In situ determination of sulfur speciation and partitioning in aqueous fluid-silicate melt systems
Affiliations | Corresponding Author | Cite as | Funding information- Share this article
Article views:163Cumulative count of HTML views and PDF downloads.
- Download Citation
- Rights & Permissions
top
Abstract
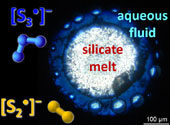




Figures
![]() Figure 1 In situ approach applied in this study to determine sulfur speciation and partitioning in fluid-magma systems. A chip of silicate glass and aqueous thiosulfate solution are loaded in a diamond anvil cell (left), which is then heated to 700 °C and 0.3–1.5 GPa yielding a silicate melt and a blue aqueous fluid containing significant amounts of trisulfur radical ion (right). The coexisting phases are directly probed by micro-Raman spectroscopy and quantification based on Raman band intensities was performed using a method developed in this study (Supplementary Information). | ![]() Figure 2 Raman spectra from representative experiments with indicated fluid and melt compositions, acquired at 700 °C and ∼1 GPa with 473 and 532 nm excitation, and the major identified species. | ![]() Figure 3 Partition coefficients (defined as the ratio of the species concentration in the fluid over its concentration in the silicate melt) at 700 °C and 0.3–1.5 GPa between fluid and melt for each sulfur species ( | ![]() Figure 4 (Upper panel) In situ fluid/melt partition coefficients of sulfate, sulfide, |
Figure 1 | Figure 2 | Figure 3 | Figure 4 |
top
Introduction
The solubility, partitioning, and chemical speciation of sulfur in aqueous fluid-silicate melt systems are controlling factors in the processes of volatile and metal transfer and ore deposit formation in geodynamic settings associated with subduction zones. Our knowledge of these factors is currently based on ex situ analyses of fluids and melts brought to the Earth’s surface or recovered in laboratory experiments upon cooling, such as volcanic sublimates and gases, fluid inclusions or silicate glasses. Yet, the complex and highly variable chemical and redox states of sulfur, spanning from S2− (sulfide) to S6+ (sulfate), raise questions of how stable the different sulfur species are at temperatures (T) and pressures (P) relevant to magma generation and evolution, and how representative quenched natural and experimental samples are with regard to sulfur speciation and partitioning across the lithosphere. Indeed, along with coexisting sulfate and sulfide commonly observed in quenched samples, other previously disregarded species such as the tri- and disulfur radical ions,


Pokrovski, G.S., Dubrovinsky, L.S. (2011) The S3– ion is stable in geological fluids at elevated temperatures and pressures. Science 331, 1052–1054.
; Schmidt and Seward, 2017Schmidt, C., Seward, T.M. (2017) Raman spectroscopic quantification of sulfur species in aqueous fluids: Ratios of relative molar scattering factors of Raman bands of H2S, HS−, SO2, HSO4−, SO42−, S2O32−, S3− and H2O at ambient conditions and information on changes with pressure and temperature. Chemical Geology 467, 64–75.
). On the other hand, these radicals can bind to gold in hydrothermal fluids thereby enhancing the efficiency of gold supply to ore deposits (Pokrovski et al., 2015Pokrovski, G.S., Kokh, M.A., Guillaume, D., Borisova, A.Y., Gisquet, P., Hazemann, J.-L., Lahera, E., Del Net, W., Proux, O., Testemale, D., Haigis, V., Jonchière, R., Seitsonen, A.P., Ferlat, G., Vuilleumier, R., Saitta, A.M., Boiron, M.-C., Dubessy, J. (2015) Sulfur radical species form gold deposits on Earth. Proceeding of the National Academy of Sciences of the U.S.A. 112, 13484–13489.
; 2019Pokrovski, G.S., Kokh, M.A., Proux, O., Hazemann, J.-L., Bazarkina, E.F., Testemale, D., Escoda, C., Boiron, M.-C., Blanchard, M., Ajgouy, T., Gouy, S., de Parseval, P., Thibaut, M. (2019) The nature and partitioning of invisible gold in the pyrite-fluid system. Ore Geology Reviews 109, 545–563.
). At shallow crust conditions (<0.3 GPa, <10 km depth), porphyry Cu-Mo-Re-Au and associated epithermal and skarn deposits, which are among the most significant resources of those metals on Earth, form in the vicinity of degassing magma bodies emplaced in the upper continental crust of arcs and back arc settings (Hedenquist and Lowenstern, 1994Hedenquist, J.W., Lowenstern, J.B. (1994) The role of magmas in the formation of hydrothermal ore deposits. Nature 370, 519–527.
; Fontboté et al., 2017Fontboté, L., Kouzmanov, K., Chiaradia, M., Pokrovski, G.S. (2017) Sulfide minerals in hydrothermal deposits. Elements 13, 97–103.
). Although available natural silicate glass analyses, experimental data, and resulting models for sulfur in hydrous silicate melts provide a robust understanding of sulfur redox and partitioning at such conditions (e.g., Moretti and Baker, 2008Moretti, R., Baker, D.R. (2008) Modeling the interplay of fO2 and fS2 along the FeS-silicate melt equilibrium. Chemical Geology 256, 286–298.
; Klimm and Botcharnikov, 2010Klimm, K., Botcharnikov, R.E. (2010) The determination of sulfate and sulfide species in hydrous silicate glasses using Raman spectroscopy. American Mineralogist 95, 1574–1579.
; Wallace and Edmonds, 2011Wallace, P.J., Edmonds, M. (2011) The sulfur budget in magmas: evidence from melt inclusions, submarine glasses and volcanic emissions. Reviews in Mineralogy and Geochemistry 73, 215–246.
; Zajacz et al., 2012Zajacz, Z., Candela, P.A., Piccoli, P.M., Wälle, M., Sanchez-Valle, C. (2012) Gold and copper in volatile saturated mafic to intermediate magmas: Solubilities, partitioning, and implications for ore deposit formation. Geochimica et Cosmochimica Acta 91, 140–159.
; Zajacz, 2015Zajacz, Z. (2015) The effect of melt composition on the partitioning of oxidized sulfur between silicate melts and magmatic volatiles. Geochimica et Cosmochimica Acta 158, 223–244.
; Binder et al., 2018Binder, B., Wenzel, T., Keppler, H. (2018) The partitioning of sulfur between multicomponent aqueous fluids and felsic melts. Contribution to Mineralogy and Petrology 173, 18.
), much less is known about S redox and distribution in fluid-melt systems from lower crustal and upper mantle settings relevant to magma generation in subduction zones from which water, sulfur and metals for these deposits are sourced. At such depths (30–100 km, ∼1–3 GPa), dehydration of the subducting slab generates aqueous fluids that can cause partial melting of the overlying mantle wedge (Hedenquist and Lowenstern, 1994Hedenquist, J.W., Lowenstern, J.B. (1994) The role of magmas in the formation of hydrothermal ore deposits. Nature 370, 519–527.
; Richards, 2003Richards, J.P. (2003) Tectono-magmatic precursors for porphyry Cu-(Mo-Au) deposit formation. Economic Geology 98, 1515–1533.
). Such melts that become fluid saturated at some depth may carry and release significant amounts of sulfur-bound metals such as Au, Cu and Mo, whose fate strongly depends on the redox state and partitioning of sulfur itself between fluid and melt, during magma storage, evolution and transport across the subduction zone (e.g., Richards, 2003Richards, J.P. (2003) Tectono-magmatic precursors for porphyry Cu-(Mo-Au) deposit formation. Economic Geology 98, 1515–1533.
; Audétat and Simon, 2012Audétat, A., Simon, A.C. (2012) Magmatic controls on porphyry copper genesis. Society of Economic Geologists Special Publication 16, 553–572.
; Chelle-Michou et al., 2017Chelle-Michou, C., Rottier, B., Caricchi, L., Simpson, G. (2017) Tempo of magma degassing and the genesis of porphyry copper deposits. Scientific Reports 7, 40566.
).In an attempt to probe directly the sulfur chemical speciation and partitioning at the magmatic-hydrothermal transition, and to assess the impact of non-quenchable sulfur species such as


