016).
View in article
Koga, K.T., Walter, M.J., Nakamura, E., Kobayashi, K. (2005) Carbon self-diffusion in a natural diamond. Physical Review B 72, 024108.

The C isotopic fractionation can be modelled by using a Rayleigh distillation if the diamonds produced do not back-react with the C source, which is reasonable if the Fe-C source is a melt and the reaction product is a solid characterised by a low self-diffusivity (Koga et al., 2005).
View in article
Lin, J.-F., Struzhkin, V.V., Mao, H., Hemley, R.J., Chow, P., Hu, M.Y., Li, J. (2004) Magnetic transition in compressed Fe3C from x-ray emission spectroscopy. Physical Review B 70, 212405.

However, super-deep diamonds form under high P-T conditions in the mantle below 250 km depth, and applied pressure has undoubtedly been shown to stiffen lattice bonds and induce structural and electronic transitions, which in turn can affect β-factors of C in host phases (e.g., Lin et al., 2004, 2012).
View in article
The magnetic state of Fe3C changes from ferromagnetic at ambient condition to paramagnetic and finally nonmagnetic at pressures higher than ~22-60 GPa (Lin et al., 2004; Gao et al., 2008).
View in article
Lin, J.-F., Liu, J., Jacobs, C., Prakapenka, V.B. (2012) Vibrational and elastic properties of ferromagnesite across the electronic spin-pairing transition of iron. American Mineralogist 97, 583–591.

However, super-deep diamonds form under high P-T conditions in the mantle below 250 km depth, and applied pressure has undoubtedly been shown to stiffen lattice bonds and induce structural and electronic transitions, which in turn can affect β-factors of C in host phases (e.g., Lin et al., 2004, 2012).
View in article
Figure 1 [...] According to previous high pressure Raman and infrared studies of FeCO3 (Santillán and Williams, 2004; Lin et al., 2012), the other peaks can be assigned to librational mode (L), translational mode (T) and in-plane bending mode (v4), out of plane bending vibration (v2) and asymmetric stretch (v3) of CO32- (marked as dashed vertical lines).
View in article
Figure 1 [...] In the PDOS of Fe at 60 GPa (b), the splitting of v4 mode at approximately 100-120 meV has also been observed in a previous Raman study (Lin et al., 2012), which is explained as a result of the enhanced interaction between low-spin Fe2+ and neighbouring CO32- units.
View in article
Liu, J., Li, J., Hrubiak, R., Smith, J.S. (2016) Origins of ultralow velocity zones through slab-derived metallic melt. Proceedings of the National Academy of Sciences 113, 5547–5551.

Moreover, Fe-C alloys/mixtures may melt under the pressure and temperature (P-T) conditions of the mantle because of their relatively low melting temperatures, especially in the presence of Ni, as compared to other mantle minerals (e.g., Rohrbach et al., 2014; Liu et al., 2016).
View in article
Mikhail, S., Guillermier, C., Franchi, I.A., Beard, A.D., Crispin, K., Verchovsky, A.B., Jones, A.P., Milledge, H.J. (2014) Empirical evidence for the fractionation of carbon isotopes between diamond and iron carbide from the Earth’s mantle. Geochemistry, Geophysics, Geosystems 15, 855–866.

Similar to previous theoretical calculations (Horita and Polyakov, 2015) and C isotopic measurements on natural diamonds and iron carbide inclusions (Mikhail et al., 2014), the magnitude of ΔCDia-Fe3C is larger than other inter-mineral fractionations involving diamond, such as ΔCDia-Carbonates (Fig. S-6).
View in article
Palyanov, Y.N., Bataleva, Y.V., Sokol, A.G., Borzdov, Y.M., Kupriyanov, I.N., Reutsky, V.N., Sobolev, N.V. (2013) Mantle-slab interaction and redox mechanism of diamond formation. Proceedings of the National Academy of Sciences of the United States of America 110, 20408–20413.

The resulting metallic Fe would react with carbonates to form either diamond (Equation 2) or iron carbide (Equation 3), depending on the local Fe:C ratio and thus redox state (Palyanov et al., 2013): FeCO3 + 2Fe = 3FeO + C
View in article
Reutsky, V.N., Borzdov, Y.M., Palyanov, Y.N. (2015) Carbon isotope fractionation during high pressure and high temperature crystallization of Fe–C melt. Chemical Geology 406, 18–24.

