Mercury reallocation in thawing subarctic peatlands
Affiliations | Corresponding Author | Cite as | Funding information- Share this article
Article views:5,217Cumulative count of HTML views and PDF downloads.
- Download Citation
- Rights & Permissions
top
Abstract

Figures and Tables
![]() Figure 1 (a) Relationships between TGM flux and ambient air temperature (°C) and (b) photosynthetically active radiation (PAR). Symbols denote palsa (brown squares), bog (green triangles) and fen (blue diamonds) and include least squares linear regressions and associated r2 and p values. Evasion of TGM interpreted for values greater than zero and deposition for values less than zero. | ![]() Figure 2 Summarised Hg in the three sub-habitats (from top to bottom): TGM, porewater methyl Hg (MeHg), and total peat Hg (corrected for dry bulk density). | ![]() Figure 3 Mean relative abundance of potential Hg methylators at Stordalen Mire (n = 38 for palsa, 47 for bog, and 42 for fen), using microbial community data from 2010-2012 reanalysed from Mondav et al. (2017). * indicates genera with members experimentally confirmed to methylate Hg. | ![]() Figure 4 Schematic of biogeochemical Hg cycling across permafrost thaw with emphasis on Hg pools and major pathways. Red arrows denote gaseous (Hg0) flux for each stage of the thaw sequence. Peat inventories are for total Hg for the top 40 cm of the mire surface (cf. legend for corresponding range). |
Figure 1 | Figure 2 | Figure 3 | Figure 4 |
top
Introduction
Anthropogenic Hg emissions have increased Hg deposition to the Arctic three-fold since the Industrial Revolution (Fitzgerald et al., 2005
Fitzgerald, W.F., Engstrom, D.R., Lamborg, C.H., Tseng, C., Balcom, P.H., Hammerschmidt, C.R. (2005) Modern and historic atmospheric mercury fluxes in northern Alaska: Global sources and arctic depletion. Environmental Science & Technology 39, 557–568.
). Terrestrial ecosystems are generally net sinks for atmospheric Hg deposition (Selin, 2009Selin, N.E. (2009) Global biogeochemical cycling of mercury: a review. Annual Review of Environment and Resources 34, 43–63.
), and Hg sequestration in soil is positively correlated with organic matter content (Grigal, 2003Grigal, D.F. (2003) Mercury sequestration in forests and peatlands: a review. Journal of Environmental Quality 32, 393–405.
; Smith-Downey et al., 2010Smith-Downey, N.V., Sunderland, E.M., Jacob, D.J. (2010) Anthropogenic impacts on global storage and emissions of mercury from terrestrial soils: Insights from a new global model. Journal of Geophysical Research: Biogeosciences 115, G03008.
). Permafrost constitutes the largest long term reservoir of Hg, containing twice that of all other reservoirs combined (Schuster et al., 2018Schuster, P.F., Schaefer, K.M., Aiken, G.R., Antweiler, R.C., Dewild, J.F., Gryziec, J.D., Gusmeroli, A., Hugelius, G., Jafarov, E., Krabbenhoft, D.P., Liu, L., Herman-Mercer, N., Mu, C., Roth, D.A., Schaefer, T., Striegl, R.G., Wickland, K.P., Zhang, T. (2018) Permafrost Stores a Globally Significant Amount of Mercury. Geophysical Research Letters 45, 1463–1471.
and references therein). Changes in the organic carbon (OC) balance from thawing permafrost, coupled with changing redox conditions associated with increases in soil moisture result in the release of labile OC (Hodgkins et al., 2014Hodgkins, S.B., Tfaily, M.M., McCalley, C.K., Logan, T.A., Crill, P.M., Saleska, S.R., Rich, V.I., Chanton, J.P. (2014) Changes in peat chemistry associated with permafrost thaw increase greenhouse gas production. Proceedings of the National Academy of Science 111, 5819–5824.
) that likely drives Hg export (Mu et al., 2019Mu, C., Zhang, F., Chen, X., Ge, S., Mu, M., Jia, L., Wu, Q., Zhang, T. (2019) Carbon and mercury export from the Arctic rivers and response to permafrost degradation. Water Research 161, 54–60.
). Reducing conditions favoured in fen environments also influence the bioavailability of Hg via binding to thiol functional groups (Skyllberg et al., 2003Skyllberg, U., Qian J., Frech, W., Xia, K., Bleam, W.F. (2003) Distribution of mercury, methyl mercury and organic sulphur species in soil, soil solution and stream of a boreal forest catchment. Biogeochemistry 64, 53–76.
). Studies of northern peatlands find that Hg export accompanies permafrost thaw associated with climate change (e.g., Rydberg et al., 2010Rydberg, J., Klaminder, J., Rosén, P., Bindler, R. (2010) Climate driven release of carbon and mercury from permafrost mires increases mercury loading to sub-arctic lakes. Science of The Total Environment 408, 4778–4783.
; St. Pierre et al. 2018St. Pierre, K.A., Zolkos, S., Shakil, S., Tank, S.E., St. Louis, V.L., Kokelj, S.V. (2018) Unprecedented Increases in Total and Methyl Mercury Concentrations Downstream of Retrogressive Thaw Slumps in the Western Canadian Arctic. Environmental Science & Technology 52, 14099–14109.
; Mu et al., 2019Mu, C., Zhang, F., Chen, X., Ge, S., Mu, M., Jia, L., Wu, Q., Zhang, T. (2019) Carbon and mercury export from the Arctic rivers and response to permafrost degradation. Water Research 161, 54–60.
). Increasing Arctic temperatures also contribute to significant changes in microbial communities and activity which can influence the production of methyl Hg (MeHg), a powerful neurotoxin (Barkay et al., 2011Barkay, T., Kroer, N., Poulain, A.J. (2011) Some like it cold: microbial transformations of mercury in polar regions. Polar Research 30, 15469.
and references therein; Yang et al., 2016Yang, Z., Fang, W., Lu, X., Sheng, G.P., Graham, D.E., Liang, L., Wullschleger, S.D., Gu, B. (2016) Warming increases methylmercury production in an Arctic soil. Environmental Pollution 214, 504–509.
