Mobility of chromium in high temperature crustal and upper mantle fluids
Affiliations | Corresponding Author | Cite as | Funding information- Share this article
Article views:1,969Cumulative count of HTML views and PDF downloads.
- Download Citation
- Rights & Permissions
top
Abstract

Figures and Tables
![]() Figure 1 (a, b) Effect of temperature and pressure on the valence state of ions at 25 °C and Psat (dashed lines), 200 °C and Psat (dotted dashed lines) and 600 °C and 2.0 GPa (solid lines). Horizontal lines represent the QFM buffer (black): (a) Fe3+/Fe2+ (orange) and Cr3+/Cr2+ (green) (b) Cu2+/Cu+ (blue) and Cr3+/Cr2+ (green). (c, d) Experimental Cr2O3 solubilities in HCl-rich fluids at 500–700 °C and 0.1–1.0 GPa. (c) HCl = 1.646 mol kg-1. (d) HCl = 2.249 mol kg-1. Solid curves are fitted to the experimental data at QFM + 1. | ![]() Figure 2 Experimental Cr2O3 solubilities in KCl fluids at 1000 °C and 4.0 GPa. Curves were fit to the highest two KCl points from QFM - 2 to QFM + 2. | ![]() Figure 3 Regression of log K for CrCl(OH)0 for dissociation reaction versus (a) pressure and (b) temperature up to 6.0 GPa and 1100 °C. | ![]() Figure 4 Prediction of Cr solubility in model fluids. (a) MOR, subduction zone, and diamond-forming fluids. (b) mafic eclogitic fluid at 570 °C and 2.0 GPa, Cr concentration versus Cl at QFM (red), QFM - 1 (green) and QFM - 2 (blue). Effects of Cl concentration and activity of Cr species on the solubility of Cr2O3(s) and Cr speciation at 600 °C and 2.0 GPa: (c) a(Cl-) = 0.5 (solid blue), 1 (dashed green) and 2 (dashed red) mol kg-1, assuming a(CrCl(OH)0) = a(Cr(OH–4) = 10-5 mol kg-1. (d) a(CrCl(OH)0) = a(Cr(OH–4) = 10-5 (solid blue), 10-4 (dashed green), 10-3 (dashed red) mol kg-1, when a(Cl-) = 0.5 mol kg-1. |
Figure 1 | Figure 2 | Figure 3 | Figure 4 |
top
Introduction
Chromium is a mobile element in ultramafic or mafic magmas but its mobility in high temperature fluids has long been unclear. Recent studies of chromium-rich geological settings have suggested that chromium could be mobile in deep crustal and upper mantle fluids. For example, fluid inclusions in chromite from New Caledonia (Johan et al., 2017
Johan, Z., Martin, R.F., Ettler, V. (2017) Fluids are bound to be involved in the formation of ophiolitic chromite deposits. European Journal of Mineralogy 29, 543–555.
) and in diopsidites and anorthosites associated with chromite and uvarovite from Oman (Akizawa et al., 2016Akizawa, N., Tamura, A., Fukushi, K., Yamamoto, J., Mizukami, T., Python, M., Arai, S. (2016) High-temperature hydrothermal activities around suboceanic Moho: An example from diopsidite and anorthosite in Wadi Fizh, Oman ophiolite. Lithos 263, 66–87.
) suggest the mobility of Cr in hydrothermal fluids at temperatures of about 500 to 800 °C. In addition, garnet inclusions in peridotitic diamonds are characteristically Cr-rich (Stachel and Harris, 2008Stachel, T., Harris, J.W. (2008) The origin of cratonic diamonds - Constraints from mineral inclusions. Ore Geology Reviews 34, 5–32.
), suggesting that Cr could be involved in the metasomatic processes of diamond formation under sub-solidus conditions. However, aqueous Cr3+ is highly insoluble in water at near neutral pH (Rai et al., 1987Rai, D., Sass, B.M., Moore, D.A. (1987) Chromium (III) hydrolysis constants and solubility of chromium (III) hydroxide. Inorganic Chemistry 26, 345–349.
; Saleh et al., 1989Saleh, F.Y., Parkerton, T.F., Lewis, R.V., Huang, J.H., Dickson, K.L. (1989) Kinetics of chromium transformations in the environment. Science of the Total Environment 86, 25-41.
; Ziemniak et al., 1998Ziemniak, S.E., Jones, M.E., Combs, K.E.S. (1998) Solubility and phase behavior of Cr(III) oxides in alkaline media at elevated temperatures. Journal of Solution Chemistry 27, 33–66.
; Sander and Koschinsky, 2000Sander, S., Koschinsky, A. (2000) Onboard-ship redox speciation of chromium in diffuse hydrothermal fluids from the North Fiji Basin. Marine Chemistry 71, 83–102.
), so a mechanism for Cr mobility has remained mysterious.One potential mechanism for Cr solubility at high temperatures is the likelihood of an aqueous Cr(II) oxidation state (Fig. 1a,b). Many metals exhibit the tendency for a lower oxidation state in aqueous solution at elevated temperatures, e.g., aqueous Cu+ vs. Cu2+ (Mei et al., 2013
Mei, Y., Sherman, D.M., Liu, W., Brugger, J. (2013) Ab initio molecular dynamics simulation and free energy exploration of copper(I) complexation by chloride and bisulfide in hydrothermal fluids. Geochimica et Cosmochimica Acta 102, 45–64.
), Fe2+ vs. Fe3+ (Testemale et al., 2009Testemale, D., Brugger, J., Liu, W., Etschmann, B., Hazemann, J.L. (2009) In-situ X-ray absorption study of Iron(II) speciation in brines up to supercritical conditions. Chemical Geology 264, 295–310.
), and Eu2+ vs. Eu3+ (Sverjensky, 1984Sverjensky, D.A. (1984) Europium redox equilibria in aqueous solution. Earth and Planetary Science Letters 67, 70–78.