As it is challenging to calculate the β-factor of C in Fe-C melt directly, Δ13CDia-FeC melt is calculated by the sum of Δ13CDia-Fe3C (Fig. S-6) and the equilibrium fractionation Δ13CFe3C-FeC melt = -5.6 × 106/T2 anchored to the experimentally determined value of ~-2 ‰ at 6.3 GPa and 1673 K (Reutsky et al., 2015).
View in article
Rohrbach, A., Ballhaus, C., Golla–Schindler, U., Ulmer, P., Kamenetsky, V.S., Kuzmin, D.V. (2007) Metal saturation in the upper mantle. Nature 449, 456–458.

Through plate tectonics, relatively oxidised iron and carbon species at the Earth’s surface are transported to the deep mantle by subducted slabs, where Fe2+ can disproportionate into Fe3+ and metallic Fe (Equation 1) below ~250 km due to stabilisation of Fe3+ in garnet, pyroxene and bridgmanite (Frost et al., 2004; Rohrbach et al., 2007): 3FeO ↔ Fe + Fe2O3
View in article
Rohrbach, A., Ghosh, S., Schmidt, M.W., Wijbrans, C.H., Klemme, S. (2014) The stability of Fe–Ni carbides in the Earth’s mantle: Evidence for a low Fe–Ni–C melt fraction in the deep mantle. Earth and Planetary Science Letters 388, 211–221.

Moreover, Fe-C alloys/mixtures may melt under the pressure and temperature (P-T) conditions of the mantle because of their relatively low melting temperatures, especially in the presence of Ni, as compared to other mantle minerals (e.g., Rohrbach et al., 2014; Liu et al., 2016).
View in article
Santillán, J., Williams, Q. (2004) A high-pressure infrared and X-ray study of FeCO3 and MnCO3: comparison with CaMg(CO3)2-dolomite. Physics of the Earth and Planetary Interiors 143–144, 291–304.

Figure 1 [...] According to previous high pressure Raman and infrared studies of FeCO3 (Santillán and Williams, 2004; Lin et al., 2012), the other peaks can be assigned to librational mode (L), translational mode (T) and in-plane bending mode (v4), out of plane bending vibration (v2) and asymmetric stretch (v3) of CO32- (marked as dashed vertical lines).
View in article
Figure 1 [...] The energies of v2 and v3 modes (marked as dotted vertical lines) at 60 GPa are linearly extrapolated from those measured up to 50 GPa for FeCO3 (Santillán and Williams, 2004).
View in article
Santosh, M., Maruyama, S., Komiya, T., Yamamoto, S. (2010) Orogens in the evolving Earth: from surface continents to ‘lost continents’ at the core–mantle boundary. Geological Society, London, Special Publications 338, 77–116.

Figure 2 [...] The yellow dotted curve is calculated by using Δ13CDia-FeC melt at 250 km along the modern mantle geotherm (Yang et al., 2017), which may be similar to the Archean mantle geotherm (Santosh et al., 2010).
View in article
Smart, K.A., Chacko, T., Stachel, T., Muehlenbachs, K., Stern, R.A., Heaman, L.M. (2011) Diamond growth from oxidized carbon sources beneath the Northern Slave Craton, Canada: A δ13C–N study of eclogite-hosted diamonds from the Jericho kimberlite. Geochimica et Cosmochimica Acta 75, 6027–6047.

Of particular interests are very low δ13C values of -26 ‰ to -41 ‰ found in some eclogitic and super-deep diamonds (e.g., De Stefano et al., 2009; Smart et al., 2011; Smith et al., 2016).
View in article
Although eclogitic diamonds with lowest δ13C values may originate from organic matter at 2.0-2.7 Ga (δ13C -40 ‰ to -60 ‰) (Smart et al., 2011), such organic matter unlikely survives at the depths (300-1000 km) where super-deep diamonds form (e.g., Anzolini et al., 2019).
View in article
Smith, E.M., Shirey, S.B., Nestola, F., Bullock, E.S., Wang, J.H., Richardson, S.H., Wang, W.Y. (2016) Large gem diamonds from metallic liquid in Earth’s deep mantle. Science 354, 1403–1405.

Of particular interests are very low δ13C values of -26 ‰ to -41 ‰ found in some eclogitic and super-deep diamonds (e.g., De Stefano et al., 2009; Smart et al., 2011; Smith et al., 2016).
View in article
For example, Fe-Ni-S-C inclusions have been found in super-deep diamonds (e.g., Kaminsky and Wirth, 2011; Smith et al., 2016).
View in article
Finding such metallic inclusions requires careful examination as these inclusions are small in size (µm to nm scale) and can be mistaken for graphite (Kaminsky and Wirth, 2011; Smith et al., 2016).
View in article
Walter, M.J., Kohn, S.C., Araujo, D., Bulanova, G.P., Smith, C.B., Gaillou, E., Wang, J., Steele, A., Shirey, S.B. (2011) Deep Mantle Cycling of Oceanic Crust: Evidence from Diamonds and Their Mineral Inclusions. Science 334, 54–57.