). In this study we present a holistic view of Hg cycling across a permafrost thaw gradient that addresses the interactions of atmospheric, hydrologic and geochemical processes with ecosystem response to changing the distribution and methylating potential of Hg under permafrost thaw (detailed methods descriptions can be found in Supplementary Information).Stordalen Mire in Abisko, Sweden (68° 22’ N, 19° 03’ E) contains distinct sub-habitats sampled for this study. These representative sub-habitats along a permafrost thaw gradient common to northern peatlands include: i) permafrost-dominated, well-drained palsas occupied by feather mosses and ericaceous and woody plants (ii) intermediate permafrost sites with variable water table depth, dominated by Sphagnum spp. (iii) full summer-thaw, fully submerged sites with Eriophorum angustifolium (Fig. S-1). Between 1970 and 2000, permafrost thaw and palsa collapse led to the expansion of Sphagnum (bog) and Eriophorum (fen) by 3 % and 54 %, respectively (Johansson et al., 2006a
Johansson, T., Malmer, N., Crill, P.M., Friborg, T., Åkerman, J.H., Mastepanov, M., Christensen, T.R. (2006a) Decadal vegetation changes in a northern peatland, greenhouse gas fluxes and net radiative forcing. Global Change Biology 12, 2352–2369.
). Linking thawing permafrost with hydrologic change (Johansson et al., 2006bJohansson, M., Christensen, T.R., Åkerman, H.J., Callaghan, T.V. (2006b) What Determines the Current Presence or Absence of Permafrost in the Torneträsk Region, a Sub-arctic Landscape in Northern Sweden? AMBIO: A Journal of the Human Environment 35, 190–197.
), vegetation shifts over a 30 year period (Malmer et al., 2005Malmer, N., Johansson, T., Olsrud, M., Christensen, T.R. (2005) Vegetation, climatic changes and net carbon sequestration in a North-Scandinavian subarctic mire over 30 years. Global Change Biology 11, 1895–1909.
; Johansson et al., 2006aJohansson, T., Malmer, N., Crill, P.M., Friborg, T., Åkerman, J.H., Mastepanov, M., Christensen, T.R. (2006a) Decadal vegetation changes in a northern peatland, greenhouse gas fluxes and net radiative forcing. Global Change Biology 12, 2352–2369.
) and carbon dynamics in a mire (e.g., Öquist and Svensson, 2002Öquist, M.G., Svensson, B.H. (2002) Vascular plants as regulators of methane emissions from a subarctic mire ecosystem. Journal of Geophysical Research 107, 4580.
; Bäckstrand et al., 2010Bäckstrand, K., Crill, P.M., Jackowicz-Korczyñski, M., Mastepanov, M., Christensen, T.R., Bastviken, D. (2010) Annual carbon gas budget for a subarctic peatland, Northern Sweden. Biogeosciences 7, 95–108.
) provide essential context to develop a predictive framework for Hg cycling in the future thawed permafrost.top
Results and Discussion
Mechanisms driving Hg cycling in Arctic ecosystems
1. Evasion. Full quantification of Hg mobilisation requires an assessment of the dynamics of Hg exchange at the atmosphere-land interface. During three days of dynamic chamber flux measurements (in the peak of the growing season) all three sites exhibited a diel pattern in TGM fluxes, as shown in previous studies (Carpi and Lindberg, 1997
Carpi, A., Lindberg, S.E. (1997) Sunlight-Mediated emission of elemental mercury from soil amended with municipal sewage sludge. Environmental Science & Technology 31, 2085–2091.
; Poissant et al., 2004Poissant, L., Pilote, M., Xu, X., Zhang, H., Beauvais, C. (2004) Mercury Gas Exchanges over Selected Bare Soil and Flooded Sites in the Bay St. François Wetlands (Québec, Canada). Atmospheric Environment 38, 4205–4214.
; chamber flux measurement approach described in Supplementary Information). Evasion of TGM occurred during warmer, peak daytime PAR while TGM deposition occurred during cooler, low PAR (<400) periods (Fig. 1). The palsa site yielded the highest TGM evasion coupled with the least amount of Hg deposition during the low PAR hours. The bog exhibited the most variability of the three sites. The strong correlation between TGM flux and PAR in the bog site is consistent with earlier interpretations (Klaminder et al., 2008Klaminder, J., Yoo, K., Rydberg, J., Giesler, R. (2008) An explorative study of mercury export from a thawing palsa mire. Journal of Geophysical Research: Biogeosciences 113, G04034.
) of increased photoreduction reactions in water-saturated soils, resulting from permafrost thaw, leading to lower peat Hg concentrations (Gustin et al., 2006Gustin, M.S., Engle, M., Ericksen, J., Lyman, S., Stamenkovi, J., Xin, M. (2006) Mercury exchange between the atmosphere and low mercury containing substrates. Applied Geochemistry 21, 1913–1923.
). The bog TGM flux, together with lower HgTot concentrations deeper in the peat, suggest significant Hg loss (Klaminder et al., 2008Klaminder, J., Yoo, K., Rydberg, J., Giesler, R. (2008) An explorative study of mercury export from a thawing palsa mire. Journal of Geophysical Research: Biogeosciences 113, G04034.
), with the semi-wet or intermediate stage of the thaw resulting in less Hg retention in soils and vegetation (Fig. 2). The fen site experienced both the lowest TGM evasion and the highest TGM deposition (most negative fluxes). Some studies have found that biotic demethylation of Hg can be a significant source of evasion (Pannu et al., 2014Pannu, R., Siciliano, S.D., O'Driscoll, N.J. (2014) Quantifying the effects of soil temperature, moisture and sterilization on elemental mercury formation in boreal soils. Environmental Pollution 193, 138.
and references therein). Both the fen and the palsa sites showed stronger relationships between air temperature and TGM flux and this may influence both biotic and abiotic reactions (Fig. 1).
Figure 1 (a) Relationships between TGM flux and ambient air temperature (°C) and (b) photosynthetically active radiation (PAR). Symbols denote palsa (brown squares), bog (green triangles) and fen (blue diamonds) and include least squares linear regressions and associated r2 and p values. Evasion of TGM interpreted for values greater than zero and deposition for values less than zero.