). To investigate the potential mobility of Cr(II) and Cr(III) in deep fluids, we predicted the solubility of Cr oxide minerals by updating previous estimates of aqueous Cr species (Shock et al., 1997Shock, E.L., Sassani, D.C., Willis, M., Sverjensky, D.A. (1997) Inorganic species in geologic fluids: Correlations among standard molal thermodynamic properties of aqueous ions and hydroxide complexes. Geochimica et Cosmochimica Acta 61, 907–950.
; Hao, 2016Hao, J. (2016) Geochemical signatures of weathering and surface water chemistry in the late Archean. PhD thesis, Johns Hopkins University, 248 pp.
) in the Deep Earth Water (DEW) model (Sverjensky et al., 2014Sverjensky, D.A., Harrison, B., Azzolini, D. (2014). Water in the deep Earth: the dielectric constant and the solubilities of quartz and corundum to 60 kb and 1200 C. Geochimica et Cosmochimica Acta, 129, 125–145.
). We then regressed experimental solubility data for Cr2O3 to characterise a proposed new Cr(II) complex CrCl(OH)0 and obtain a predictive model for Cr solubility. Our model was applied to a wide range of geological settings: mid-ocean ridges (MOR), subduction zones and the subcontinental lithospheric mantle environment of diamond formation. Models and calculation methods are given in the Supplementary Information (SI).
Figure 1 (a, b) Effect of temperature and pressure on the valence state of ions at 25 °C and Psat (dashed lines), 200 °C and Psat (dotted dashed lines) and 600 °C and 2.0 GPa (solid lines). Horizontal lines represent the QFM buffer (black): (a) Fe3+/Fe2+ (orange) and Cr3+/Cr2+ (green) (b) Cu2+/Cu+ (blue) and Cr3+/Cr2+ (green). (c, d) Experimental Cr2O3 solubilities in HCl-rich fluids at 500–700 °C and 0.1–1.0 GPa. (c) HCl = 1.646 mol kg-1. (d) HCl = 2.249 mol kg-1. Solid curves are fitted to the experimental data at QFM + 1.
top
Results
Analysis of solubilities in solutions. We used published solubilities of Cr2O3 in HCl-rich fluids containing 1.646 mol kg-1 and 2.249 mol kg-1 HCl from 500–700 °C and 0.1–1.0 GPa (Watenphul et al., 2014
Watenphul, A., Schmidt, C., Jahn, S. (2014) Cr(III) solubility in aqueous fluids at high pressures and temperatures. Geochimica et Cosmochimica Acta 126, 212–227.
) to test the predicted properties of aqueous Cr species. The thermodynamic properties of eskolaite (Cr2O3) and picrochromite (MgCr2O4) were obtained from published experimental data (SI). The data points in Figure 1c,d represent the logarithms of measured Cr concentration vs. pressure. Cr concentrations at 400 °C were not considered in our analysis because the reactions may not have reached equilibrium (Watenphul et al., 2014Watenphul, A., Schmidt, C., Jahn, S. (2014) Cr(III) solubility in aqueous fluids at high pressures and temperatures. Geochimica et Cosmochimica Acta 126, 212–227.
). The horizontal error bar is the random error of pressure (±0.05 GPa). The vertical error bar is the standard error of the Cr concentration (0.01 mol kg-1) calculated from the minimum detection limit of Cr (Watenphul et al., 2014Watenphul, A., Schmidt, C., Jahn, S. (2014) Cr(III) solubility in aqueous fluids at high pressures and temperatures. Geochimica et Cosmochimica Acta 126, 212–227.
).Our preliminary solubility model considered the previously estimated properties of aqueous Cr(II), (III), and (VI) species (Table S-3; Shock et al., 1997
Shock, E.L., Sassani, D.C., Willis, M., Sverjensky, D.A. (1997) Inorganic species in geologic fluids: Correlations among standard molal thermodynamic properties of aqueous ions and hydroxide complexes. Geochimica et Cosmochimica Acta 61, 907–950.
; Hao, 2016Hao, J. (2016) Geochemical signatures of weathering and surface water chemistry in the late Archean. PhD thesis, Johns Hopkins University, 248 pp.
). This model resulted in predicted solubilities much lower than measured. For example, at 600 °C, 1.0 GPa and 2.249 mol kg-1 solution, the predicted Cr concentration was 87.5 mmol kg-1, about 2.6 times lower than the measured result at a similar pressure (228 mmol kg-1 at 0.917 GPa) (Table S-2b). Even larger discrepancies would be obtained in the KCl solutions at high pressures to be discussed below. The predominant oxidation state of the aqueous Cr was overwhelmingly +II at an oxidation state of QFM + 1 as expected by comparison with other metals. Despite the uncertainties in our overall approach, these results suggest that an additional Cr complex, probably of Cr(II), is needed to explain the high measured Cr solubilities.The apparent importance of aqueous Cr(II) species raises the concern that there was no measurement of the oxygen fugacities for the experiments shown in Figure 1c,d. However, Watenphul et al. (2014)
Watenphul, A., Schmidt, C., Jahn, S. (2014) Cr(III) solubility in aqueous fluids at high pressures and temperatures. Geochimica et Cosmochimica Acta 126, 212–227.