These low δ13C values are most commonly found in eclogitic diamonds (e.g., Walter et al., 2011), which presumably incorporated a recycled oceanic crust component.
View in article
Yang, D., Wang, W., Wu, Z. (2017) Elasticity of superhydrous phase B at the mantle temperatures and pressures: Implications for 800 km discontinuity and water flow into the lower mantle. Journal of Geophysical Research: Solid Earth 122, 5026–5037.

Figure 2 [...] The red, green and black solid curves are calculated PDFs by using Δ13CDia-FeC melt at 250 km, 660 km and 1500 km depths along a cold slab geotherm (Yang et al., 2017), respectively.
View in article
Figure 2 [...] The yellow dotted curve is calculated by using Δ13CDia-FeC melt at 250 km along the modern mantle geotherm (Yang et al., 2017), which may be similar to the Archean mantle geotherm (Santosh et al., 2010).
View in article
top
Supplementary Information
The Supplementary Information includes:
- 1. High-Pressure Experiment
- 2. First-Principles Calculations
- 3. C Isotope Model
- Tables S-1 and S-2
- Figures S-1 to S-9
- Supplementary Information References
Download the Supplementary Information (PDF)
Figures and Tables
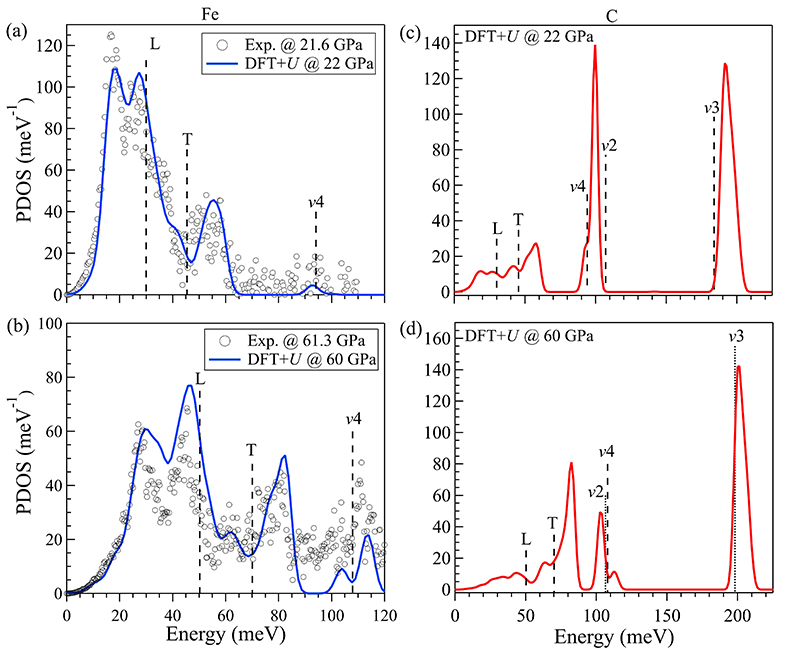
Figure 1 Partial phonon density of states (PDOS) of 57Fe (a, b) and 12C (c, d) in FeCO3 at high pressures. In (a) and (b), the open cycles are PDOS of Fe measured by NRIXS; the blue curves are calculated PDOS of Fe by DFT + U. The red curves in (c) and (d) are calculated PDOS of C. The lowest energy peak at 20-40 meV can be attributed to the acoustic phonons. According to previous high pressure Raman and infrared studies of FeCO3 (Santillán and Williams, 2004
Santillán, J., Williams, Q. (2004) A high-pressure infrared and X-ray study of FeCO3 and MnCO3: comparison with CaMg(CO3)2-dolomite. Physics of the Earth and Planetary Interiors 143–144, 291–304.
; Lin et al., 2012Lin, J.-F., Liu, J., Jacobs, C., Prakapenka, V.B. (2012) Vibrational and elastic properties of ferromagnesite across the electronic spin-pairing transition of iron. American Mineralogist 97, 583–591.
), the other peaks can be assigned to librational mode (L), translational mode (T) and in-plane bending mode (v4), out of plane bending vibration (v2) and asymmetric stretch (v3) of CO32- (marked as dashed vertical lines). The energies of v2 and v3 modes (marked as dotted vertical lines) at 60 GPa are linearly extrapolated from those measured up to 50 GPa for FeCO3 (Santillán and Williams, 2004Santillán, J., Williams, Q. (2004) A high-pressure infrared and X-ray study of FeCO3 and MnCO3: comparison with CaMg(CO3)2-dolomite. Physics of the Earth and Planetary Interiors 143–144, 291–304.