Figure 2 Summarised Hg in the three sub-habitats (from top to bottom): TGM, porewater methyl Hg (MeHg), and total peat Hg (corrected for dry bulk density).
2. Soil carbon labileness and sulphur redox. The three habitat types representing a permafrost thaw gradient were also characterised by markedly different peat HgTot concentrations. The palsa site had the highest overall HgTot with concentrations ranging from 257 µg kg-1 near the surface and decreasing to 15 µg kg-1 with depth to the active layer boundary (44 cm from surface) (Fig. S-4). The bog site had the lowest overall HgTot (concentrations from 10-75 μg kg-1). The fully thawed fen habitat had intermediate HgTot concentrations (10-165 μg kg-1), with values decreasing gradually with depth as in the palsa. Relative HgTot pools estimated by combining bulk density at each site (profiled at ~5 cm increments) with the HgTot measurements (details in Supplementary Information) followed the same pattern, with; palsa (2.7 mg m-2), bog (1.4 mg m-2) and fen (4.7 mg m-2) (Fig. 2). These findings suggest that the Hg liberated from thawing palsa has two potential pathways of reallocation; palsa may thaw directly to a bog and from there result in Hg export, or alternatively palsa may transition directly to a fen where Hg accumulation is enhanced.
Total peat sulphur (S) concentrations (Fig. S-4) provide additional evidence pointing to HgTot accumulation. The surface of the fen sites (~0-1 cm depths) contain almost double the amount of S relative to both the palsa and the bog sites. At the fen, total Hg shows a strong relationship with C, N and S (r2 = 0.73, 0.77, 0.82), whereas in the bog and palsa the correlations are weak or insignificant (Fig. S-5). In reducing environments, S in peat forms organo-sulphides, especially in the presence of hydrogen sulphide (Schartup et al., 2014
Schartup, A.T., Balcom, P.H., Mason, R.P. (2014) Sediment-porewater partitioning, total sulfur, and methylmercury production in estuaries. Environmental Science & Technology 48, 954–960.
) which enhances storage of Hg.The degree to which these dynamic landscapes might serve as sources of the biologically available and toxic MeHg was evaluated through the analysis of porewater chemistry. In addition to enhanced peat Hg accumulation in the fen, we also found elevated concentrations of MeHg in fen porewater that were similar to those reported in other wetland studies (e.g., Tjerngren et al., 2012
Tjerngren, I., Karlsson, T., Bjorn, E., Skyllberg, U. (2012) Potential Hg methylation and MeHg demethylation rates related to the nutrient status of different boreal wetlands. Biogeochemistry 108, 335–350.
). This pattern was in contrast to the notably lower porewater MeHg concentrations in the bog site (Table S-1). The methylation of Hg in the mire may be a function of bioavailable Hg and/or linked with the ability of the microbial community to methylate Hg. Differences in redox conditions, organic matter structure and composition, and microbial communities between bog and fen sites may explain the observed differences in MeHg. The presence of higher total S in the fen site may also enhance the bioavailability of ionic Hg leading to methylation (Skyllberg et al., 2003Skyllberg, U., Qian J., Frech, W., Xia, K., Bleam, W.F. (2003) Distribution of mercury, methyl mercury and organic sulphur species in soil, soil solution and stream of a boreal forest catchment. Biogeochemistry 64, 53–76.
). Additionally, the Hg bound to more labile OC may be more easily methylated by microbes than Hg bound to more recalcitrant C. Hodgkins et al. (2014)Hodgkins, S.B., Tfaily, M.M., McCalley, C.K., Logan, T.A., Crill, P.M., Saleska, S.R., Rich, V.I., Chanton, J.P. (2014) Changes in peat chemistry associated with permafrost thaw increase greenhouse gas production. Proceedings of the National Academy of Science 111, 5819–5824.
compared the same bog and fen sites in this study, drawing clear distinctions between the compositions of the organic matter. They highlight the ability of Sphagnum to control the rate of decomposition in the bog by producing phenolic compounds. This pattern is in stark contrast to the fen, where decomposition rates highly correspond to microbially derived organic matter (Hodgkins et al., 2016Hodgkins, S.B., Tfaily, M.M., Podgorski, D.C., McCalley, C.K., Saleska, S.R., Crill, P.M., Rich, V.I., Chanton, J.P., Cooper, W.T. (2016) Elemental composition and optical properties reveal changes in dissolved organic matter along a permafrost thaw chronosequence in a subarctic peatland. Geochimica et Cosmochimica Acta 187, 123–140.
).3. Methylation potential of peatland sub-habitats. The microbial communities are markedly different across the palsa, bog and fen as attested by 16S rRNA gene analyses (Mondav et al., 2014
Mondav, R., Woodcroft, B.J., Kim, E., McCalley, C.K., Hodgkins, S.B., Crill, P.M., Chanton, J., Hurst, G.B., VerBerkmoes, N.C., Saleska, S.R., Hugenholtz, P., Rich, V.I., Tyson, G.W. (2014) Discovery of a novel methanogen prevalent in thawing permafrost. Nature Communications 5, 3212.
; 2017Mondav, R., McCalley, C.K., Hodgkins, S.B., Frokling, S., Saleska, S.R., Rich, V.I., Chanton, J.P., Crill, P.M. (2017) Microbial network, phylogenetic diversity and community membership in the active layer across a permafrost thaw gradient. Environmental Microbiology 19, 3201–3218.
). We examined observed lineages for potential Hg methylators (i.e. microbial genera either experimentally demonstrated to methylate Hg or inferred to do so by the presence of the hgcA and hgcB genes required for Hg methylation (Parks et al., 2013Parks, J.M., Johs, A., Podar, M., Bridou, R., Hurt Jr., R.A., Smith, S.D., Tomanicek, S.J., Qian, Y., Brown, S.D., Brandt, C.C., Palumbo, A.V., Smith, J.C., Wall, J.D., Elias, D.A., Liang, L. (2013) The Genetic Basis for Bacterial Mercury Methylation. Science 339, 1332–1335.
; Table S-2; detailed methods in Supplementary Information). These potential Hg-methylating microbes increased across the thaw gradient from low relative abundance in the palsa and bog (~0.5 % and ~0.7 % of the community, respectively) to markedly higher abundance in the fen (~7.8 %) (Fig. 3 and Table S-2). The identified potential Hg-methylators include sulphate- and iron-reducing lineages from the Deltaproteobacteria and Clostridia, as well as methanogens of Methanomicrobia (Table S-2). Methanoregula, an important methanogen at the site (Mondav et al., 2017Mondav, R., McCalley, C.K., Hodgkins, S.B., Frokling, S., Saleska, S.R., Rich, V.I., Chanton, J.P., Crill, P.M. (2017) Microbial network, phylogenetic diversity and community membership in the active layer across a permafrost thaw gradient. Environmental Microbiology 19, 3201–3218.