suggested that the experiments reflected oxidation states about 1.0 log unit above the QFM buffer. Under these conditions, we found that Cr(II) was the predominant valence. A second concern is the lack of experimental data for Cr speciation. However, based on ab initio simulations, Watenphul et al. (2014)Watenphul, A., Schmidt, C., Jahn, S. (2014) Cr(III) solubility in aqueous fluids at high pressures and temperatures. Geochimica et Cosmochimica Acta 126, 212–227.
suggested mixed Cr complexes with Cl, OH–, and/or H2O. After repeated trials of complexes such as CrCl+, Cr(OH)+ and CrCl(OH)02, we found a satisfactory fit to the experimental data using the mixed complex CrCl(OH)0. This complex may also have H2O coordinated to the Cr, but we cannot determine how many with the available experimental data. Therefore, we have omitted writing them. The curves in Figure 1c,d show our best fit to the experimental data at both low and high HCl concentrations. These calculations enabled us to retrieve the log K of the dissociation reaction of CrCl(OH)0 according toEq. 1
CrCl(OH)0 + H+ = Cr2+ + Cl– + H2O
The retrieved log K values have an uncertainty of ±0.3 units (Table S-2a). It should be noted that retrieval of the equilibrium constant for Equation 1 was carried out with simultaneous consideration of the other aqueous Cr species mentioned above as well as species such as HCl and OH– (SI). Because CrCl(OH)0 was by far the predominant Cr species in the solubility model, the overall solubility reaction studied experimentally can be approximated by the reaction
Eq. 2
Cr2O3 + 2Cl– + 2H+ = 2CrClOH0 + 0.5O2 (g)
which is a redox reaction that depends on the logƒO2 used in the calculations.
Analysis of Cr2O3 solubilities in KCl solutions. The only experimental data for Cr solubility at upper mantle conditions (Klein-BenDavid et al., 2011
Klein-BenDavid Ofra, O., Pettke, T., Kessel, R. (2011) Chromium mobility in hydrous fluids at upper mantle conditions. Lithos 125, 122–130.
) refer to the solubility of Cr2O3 at three KCl concentrations using the diamond trap method at 1000, 1200 °C and 4.0, 6.0 GPa. The solubilities varied with KCl concentration but did not vary significantly with temperature and pressure. Therefore, at each KCl concentration, we averaged the measurements at the different temperatures and pressures. We found that the complex CrCl(OH)0 could be fitted to the data at the two highest concentrations. The data points in Figure 2 represent the experimental Cr concentrations with a standard deviation as a vertical error bar. The scatter in the experimental data precludes fitting all three data points with a single complex. However, we do not have sufficient data to justify using more than one complex. We therefore calibrated our CrCl(OH)0 complex at the highest Cl concentration (Fig. 2). As a result, our predicted curve lies between the mid-KCl and low-KCl data points. The oxygen fugacity was again not controlled or measured in the experiments but was suggested to lie near to the QFM buffer. To test the sensitivity of our model calculations to the oxygen fugacity, we carried out calculations over a range of logƒO2 from QFM - 2 to QFM + 2 (Fig. 2). We subsequently used the equilibrium constant for the complex CrCl(OH)0 referring to QFM + 1 for comparison with the equilibrium constants retrieved from the solubility data in Figure 1c,d. The corresponding log K values have an uncertainty of ±0.3 units (SI).
Figure 2 Experimental Cr2O3 solubilities in KCl fluids at 1000 °C and 4.0 GPa. Curves were fit to the highest two KCl points from QFM - 2 to QFM + 2.
Equation of state for CrCl(OH)0. Assuming CrCl(OH)0 was the main Cr species in both the HCl and KCl experimental fluids, we combined the values of log K retrieved from the solubility of Cr2O3 in HCl and KCl fluids. For the HCl experiments, each log K value shown in Figure 3 represented a fit to the trend of experimental solubilities with pressure in Figure 1 (Table S-2a). The curves in Figure 3 represent regression using the DEW model. Estimated uncertainties are on the order of ±0.3 log units. It can be seen in Figure 3a that, as expected, the CrCl(OH)0 complex becomes stronger with increasing temperature and decreasing pressure, but the influence of pressure diminishes above about 2.0 GPa.
Although the curves agree within the estimated uncertainties with the experimentally calibrated log K values, it should be emphasised that the present characterisation is just a first approximation because no experimentally calibrated log K values are known below 500 °C. Nevertheless, the results serve as a valuable bridge between experiments involving Cr2O3 in chemically simple but different systems, i.e. HCl and KCl solutions, and enable extrapolation over a wide range of temperatures and pressures as shown in Figure 3b and Table S-4. We tested our model by applying it to the geochemically more complex systems of interest discussed below, some of which occur at temperatures and pressures well outside the range of calibration of the model.