). In the PDOS of Fe at 60 GPa (b), the splitting of v4 mode at approximately 100-120 meV has also been observed in a previous Raman study (Lin et al., 2012Lin, J.-F., Liu, J., Jacobs, C., Prakapenka, V.B. (2012) Vibrational and elastic properties of ferromagnesite across the electronic spin-pairing transition of iron. American Mineralogist 97, 583–591.
), which is explained as a result of the enhanced interaction between low-spin Fe2+ and neighbouring CO32- units.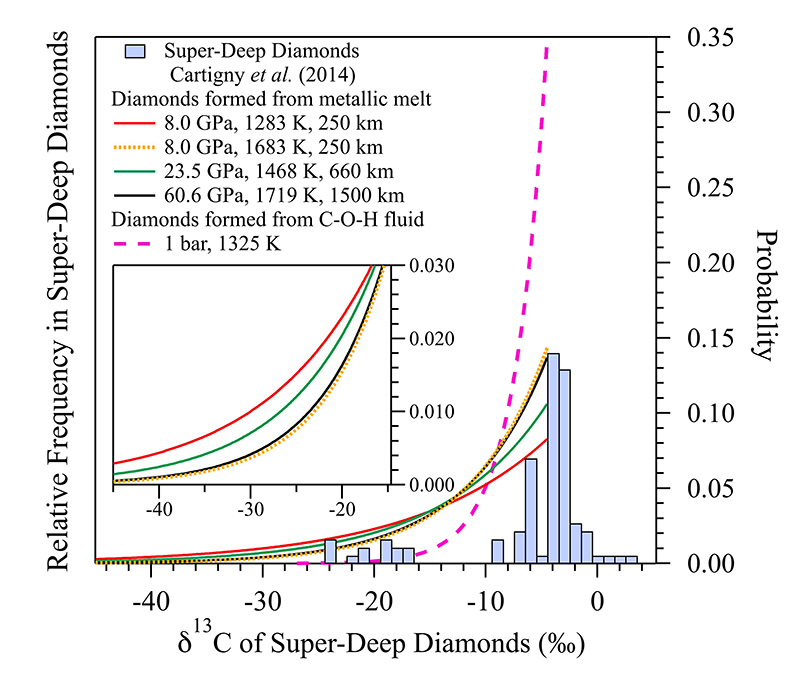
Figure 2 Comparison of the histogram for δ13C of super-deep diamonds (left axis) and the probability density functions (PDFs, right axis) of δ13CDia derived from metallic melt for different P-T conditions. The red, green and black solid curves are calculated PDFs by using Δ13CDia-FeC melt at 250 km, 660 km and 1500 km depths along a cold slab geotherm (Yang et al., 2017
Yang, D., Wang, W., Wu, Z. (2017) Elasticity of superhydrous phase B at the mantle temperatures and pressures: Implications for 800 km discontinuity and water flow into the lower mantle. Journal of Geophysical Research: Solid Earth 122, 5026–5037.
), respectively. The yellow dotted curve is calculated by using Δ13CDia-FeC melt at 250 km along the modern mantle geotherm (Yang et al., 2017Yang, D., Wang, W., Wu, Z. (2017) Elasticity of superhydrous phase B at the mantle temperatures and pressures: Implications for 800 km discontinuity and water flow into the lower mantle. Journal of Geophysical Research: Solid Earth 122, 5026–5037.
), which may be similar to the Archean mantle geotherm (Santosh et al., 2010Santosh, M., Maruyama, S., Komiya, T., Yamamoto, S. (2010) Orogens in the evolving Earth: from surface continents to ‘lost continents’ at the core–mantle boundary. Geological Society, London, Special Publications 338, 77–116.
). The pink dashed curve is for diamonds forming from C-H-O fluids, which is calculated using the largest reported value of Δ13CDia-COH (-2.9 ‰) (Cartigny et al., 2014Cartigny, P., Palot, M., Thomassot, E., Harris, J.W. (2014) Diamond Formation: A Stable Isotope Perspective. Annual Review of Earth and Planetary Sciences 42, 699–732.
). The inset figure shows the negative tailings of these three PDFs. δ13C data of super-deep diamonds are from Cartigny et al. (2014)Cartigny, P., Palot, M., Thomassot, E., Harris, J.W. (2014) Diamond Formation: A Stable Isotope Perspective. Annual Review of Earth and Planetary Sciences 42, 699–732.
.