) and one of the potential Hg-methylators present, was both relatively more abundant and more diverse (more lineages recovered) in the fen. Overall, the presence of a larger and more diverse community of potential Hg-methylators in the fen supports the role of microbes in driving elevated MeHg in fen porewaters and the presence of potential Hg-methylating methanogens highlights the interacting impacts of permafrost thaw on the production of both methane and MeHg.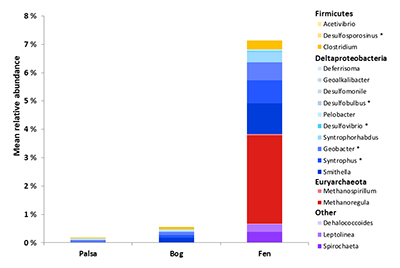
Figure 3 Mean relative abundance of potential Hg methylators at Stordalen Mire (n = 38 for palsa, 47 for bog, and 42 for fen), using microbial community data from 2010-2012 reanalysed from Mondav et al. (2017)
Mondav, R., McCalley, C.K., Hodgkins, S.B., Frokling, S., Saleska, S.R., Rich, V.I., Chanton, J.P., Crill, P.M. (2017) Microbial network, phylogenetic diversity and community membership in the active layer across a permafrost thaw gradient. Environmental Microbiology 19, 3201–3218.
. * indicates genera with members experimentally confirmed to methylate Hg.A revised framework for Hg cycling in Arctic ecosystems
Contrary to earlier conceptual models of Hg export from Arctic ecosystems (e.g., Klaminder et al., 2008
Klaminder, J., Yoo, K., Rydberg, J., Giesler, R. (2008) An explorative study of mercury export from a thawing palsa mire. Journal of Geophysical Research: Biogeosciences 113, G04034.
), we found that while Hg is liberated from peat during the intermediate thaw stage it is subsequently accumulated in a later thaw stage represented in the fen site. Recent studies from Alaska (Obrist et al., 2017Obrist, D., Agnan, Y., Jiskra, M., Olson, C.L., Colegrove, D.P., Hueber, J., Moore, C.W., Sonke, J.E., Helmig, D. (2017) Tundra uptake of atmospheric elemental mercury drives Arctic mercury pollution. Nature 547, 201–204.
; Douglas and Blum, 2019Douglas, T.A., Blum, J.D. (2019) Mercury isotopes reveal atmospheric gaseous mercury deposition directly to the Arctic coastal snowpack. Environmental Science & Technology Letters 6, 235–242.
) found TGM uptake by vegetation to be a significant pathway of Hg accumulation. Increasingly longer growing seasons associated with changing climate (Callaghan et al., 2010Callaghan, T.V., Bergholm, F., Christensen, T.R., Jonasson, C., Kokfelt, U., Johansson, M. (2010) A new climate era in the sub‐Arctic: Accelerating climate changes and multiple impacts. Geophysical Research Letters 37, L14705.
and references therein), coupled with the expansion of fen sites throughout the mire (Johansson et al., 2006aJohansson, T., Malmer, N., Crill, P.M., Friborg, T., Åkerman, J.H., Mastepanov, M., Christensen, T.R. (2006a) Decadal vegetation changes in a northern peatland, greenhouse gas fluxes and net radiative forcing. Global Change Biology 12, 2352–2369.
), suggest this mechanism is likely relevant in Stordalen Mire. A second potential fate, depending on the pace of climate change, may be catastrophic collapse of permafrost as observed in the western Canadian Arctic (St. Pierre et al. 2018St. Pierre, K.A., Zolkos, S., Shakil, S., Tank, S.E., St. Louis, V.L., Kokelj, S.V. (2018) Unprecedented Increases in Total and Methyl Mercury Concentrations Downstream of Retrogressive Thaw Slumps in the Western Canadian Arctic. Environmental Science & Technology 52, 14099–14109.
). This second pathway bypasses the peat or fen storage and flushes large quantities of both total and methyl Hg from the ecosystem (St. Pierre et al. 2018St. Pierre, K.A., Zolkos, S., Shakil, S., Tank, S.E., St. Louis, V.L., Kokelj, S.V. (2018) Unprecedented Increases in Total and Methyl Mercury Concentrations Downstream of Retrogressive Thaw Slumps in the Western Canadian Arctic. Environmental Science & Technology 52, 14099–14109.
).top
Conclusions
Examination of HgTot pools in thawing peat, combined with mechanisms of gaseous Hg flux and MeHg production, lead to a revised conceptual model of pathways of Hg loss and retention in thawing permafrost peatlands (Fig. 4). Taken together, these results suggest a more complex set of processes amongst permafrost thaw regions. During initial stages of permafrost thaw, when the active layer in the palsa deepens, export of gas-phase TGM is a primary pathway of Hg loss. As the thaw continues vegetation changes to a Sphagnum-dominated semi-thawed ecosystem, where Hg export into the atmosphere limits accumulation of Hg in peat and the peat bound HgTot pool is depleted. The final stage of thaw, characterised by fully thawed fens, results in a more biologically mobile Hg pool. This transition is facilitated via favourable redox conditions, more labile organic matter and a diverse microbial community capable of methylation, resulting in greater Hg retention in fen peat and also higher levels of porewater MeHg that may be exported to nearby lakes and streams. Given the prediction of loss of discontinuous zone permafrost by 2100 (Slater and Lawrence, 2013
Slater, A.G., Lawrence, D.M. (2013) Diagnosing present and future permafrost from climate models. Journal of Climate 26, 5608.
), it is imperative that long term studies coupling Hg cycling, microbial community composition and mechanisms driving gene expression of Hg methylation as well as varying environmental conditions are carried out to improve predictions of the fate of Hg stored in permafrost within these dynamically changing ecosystems.
Figure 4 Schematic of biogeochemical Hg cycling across permafrost thaw with emphasis on Hg pools and major pathways. Red arrows denote gaseous (Hg0) flux for each stage of the thaw sequence. Peat inventories are for total Hg for the top 40 cm of the mire surface (cf. legend for corresponding range).
top
Acknowledgements
We thank the Abisko Scientific Research Station and Dr. R. Geisler for infrastructure and logistical support. We also thank N. Rakos, C. Hemmingsson and L. Erickson for field sampling assistance, members of ISOGENIE 2013 sampling personnel, B. Verbek and R. Wilson of FSU for bulk density data and Dr. S. Frey and M. Knorr for sample preparation assistance. This work was funded by an UNH Earth Sciences Graduate Research Award, the Karen Von Damm Memorial Student Award, the Karen Von Damm Leadership Development Award (JGB), NSF1255888 (JGB) and associated technical support from UNH, the Iola Hubbard Climate Change Endowment (RKV, JGB), the Northern Ecosystems Research for Undergraduates (NSF1063037), the Macrosystems Biology (NSF EF#1241037 (RKV) and by the Genomic Science Program of the United States Department of Energy Office of Biological and Environmental Research, grants DE-SC0010580 and DE-SC0016440.
Editor: Karim Benzerara
top
References
Bäckstrand, K., Crill, P.M., Jackowicz-Korczyñski, M., Mastepanov, M., Christensen, T.R., Bastviken, D. (2010) Annual carbon gas budget for a subarctic peatland, Northern Sweden. Biogeosciences 7, 95–108.