Figure 3 Regression of log K for CrCl(OH)0 for dissociation reaction versus (a) pressure and (b) temperature up to 6.0 GPa and 1100 °C.
Predictions of Cr solubility in crustal hydrothermal and upper mantle fluids. In order to test the model, we used a range of different mineral assemblages (Table S-5) to constrain aqueous speciation and solubility model predictions of the fluid chemistry. The results (Table S-6) are not sensitive to the amounts of minerals but are sensitive to temperature, pressure and Cl concentration as discussed below. Figure 4a displays the predicted Cr solubility in five model fluids over a range of conditions: a crustal MOR fluid, pelitic, mafic, and ultramafic subduction zone fluids, and a peridotitic, diamond-forming fluid (Tomlinson et al., 2006
Tomlinson, E.L., Jones, A.P., Harris, J.W. (2006) Co-existing fluid and silicate inclusions in mantle diamond. Earth and Planetary Science Letters 250, 581–595.
; Huang, 2017Huang, F. (2017) Evolution of aqueous fluids, hydrocarbons, and diamond formation in the upper mantle. PhD thesis, Johns Hopkins University, 207 pp.
). The Cl concentrations are 0.5 mol kg-1, except in the peridotitic fluid where it is 8.0 mol kg-1 (Huang, 2017Huang, F. (2017) Evolution of aqueous fluids, hydrocarbons, and diamond formation in the upper mantle. PhD thesis, Johns Hopkins University, 207 pp.
). The ultramafic subduction zone fluid and the peridotitic fluid are in equilibrium with picrochromite, while the others are in equilibrium with eskolaite. Because these are pure minerals, the predicted solubilities are maxima. The predicted range of Cr solubilities is enormous: from 0.15 mg kg–1 H2O in the MOR fluid to 3500 mg kg–1 H2O in the peridotitic fluid. In all the models, CrCl(OH)0 is the major Cr species.top
Discussion
Crustal mid-ocean ridge (MOR) hydrothermal fluid. Prediction of the Cr content of MOR fluids is an important test of our model because the temperatures are much lower than in the experiments used to calibrate the model (Fig. 4a). At 350 °C and Psat (0.017 GPa), the MOR fluid in Figure 4a is predicted to contain only 0.1 mg kg-1 H2O of Cr. The low predicted value is consistent with observations by Sander and Koschinsky (2000)
Sander, S., Koschinsky, A. (2000) Onboard-ship redox speciation of chromium in diffuse hydrothermal fluids from the North Fiji Basin. Marine Chemistry 71, 83–102.
who suggested that Cr was about 1200 nM (0.062 mg kg-1) in their hydrothermal fluids. One difference from our prediction is that Sander and Koschinsky (2000)Sander, S., Koschinsky, A. (2000) Onboard-ship redox speciation of chromium in diffuse hydrothermal fluids from the North Fiji Basin. Marine Chemistry 71, 83–102.
further suggested that hydrothermal Cr was predominantly Cr(III). However, the Cr(III) concentration was not measured directly. Instead, it was obtained from the difference between the total Cr and the Cr(VI) concentrations at ambient temperatures and pressures where Cr(III) is expected to predominate.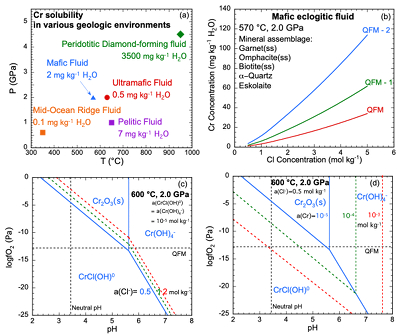
Figure 4 Prediction of Cr solubility in model fluids. (a) MOR, subduction zone, and diamond-forming fluids. (b) mafic eclogitic fluid at 570 °C and 2.0 GPa, Cr concentration versus Cl at QFM (red), QFM - 1 (green) and QFM - 2 (blue). Effects of Cl concentration and activity of Cr species on the solubility of Cr2O3(s) and Cr speciation at 600 °C and 2.0 GPa: (c) a(Cl-) = 0.5 (solid blue), 1 (dashed green) and 2 (dashed red) mol kg-1, assuming a(CrCl(OH)0) = a(Cr(OH–4)< = 10-5 mol kg-1. (d) a(CrCl(OH)0) = a(Cr(OH–4)) = 10-5 (solid blue), 10-4 (dashed green), 10-3 (dashed red) mol kg-1, when a(Cl-) = 0.5 mol kg-1.
Subduction zone fluids. In the subduction zone fluids, the predicted Cr concentrations are 0.5, 2, and 7 mg kg-1 H2O in ultramafic, mafic, and pelitic fluids, respectively. Although much higher than in the MOR fluid, Cr is still a trace element at these higher temperatures (570–650 °C) and pressures (1.0–2.0 GPa). The model ultramafic fluid has the lowest Cr because the fluid is at QFM + 2, buffered by magnetite, antigorite and chlorite (Debret and Sverjensky, 2017
Debret, B., Sverjensky, D.A. (2017) Highly oxidising fluids generated during serpentinite breakdown in subduction zones. Scientific Reports 7, 10351.
). The pelitic schist fluid has a higher Cr than the mafic eclogitic fluid, because the major Cr species CrCl(OH)0 favours high temperature and low pressure. The strongest influences on Cr solubility are oxygen fugacity and Cl concentration.Upper mantle peridotitic diamond-forming fluids. Cr in the peridotitic diamond-forming fluid is three orders of magnitude higher than in the other fluids. There are two reasons for this. First, the diamond-forming fluid contained 8.0 mol kg-1 Cl, much higher than in the other fluids. Second, the oxygen fugacity was low (QFM - 4). Although Cr was not detected in fluid inclusion studies of the Panda diamonds (Tomlinson et al., 2006