Linking thawing permafrost with hydrologic change (Johansson et al., 2006b), vegetation shifts over a 30 year period (Malmer et al., 2005; Johansson et al., 2006a) and carbon dynamics in a mire (e.g., Öquist and Svensson, 2002; Bäckstrand et al., 2010) provide essential context to develop a predictive framework for Hg cycling in the future thawed permafrost.
View in article
Barkay, T., Kroer, N., Poulain, A.J. (2011) Some like it cold: microbial transformations of mercury in polar regions. Polar Research 30, 15469.

Increasing Arctic temperatures also contribute to significant changes in microbial communities and activity which can influence the production of methyl Hg (MeHg), a powerful neurotoxin (Barkay et al., 2011 and references therein; Yang et al., 2016).
View in article
Callaghan, T.V., Bergholm, F., Christensen, T.R., Jonasson, C., Kokfelt, U., Johansson, M. (2010) A new climate era in the sub‐Arctic: Accelerating climate changes and multiple impacts. Geophysical Research Letters 37, L14705.

Increasingly longer growing seasons associated with changing climate (Callaghan et al., 2010 and references therein), coupled with the expansion of fen sites throughout the mire (Johansson et al., 2006a), suggest this mechanism is likely relevant in Stordalen Mire.
View in article
Carpi, A., Lindberg, S.E. (1997) Sunlight-Mediated emission of elemental mercury from soil amended with municipal sewage sludge. Environmental Science & Technology 31, 2085–2091.

During three days of dynamic chamber flux measurements (in the peak of the growing season) all three sites exhibited a diel pattern in TGM fluxes, as shown in previous studies (Carpi and Lindberg, 1997; Poissant et al., 2004; chamber flux measurement approach described in Supplementary Information).
View in article
Douglas, T.A., Blum, J.D. (2019) Mercury isotopes reveal atmospheric gaseous mercury deposition directly to the Arctic coastal snowpack. Environmental Science & Technology Letters 6, 235–242.

Recent studies from Alaska (Obrist et al., 2017; Douglas and Blum, 2019) found TGM uptake by vegetation to be a significant pathway of Hg accumulation.
View in article
Fitzgerald, W.F., Engstrom, D.R., Lamborg, C.H., Tseng, C., Balcom, P.H., Hammerschmidt, C.R. (2005) Modern and historic atmospheric mercury fluxes in northern Alaska: Global sources and arctic depletion. Environmental Science & Technology 39, 557–568.

Anthropogenic Hg emissions have increased Hg deposition to the Arctic three-fold since the Industrial Revolution (Fitzgerald et al., 2005).
View in article
Grigal, D.F. (2003) Mercury sequestration in forests and peatlands: a review. Journal of Environmental Quality 32, 393–405.

Terrestrial ecosystems are generally net sinks for atmospheric Hg deposition (Selin, 2009), and Hg sequestration in soil is positively correlated with organic matter content (Grigal, 2003; Smith-Downey et al., 2010).
View in article
Gustin, M.S., Engle, M., Ericksen, J., Lyman, S., Stamenkovi, J., Xin, M. (2006) Mercury exchange between the atmosphere and low mercury containing substrates. Applied Geochemistry 21, 1913–1923.

The strong correlation between TGM flux and PAR in the bog site is consistent with earlier interpretations (Klaminder et al., 2008) of increased photoreduction reactions in water-saturated soils, resulting from permafrost thaw, leading to lower peat Hg concentrations (Gustin et al., 2006).
View in article
Hodgkins, S.B., Tfaily, M.M., McCalley, C.K., Logan, T.A., Crill, P.M., Saleska, S.R., Rich, V.I., Chanton, J.P. (2014) Changes in peat chemistry associated with permafrost thaw increase greenhouse gas production. Proceedings of the National Academy of Science 111, 5819–5824.

Changes in the organic carbon (OC) balance from thawing permafrost, coupled with changing redox conditions associated with increases in soil moisture result in the release of labile OC (Hodgkins et al., 2014) that likely drives Hg export (Mu et al., 2019).
View in article
Hodgkins et al. (2014) compared the same bog and fen sites in this study, drawing clear distinctions between the compositions of the organic matter.
View in article
Hodgkins, S.B., Tfaily, M.M., Podgorski, D.C., McCalley, C.K., Saleska, S.R., Crill, P.M., Rich, V.I., Chanton, J.P., Cooper, W.T. (2016) Elemental composition and optical properties reveal changes in dissolved organic matter along a permafrost thaw chronosequence in a subarctic peatland. Geochimica et Cosmochimica Acta 187, 123–140.

This pattern is in stark contrast to the fen, where decomposition rates highly correspond to microbially derived organic matter (Hodgkins et al., 2016).
View in article
Johansson, T., Malmer, N., Crill, P.M., Friborg, T., Åkerman, J.H., Mastepanov, M., Christensen, T.R. (2006a) Decadal vegetation changes in a northern peatland, greenhouse gas fluxes and net radiative forcing. Global Change Biology 12, 2352–2369.

Between 1970 and 2000, permafrost thaw and palsa collapse led to the expansion of Sphagnum (bog) and Eriophorum (fen) by 3 % and 54 %, respectively (Johansson et al., 2006a).
View in article
Linking thawing permafrost with hydrologic change (Johansson et al., 2006b), vegetation shifts over a 30 year period (Malmer et al., 2005; Johansson et al., 2006a) and carbon dynamics in a mire (e.g., Öquist and Svensson, 2002; Bäckstrand et al., 2010) provide essential context to develop a predictive framework for Hg cycling in the future thawed permafrost.
View in article
Increasingly longer growing seasons associated with changing climate (Callaghan et al., 2010 and references therein), coupled with the expansion of fen sites throughout the mire (Johansson et al., 2006a), suggest this mechanism is likely relevant in Stordalen Mire.
View in article
Johansson, M., Christensen, T.R., Åkerman, H.J., Callaghan, T.V. (2006b) What Determines the Current Presence or Absence of Permafrost in the Torneträsk Region, a Sub-arctic Landscape in Northern Sweden? AMBIO: A Journal of the Human Environment 35, 190–197.

Linking thawing permafrost with hydrologic change (Johansson et al., 2006b), vegetation shifts over a 30 year period (Malmer et al., 2005; Johansson et al., 2006a) and carbon dynamics in a mire (e.g., Öquist and Svensson, 2002; Bäckstrand et al., 2010) provide essential context to develop a predictive framework for Hg cycling in the future thawed permafrost.
View in article
Klaminder, J., Yoo, K., Rydberg, J., Giesler, R. (2008) An explorative study of mercury export from a thawing palsa mire. Journal of Geophysical Research: Biogeosciences 113, G04034.

The strong correlation between TGM flux and PAR in the bog site is consistent with earlier interpretations (Klaminder et al., 2008) of increased photoreduction reactions in water-saturated soils, resulting from permafrost thaw, leading to lower peat Hg concentrations (Gustin et al., 2006).
View in article
The bog TGM flux, together with lower HgTot concentrations deeper in the peat, suggest significant Hg loss (Klaminder et al., 2008), with the semi-wet or intermediate stage of the thaw resulting in less Hg retention in soils and vegetation (Fig. 2).
View in article
Contrary to earlier conceptual models of Hg export from Arctic ecosystems (e.g., Klaminder et al., 2008), we found that while Hg is liberated from peat during the intermediate thaw stage it is subsequently accumulated in a later thaw stage represented in the fen site.
View in article
Malmer, N., Johansson, T., Olsrud, M., Christensen, T.R. (2005) Vegetation, climatic changes and net carbon sequestration in a North-Scandinavian subarctic mire over 30 years. Global Change Biology 11, 1895–1909.

Linking thawing permafrost with hydrologic change (Johansson et al., 2006b), vegetation shifts over a 30 year period (Malmer et al., 2005; Johansson et al., 2006a) and carbon dynamics in a mire (e.g., Öquist and Svensson, 2002; Bäckstrand et al., 2010) provide essential context to develop a predictive framework for Hg cycling in the future thawed permafrost.
View in article
Mondav, R., Woodcroft, B.J., Kim, E., McCalley, C.K., Hodgkins, S.B., Crill, P.M., Chanton, J., Hurst, G.B., VerBerkmoes, N.C., Saleska, S.R., Hugenholtz, P., Rich, V.I., Tyson, G.W. (2014) Discovery of a novel methanogen prevalent in thawing permafrost. Nature Communications 5, 3212.

The microbial communities are markedly different across the palsa, bog and fen as attested by 16S rRNA gene analyses (Mondav et al., 2014; 2017).
View in article
Mondav, R., McCalley, C.K., Hodgkins, S.B., Frokling, S., Saleska, S.R., Rich, V.I., Chanton, J.P., Crill, P.M. (2017) Microbial network, phylogenetic diversity and community membership in the active layer across a permafrost thaw gradient. Environmental Microbiology 19, 3201–3218.

The microbial communities are markedly different across the palsa, bog and fen as attested by 16S rRNA gene analyses (Mondav et al., 2014; 2017).
View in article
Methanoregula, an important methanogen at the site (Mondav et al., 2017) and one of the potential Hg-methylators present, was both relatively more abundant and more diverse (more lineages recovered) in the fen.
View in article
Figure 3 Mean relative abundance of potential Hg methylators at Stordalen Mire (n = 38 for palsa, 47 for bog, and 42 for fen), using microbial community data from 2010-2012 reanalysed from Mondav et al. (2017).
View in article
Mu, C., Zhang, F., Chen, X., Ge, S., Mu, M., Jia, L., Wu, Q., Zhang, T. (2019) Carbon and mercury export from the Arctic rivers and response to permafrost degradation. Water Research 161, 54–60.

Changes in the organic carbon (OC) balance from thawing permafrost, coupled with changing redox conditions associated with increases in soil moisture result in the release of labile OC (Hodgkins et al., 2014) that likely drives Hg export (Mu et al., 2019).
View in article
Studies of northern peatlands find that Hg export accompanies permafrost thaw associated with climate change (e.g., Rydberg et al., 2010; St. Pierre et al. 2018; Mu et al., 2019).
View in article
Obrist, D., Agnan, Y., Jiskra, M., Olson, C.L., Colegrove, D.P., Hueber, J., Moore, C.W., Sonke, J.E., Helmig, D. (2017) Tundra uptake of atmospheric elemental mercury drives Arctic mercury pollution. Nature 547, 201–204.

Recent studies from Alaska (Obrist et al., 2017; Douglas and Blum, 2019) found TGM uptake by vegetation to be a significant pathway of Hg accumulation.
View in article
Öquist, M.G., Svensson, B.H. (2002) Vascular plants as regulators of methane emissions from a subarctic mire ecosystem. Journal of Geophysical Research 107, 4580.