Tomlinson, E.L., Jones, A.P., Harris, J.W. (2006) Co-existing fluid and silicate inclusions in mantle diamond. Earth and Planetary Science Letters 250, 581–595.
), our prediction of 3500 mg kg–1 H2O of Cr is reasonable because it is below the detection limit of about 4000 mg kg–1 (Tomlinson, pers. comm.).A comparison can also be made with an experimental study of the solubility of a mafic eclogite in Cl-free fluids (Elazar et al., 2019
Elazar, O., Frost, D., Navon, O., Kessel, R. (2019) Melting of hydrous carbonated eclogite at 4-6 GPa and 900-1200°C: implications for the generation of diamond-forming fluids. Geochimica et Cosmochimica Acta 255, 69–87.
). Chromium concentrations measured at 4.0–5.0 GPa and 900–1200 °C were 17–96 mg kg–1 H2O. Our predicted Cr concentration is about 20 mg kg–1 H2O with only Cr-OH complexes at 950 °C and 4.5 GPa, which is consistent with the experiments.As already noted, Equation 2 shows that equilibrium between a Cr(III) mineral component and aqueous Cr(II) in a high temperature fluid is a redox reaction. Consequently, precipitation of a Cr(III) mineral component will also be a redox reaction. In the peridotitic, diamond-forming environment, garnet inclusions contain 3–20 wt. % Cr2O3 (Stachel and Harris, 2008
Stachel, T., Harris, J.W. (2008) The origin of cratonic diamonds - Constraints from mineral inclusions. Ore Geology Reviews 34, 5–32.
). A possible redox reaction that could account for such garnets might beEq. 3
→ 2Mg3Cr2Si3O12 + MgAl2O4 + C(diamond) + 4Cl– + 4H+ + H2O
Can Cr be more soluble in subduction zone fluids? To investigate further the factors influencing Cr solubility, Figure 4b presents the predicted Cr concentrations at 570 °C and 2.0 GPa in the mafic fluid as a function of Cl concentration and oxygen fugacity. At QFM and with Cl increasing from 0.5 mol kg-1 to 5.0 mol kg-1, the Cr solubility increases up to 34 mg kg–1 H2O, about a 30-fold increase. The increase at QFM - 2 with Cl is even more dramatic. These results suggest that mafic fluids could transport large concentrations of Cr at Cl-rich or reducing conditions. Such concentrations of Cr are consistent with a metasomatic origin for the occurrence of Cr-rich pyroxene in eclogitic veins in a metagabbro complex (Spandler et al., 2011
Spandler, C., Pettke, T., Rubatto, D. (2011) Internal and external fluid sources for eclogite-facies veins in the Monviso Meta-ophiolite, Western Alps: Implications for fluid flow in subduction zones. Journal of Petrology 52, 1207–1236.
).In the mafic eclogitic fluid, we tested the Cr solubility with no Cl and found a Cr solubility of 0.06 mg kg-1 H2O, much lower than the original prediction when Cl was 0.5 mol kg-1. The major Cr species is Cr(OH)-4. Therefore, we explored the effect of Cl concentration and activity of CrCl(OH)0 and Cr(OH)-4 on Cr2O3 dissolution, presented in Figure 4c,d as diagrams of logƒO2 versus pH. It can be seen that the stability field of CrCl(OH)0 expands with increasing Cl concentration and total Cr in the fluids. As mentioned above, elements tend to occur with lower valence state with increasing temperature. Figure 1a,b show that ions of Fe, Cu and Cr obey this trend. Although the stability field of Cr2+ is very small at 25 °C and Psat, at 600 °C and 2.0 GPa, Cr2+ is stable above QFM at most pH values.
top
Conclusions
The model presented here provides a framework for assessing the mobility of Cr in deep crustal and upper mantle fluids. Predicted chromium solubilities range widely. In MOR fluids Cr is almost insoluble. However, Cr can be highly soluble in reducing, Cl-bearing, subduction zone fluids. High predicted Cr concentrations in a peridotitic, diamond-forming fluid imply that Cr could be involved in the formation of diamond and Cr(III) garnet via a redox reaction. Chromium in the fluid could be oxidised and incorporated into garnet while C is reduced and precipitated as diamond. Such a redox reaction would explain the strong geologic linkage between diamonds and Cr-rich garnets.
top
Acknowledgements
Research was supported by the Johns Hopkins University, the Deep Carbon Observatory, the National Science Foundation, and the Department of Energy.
Editor: Horst R. Marschall
top
References
Akizawa, N., Tamura, A., Fukushi, K., Yamamoto, J., Mizukami, T., Python, M., Arai, S. (2016) High-temperature hydrothermal activities around suboceanic Moho: An example from diopsidite and anorthosite in Wadi Fizh, Oman ophiolite. Lithos 263, 66–87.