Linking thawing permafrost with hydrologic change (Johansson et al., 2006b), vegetation shifts over a 30 year period (Malmer et al., 2005; Johansson et al., 2006a) and carbon dynamics in a mire (e.g., Öquist and Svensson, 2002; Bäckstrand et al., 2010) provide essential context to develop a predictive framework for Hg cycling in the future thawed permafrost.
View in article
Pannu, R., Siciliano, S.D., O'Driscoll, N.J. (2014) Quantifying the effects of soil temperature, moisture and sterilization on elemental mercury formation in boreal soils. Environmental Pollution 193, 138.

Some studies have found that biotic demethylation of Hg can be a significant source of evasion (Pannu et al., 2014 and references therein).
View in article
Parks, J.M., Johs, A., Podar, M., Bridou, R., Hurt Jr., R.A., Smith, S.D., Tomanicek, S.J., Qian, Y., Brown, S.D., Brandt, C.C., Palumbo, A.V., Smith, J.C., Wall, J.D., Elias, D.A., Liang, L. (2013) The Genetic Basis for Bacterial Mercury Methylation. Science 339, 1332–1335.

We examined observed lineages for potential Hg methylators (i.e. microbial genera either experimentally demonstrated to methylate Hg or inferred to do so by the presence of the hgcA and hgcB genes required for Hg methylation (Parks et al., 2013; Table S-2; detailed methods in Supplementary Information).
View in article
Poissant, L., Pilote, M., Xu, X., Zhang, H., Beauvais, C. (2004) Mercury Gas Exchanges over Selected Bare Soil and Flooded Sites in the Bay St. François Wetlands (Québec, Canada). Atmospheric Environment 38, 4205–4214.

During three days of dynamic chamber flux measurements (in the peak of the growing season) all three sites exhibited a diel pattern in TGM fluxes, as shown in previous studies (Carpi and Lindberg, 1997; Poissant et al., 2004; chamber flux measurement approach described in Supplementary Information).
View in article
Rydberg, J., Klaminder, J., Rosén, P., Bindler, R. (2010) Climate driven release of carbon and mercury from permafrost mires increases mercury loading to sub-arctic lakes. Science of The Total Environment 408, 4778–4783.

Studies of northern peatlands find that Hg export accompanies permafrost thaw associated with climate change (e.g., Rydberg et al., 2010; St. Pierre et al. 2018; Mu et al., 2019).
View in article
Schartup, A.T., Balcom, P.H., Mason, R.P. (2014) Sediment-porewater partitioning, total sulfur, and methylmercury production in estuaries. Environmental Science & Technology 48, 954–960.

In reducing environments, S in peat forms organo-sulphides, especially in the presence of hydrogen sulphide (Schartup et al., 2014) which enhances storage of Hg.
View in article
Schuster, P.F., Schaefer, K.M., Aiken, G.R., Antweiler, R.C., Dewild, J.F., Gryziec, J.D., Gusmeroli, A., Hugelius, G., Jafarov, E., Krabbenhoft, D.P., Liu, L., Herman-Mercer, N., Mu, C., Roth, D.A., Schaefer, T., Striegl, R.G., Wickland, K.P., Zhang, T. (2018) Permafrost Stores a Globally Significant Amount of Mercury. Geophysical Research Letters 45, 1463–1471.

Permafrost constitutes the largest long term reservoir of Hg, containing twice that of all other reservoirs combined (Schuster et al., 2018 and references therein).
View in article
Selin, N.E. (2009) Global biogeochemical cycling of mercury: a review. Annual Review of Environment and Resources 34, 43–63.

Terrestrial ecosystems are generally net sinks for atmospheric Hg deposition (Selin, 2009), and Hg sequestration in soil is positively correlated with organic matter content (Grigal, 2003; Smith-Downey et al., 2010).
View in article
Skyllberg, U., Qian J., Frech, W., Xia, K., Bleam, W.F. (2003) Distribution of mercury, methyl mercury and organic sulphur species in soil, soil solution and stream of a boreal forest catchment. Biogeochemistry 64, 53–76.

Reducing conditions favoured in fen environments also influence the bioavailability of Hg via binding to thiol functional groups (Skyllberg et al., 2003).
View in article
The presence of higher total S in the fen site may also enhance the bioavailability of ionic Hg leading to methylation (Skyllberg et al., 2003).
View in article
Slater, A.G., Lawrence, D.M. (2013) Diagnosing present and future permafrost from climate models. Journal of Climate 26, 5608.

Given the prediction of loss of discontinuous zone permafrost by 2100 (Slater and Lawrence, 2013), it is imperative that long term studies coupling Hg cycling, microbial community composition and mechanisms driving gene expression of Hg methylation as well as varying environmental conditions are carried out to improve predictions of the fate of Hg stored in permafrost within these dynamically changing ecosystems.
View in article
Smith-Downey, N.V., Sunderland, E.M., Jacob, D.J. (2010) Anthropogenic impacts on global storage and emissions of mercury from terrestrial soils: Insights from a new global model. Journal of Geophysical Research: Biogeosciences 115, G03008.

Terrestrial ecosystems are generally net sinks for atmospheric Hg deposition (Selin, 2009), and Hg sequestration in soil is positively correlated with organic matter content (Grigal, 2003; Smith-Downey et al., 2010).
View in article
St. Pierre, K.A., Zolkos, S., Shakil, S., Tank, S.E., St. Louis, V.L., Kokelj, S.V. (2018) Unprecedented Increases in Total and Methyl Mercury Concentrations Downstream of Retrogressive Thaw Slumps in the Western Canadian Arctic. Environmental Science & Technology 52, 14099–14109.

Studies of northern peatlands find that Hg export accompanies permafrost thaw associated with climate change (e.g., Rydberg et al., 2010; St. Pierre et al. 2018; Mu et al., 2019).
View in article
A second potential fate, depending on the pace of climate change, may be catastrophic collapse of permafrost as observed in the western Canadian Arctic (St. Pierre et al., 2018).
View in article
This second pathway bypasses the peat or fen storage and flushes large quantities of both total and methyl Hg from the ecosystem (St. Pierre et al., 2018).
View in article
Tjerngren, I., Karlsson, T., Bjorn, E., Skyllberg, U. (2012) Potential Hg methylation and MeHg demethylation rates related to the nutrient status of different boreal wetlands. Biogeochemistry 108, 335–350.