For example, fluid inclusions in chromite from New Caledonia (Johan et al., 2017) and in diopsidites and anorthosites associated with chromite and uvarovite from Oman (Akizawa et al., 2016) suggest the mobility of Cr in hydrothermal fluids at temperatures of about 500 to 800 °C.
View in article
Debret, B., Sverjensky, D.A. (2017) Highly oxidising fluids generated during serpentinite breakdown in subduction zones. Scientific Reports 7, 10351.

The model ultramafic fluid has the lowest Cr because the fluid is at QFM + 2, buffered by magnetite, antigorite and chlorite (Debret and Sverjensky, 2017).
View in article
Elazar, O., Frost, D., Navon, O., Kessel, R. (2019) Melting of hydrous carbonated eclogite at 4-6 GPa and 900-1200°C: implications for the generation of diamond-forming fluids. Geochimica et Cosmochimica Acta 255, 69–87.

A comparison can also be made with an experimental study of the solubility of a mafic eclogite in Cl-free fluids (Elazar et al., 2019).
View in article
Hao, J. (2016) Geochemical signatures of weathering and surface water chemistry in the late Archean. PhD thesis, Johns Hopkins University, 248 pp.

To investigate the potential mobility of Cr(II) and Cr(III) in deep fluids, we predicted the solubility of Cr oxide minerals by updating previous estimates of aqueous Cr species (Shock et al., 1997; Hao, 2016) in the Deep Earth Water (DEW) model (Sverjensky et al., 2014).
View in article
Our preliminary solubility model considered the previously estimated properties of aqueous Cr(II), (III), and (VI) species (Table S-3; Shock et al., 1997; Hao, 2016).
View in article
Huang, F. (2017) Evolution of aqueous fluids, hydrocarbons, and diamond formation in the upper mantle. PhD thesis, Johns Hopkins University, 207 pp.

Figure 4a displays the predicted Cr solubility in five model fluids over a range of conditions: a crustal MOR fluid, pelitic, mafic, and ultramafic subduction zone fluids, and a peridotitic, diamond-forming fluid (Tomlinson et al., 2006; Huang, 2017).
View in article
The Cl concentrations are 0.5 mol kg-1, except in the peridotitic fluid where it is 8.0 mol kg-1 (Huang, 2017).
View in article
Johan, Z., Martin, R.F., Ettler, V. (2017) Fluids are bound to be involved in the formation of ophiolitic chromite deposits. European Journal of Mineralogy 29, 543–555.

For example, fluid inclusions in chromite from New Caledonia (Johan et al., 2017) and in diopsidites and anorthosites associated with chromite and uvarovite from Oman (Akizawa et al., 2016) suggest the mobility of Cr in hydrothermal fluids at temperatures of about 500 to 800 °C.
View in article
Klein-BenDavid Ofra, O., Pettke, T., Kessel, R. (2011) Chromium mobility in hydrous fluids at upper mantle conditions. Lithos 125, 122–130.

The only experimental data for Cr solubility at upper mantle conditions (Klein-BenDavid et al., 2011) refer to the solubility of Cr2O3 at three KCl concentrations using the diamond trap method at 1000, 1200 °C and 4.0, 6.0 GPa.
View in article
Mei, Y., Sherman, D.M., Liu, W., Brugger, J. (2013) Ab initio molecular dynamics simulation and free energy exploration of copper(I) complexation by chloride and bisulfide in hydrothermal fluids. Geochimica et Cosmochimica Acta 102, 45–64.

Many metals exhibit the tendency for a lower oxidation state in aqueous solution at elevated temperatures, e.g., aqueous Cu+ vs. Cu2+ (Mei et al., 2013), Fe2+ vs. Fe3+ (Testemale et al., 2009), and Eu2+ vs. Eu3+ (Sverjensky, 1984).
View in article
Rai, D., Sass, B.M., Moore, D.A. (1987) Chromium (III) hydrolysis constants and solubility of chromium (III) hydroxide. Inorganic Chemistry 26, 345–349.

However, aqueous Cr3+ is highly insoluble in water at near neutral pH (Rai et al., 1987; Saleh et al., 1989; Ziemniak et al., 1998; Sander and Koschinsky, 2000), so a mechanism for Cr mobility has remained mysterious.
View in article
Saleh, F.Y., Parkerton, T.F., Lewis, R.V., Huang, J.H., Dickson, K.L. (1989) Kinetics of chromium transformations in the environment. Science of the Total Environment 86, 25-41.

However, aqueous Cr3+ is highly insoluble in water at near neutral pH (Rai et al., 1987; Saleh et al., 1989; Ziemniak et al., 1998; Sander and Koschinsky, 2000), so a mechanism for Cr mobility has remained mysterious.
View in article
Sander, S., Koschinsky, A. (2000) Onboard-ship redox speciation of chromium in diffuse hydrothermal fluids from the North Fiji Basin. Marine Chemistry 71, 83–102.

However, aqueous Cr3+ is highly insoluble in water at near neutral pH (Rai et al., 1987; Saleh et al., 1989; Ziemniak et al., 1998; Sander and Koschinsky, 2000), so a mechanism for Cr mobility has remained mysterious.
View in article
The low predicted value is consistent with observations by Sander and Koschinsky (2000) who suggested that Cr was about 1200 nM (0.062 mg kg-1) in their hydrothermal fluids.
View in article
One difference from our prediction is that Sander and Koschinsky (2000) further suggested that hydrothermal Cr was predominantly Cr(III).
View in article
Shock, E.L., Sassani, D.C., Willis, M., Sverjensky, D.A. (1997) Inorganic species in geologic fluids: Correlations among standard molal thermodynamic properties of aqueous ions and hydroxide complexes. Geochimica et Cosmochimica Acta 61, 907–950.

To investigate the potential mobility of Cr(II) and Cr(III) in deep fluids, we predicted the solubility of Cr oxide minerals by updating previous estimates of aqueous Cr species (Shock et al., 1997; Hao, 2016) in the Deep Earth Water (DEW) model (Sverjensky et al., 2014).
View in article
Our preliminary solubility model considered the previously estimated properties of aqueous Cr(II), (III), and (VI) species (Table S-3; Shock et al., 1997; Hao, 2016).
View in article
Spandler, C., Pettke, T., Rubatto, D. (2011) Internal and external fluid sources for eclogite-facies veins in the Monviso Meta-ophiolite, Western Alps: Implications for fluid flow in subduction zones. Journal of Petrology 52, 1207–1236.

Such concentrations of Cr are consistent with a metasomatic origin for the occurrence of Cr-rich pyroxene in eclogitic veins in a metagabbro complex (Spandler et al., 2011).
View in article
Stachel, T., Harris, J.W. (2008) The origin of cratonic diamonds - Constraints from mineral inclusions. Ore Geology Reviews 34, 5–32.

In addition, garnet inclusions in peridotitic diamonds are characteristically Cr-rich (Stachel and Harris, 2008), suggesting that Cr could be involved in the metasomatic processes of diamond formation under sub-solidus conditions.
View in article
In the peridotitic, diamond-forming environment, garnet inclusions contain 3–20 wt. % Cr2O3 (Stachel and Harris, 2008).
View in article
Sverjensky, D.A. (1984) Europium redox equilibria in aqueous solution. Earth and Planetary Science Letters 67, 70–78.

Many metals exhibit the tendency for a lower oxidation state in aqueous solution at elevated temperatures, e.g., aqueous Cu+ vs. Cu2+ (Mei et al., 2013), Fe2+ vs. Fe3+ (Testemale et al., 2009), and Eu2+ vs. Eu3+ (Sverjensky, 1984).
View in article
Sverjensky, D.A., Harrison, B., Azzolini, D. (2014). Water in the deep Earth: the dielectric constant and the solubilities of quartz and corundum to 60 kb and 1200 C. Geochimica et Cosmochimica Acta, 129, 125–145.

To investigate the potential mobility of Cr(II) and Cr(III) in deep fluids, we predicted the solubility of Cr oxide minerals by updating previous estimates of aqueous Cr species (Shock et al., 1997; Hao, 2016) in the Deep Earth Water (DEW) model (Sverjensky et al., 2014).
View in article
Testemale, D., Brugger, J., Liu, W., Etschmann, B., Hazemann, J.L. (2009) In-situ X-ray absorption study of Iron(II) speciation in brines up to supercritical conditions. Chemical Geology 264, 295–310.