In addition to enhanced peat Hg accumulation in the fen, we also found elevated concentrations of MeHg in fen porewater that were similar to those reported in other wetland studies (e.g., Tjerngren et al., 2012).
View in article
Yang, Z., Fang, W., Lu, X., Sheng, G.P., Graham, D.E., Liang, L., Wullschleger, S.D., Gu, B. (2016) Warming increases methylmercury production in an Arctic soil. Environmental Pollution 214, 504–509.

Increasing Arctic temperatures also contribute to significant changes in microbial communities and activity which can influence the production of methyl Hg (MeHg), a powerful neurotoxin (Barkay et al., 2011 and references therein; Yang et al., 2016).
View in article
top
Supplementary Information
The Supplementary Information includes:
- 1. Study Site Description
- 2. Autochamber TGM Flux Measurements
- 3. Continuous TGM Measurements
- 4. Peat Porewater Sampling and Methyl Mercury Analysis
- 5. Peat Sampling, Analysis and Mercury Pools Calculations
- 6. Estimating Resident Mercury-methylating Microbes
- Figures S-1 to S-5
- Tables S-1 to S-4
- Supplementary Information References
Download the Supplementary Information (PDF).
Figures and Tables

Figure 1 (a) Relationships between TGM flux and ambient air temperature (°C) and (b) photosynthetically active radiation (PAR). Symbols denote palsa (brown squares), bog (green triangles) and fen (blue diamonds) and include least squares linear regressions and associated r2 and p values. Evasion of TGM interpreted for values greater than zero and deposition for values less than zero.
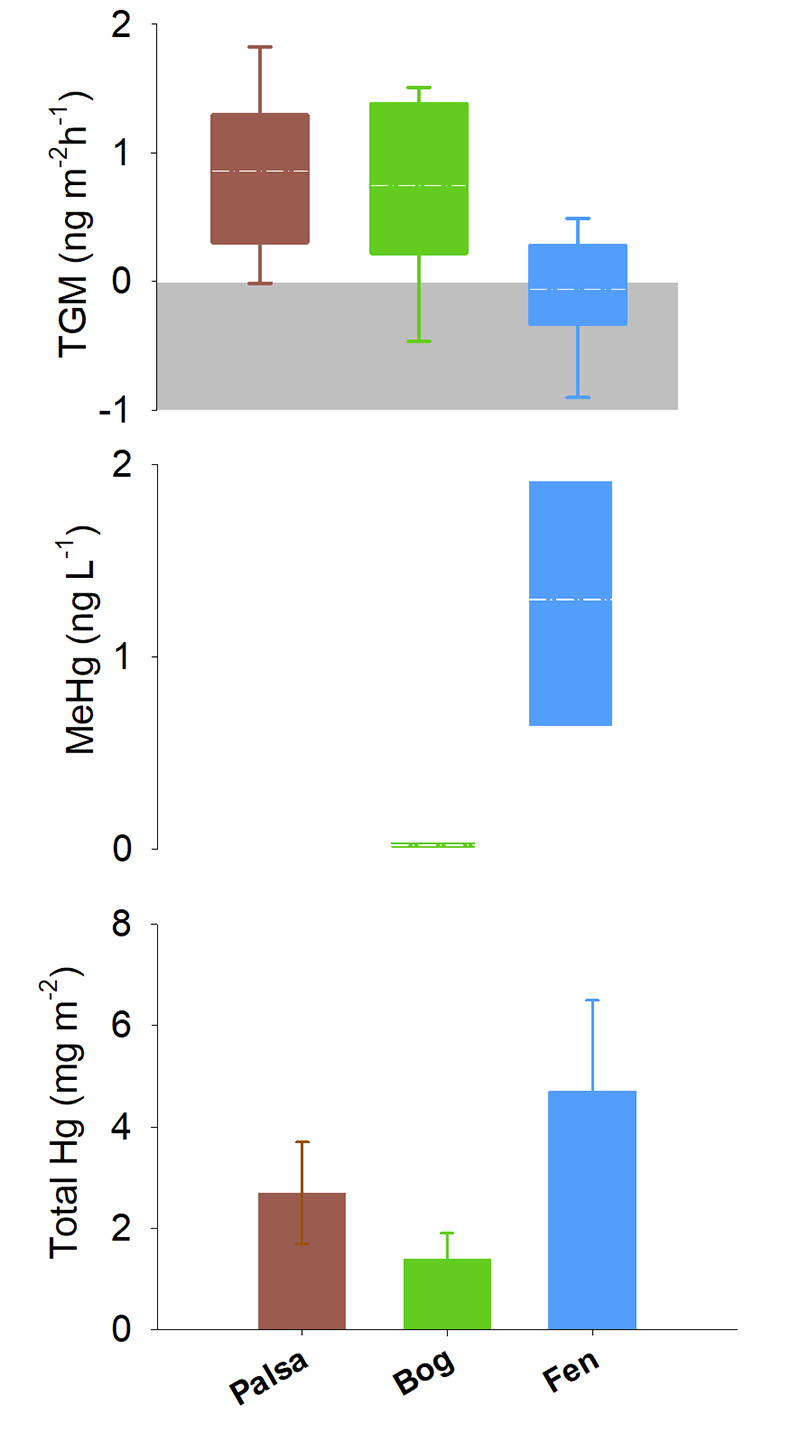
Figure 2 Summarised Hg in the three sub-habitats (from top to bottom): TGM, porewater methyl Hg (MeHg), and total peat Hg (corrected for dry bulk density).

Figure 3 Mean relative abundance of potential Hg methylators at Stordalen Mire (n = 38 for palsa, 47 for bog, and 42 for fen), using microbial community data from 2010-2012 reanalysed from Mondav et al. (2017)
Mondav, R., McCalley, C.K., Hodgkins, S.B., Frokling, S., Saleska, S.R., Rich, V.I., Chanton, J.P., Crill, P.M. (2017) Microbial network, phylogenetic diversity and community membership in the active layer across a permafrost thaw gradient. Environmental Microbiology 19, 3201–3218.
. * indicates genera with members experimentally confirmed to methylate Hg.
Figure 4 Schematic of biogeochemical Hg cycling across permafrost thaw with emphasis on Hg pools and major pathways. Red arrows denote gaseous (Hg0) flux for each stage of the thaw sequence. Peat inventories are for total Hg for the top 40 cm of the mire surface (cf. legend for corresponding range).