Many metals exhibit the tendency for a lower oxidation state in aqueous solution at elevated temperatures, e.g., aqueous Cu+ vs. Cu2+ (Mei et al., 2013), Fe2+ vs. Fe3+ (Testemale et al., 2009), and Eu2+ vs. Eu3+ (Sverjensky, 1984).
View in article
Tomlinson, E.L., Jones, A.P., Harris, J.W. (2006) Co-existing fluid and silicate inclusions in mantle diamond. Earth and Planetary Science Letters 250, 581–595.

Figure 4a displays the predicted Cr solubility in five model fluids over a range of conditions: a crustal MOR fluid, pelitic, mafic, and ultramafic subduction zone fluids, and a peridotitic, diamond-forming fluid (Tomlinson et al., 2006; Huang, 2017).
View in article
Although Cr was not detected in fluid inclusion studies of the Panda diamonds (Tomlinson et al., 2006), our prediction of 3500 mg kg–1 H2O of Cr is reasonable because it is below the detection limit of about 4000 mg kg–1 (Tomlinson, pers. comm.).
View in article
Watenphul, A., Schmidt, C., Jahn, S. (2014) Cr(III) solubility in aqueous fluids at high pressures and temperatures. Geochimica et Cosmochimica Acta 126, 212–227.

We used published solubilities of Cr2O3 in HCl-rich fluids containing 1.646 mol kg-1 and 2.249 mol kg-1 HCl from 500–700 °C and 0.1–1.0 GPa (Watenphul et al., 2014) to test the predicted properties of aqueous Cr species.
View in article
Cr concentrations at 400 °C were not considered in our analysis because the reactions may not have reached equilibrium (Watenphul et al., 2014).
View in article
The vertical error bar is the standard error of the Cr concentration (0.01 mol kg-1) calculated from the minimum detection limit of Cr (Watenphul et al., 2014).
View in article
However, Watenphul et al. (2014) suggested that the experiments reflected oxidation states about 1.0 log unit above the QFM buffer.
View in article
However, based on ab initio simulations, Watenphul et al. (2014) suggested mixed Cr complexes with Cl, OH–, and/or H2O.
View in article
Ziemniak, S.E., Jones, M.E., Combs, K.E.S. (1998) Solubility and phase behavior of Cr(III) oxides in alkaline media at elevated temperatures. Journal of Solution Chemistry 27, 33–66.

However, aqueous Cr3+ is highly insoluble in water at near neutral pH (Rai et al., 1987; Saleh et al., 1989; Ziemniak et al., 1998; Sander and Koschinsky, 2000), so a mechanism for Cr mobility has remained mysterious.
View in article
top
Supplementary Information
The Supplementary Information includes:
- Theoretical Approaches
- Uncertainties in the Regression of the Experimental Data
- Fluid Models for Prediction of Cr Solutions
- Tables S-1 to S-6
- Figures S-1 and S-5
- Supplementary Information References
Download the Supplementary Information (PDF).
Figures and Tables

Figure 1 (a, b) Effect of temperature and pressure on the valence state of ions at 25 °C and Psat (dashed lines), 200 °C and Psat (dotted dashed lines) and 600 °C and 2.0 GPa (solid lines). Horizontal lines represent the QFM buffer (black): (a) Fe3+/Fe2+ (orange) and Cr3+/Cr2+ (green) (b) Cu2+/Cu+ (blue) and Cr3+/Cr2+ (green). (c, d) Experimental Cr2O3 solubilities in HCl-rich fluids at 500–700 °C and 0.1–1.0 GPa. (c) HCl = 1.646 mol kg-1. (d) HCl = 2.249 mol kg-1. Solid curves are fitted to the experimental data at QFM + 1.

Figure 2 Experimental Cr2O3 solubilities in KCl fluids at 1000 °C and 4.0 GPa. Curves were fit to the highest two KCl points from QFM - 2 to QFM + 2.

Figure 3 Regression of log K for CrCl(OH)0 for dissociation reaction versus (a) pressure and (b) temperature up to 6.0 GPa and 1100 °C.

Figure 4 Prediction of Cr solubility in model fluids. (a) MOR, subduction zone, and diamond-forming fluids. (b) mafic eclogitic fluid at 570 °C and 2.0 GPa, Cr concentration versus Cl at QFM (red), QFM - 1 (green) and QFM - 2 (blue). Effects of Cl concentration and activity of Cr species on the solubility of Cr2O3(s) and Cr speciation at 600 °C and 2.0 GPa: (c) a(Cl-) = 0.5 (solid blue), 1 (dashed green) and 2 (dashed red) mol kg-1, assuming a(CrCl(OH)0) = a(Cr(OH–4)< = 10-5 mol kg-1. (d) a(CrCl(OH)0) = a(Cr(OH–4)) = 10-5 (solid blue), 10-4 (dashed green), 10-3 (dashed red) mol kg-1, when a(Cl-) = 0.5 mol kg-1.