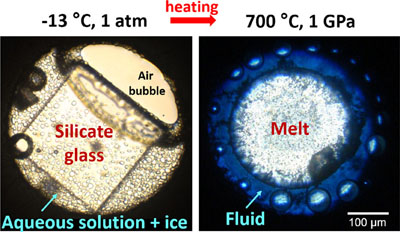
Figure 1 In situ approach applied in this study to determine sulfur speciation and partitioning in fluid-magma systems. A chip of silicate glass and aqueous thiosulfate solution are loaded in a diamond anvil cell (left), which is then heated to 700 °C and 0.3–1.5 GPa yielding a silicate melt and a blue aqueous fluid containing significant amounts of trisulfur radical ion (right). The coexisting phases are directly probed by micro-Raman spectroscopy and quantification based on Raman band intensities was performed using a method developed in this study (Supplementary Information).
top
Sulfur Speciation in the Coexisting Fluid and Melt Phases
Raman spectroscopy is the method of choice to study different sulfur species in fluids and melts owing to their characteristic and intense Raman scattering bands (Schmidt and Seward, 2017
Schmidt, C., Seward, T.M. (2017) Raman spectroscopic quantification of sulfur species in aqueous fluids: Ratios of relative molar scattering factors of Raman bands of H2S, HS−, SO2, HSO4−, SO42−, S2O32−, S3− and H2O at ambient conditions and information on changes with pressure and temperature. Chemical Geology 467, 64–75.
). The fluid phase at high T-P in all DAC experiments (Table S-2) shows Raman bands of water, dissolved silica species, and sulfate and sulfide (Fig. 2). In experiments with both sulfate and sulfide, the fluid displays a blue colour under white light illumination (Fig. 1), demonstrating the presence of the blue chromophore
Chivers, T., Elder, P.J.W. (2013) Ubiquitous trisulfur radical ion: fundamentals and applications in materials science, electrochemistry, analytical chemistry and geochemistry. Chemical Society Reviews 42, 5996–6005.
). The Raman spectrum of the fluid phase indeed shows the characteristic vibrations of





Eq. 1

Eq. 2

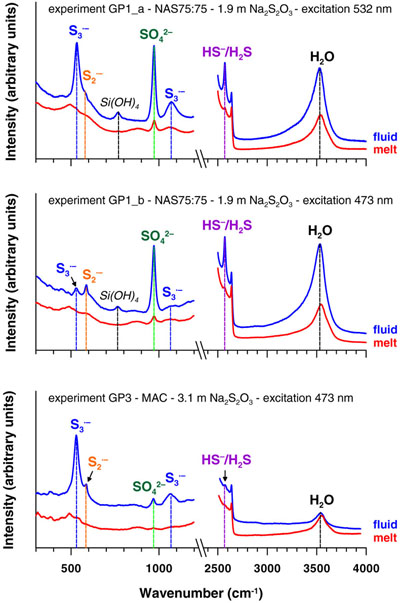
Figure 2 Raman spectra from representative experiments with indicated fluid and melt compositions, acquired at 700 °C and ∼1 GPa with 473 and 532 nm excitation, and the major identified species.
The O–H stretching band of water in the spectra of silicate melts differs both in shape and position from that in spectra of the coexisting aqueous fluid. The melt spectra also show low intensity Si–O and Si–O–Si bands, similar to those in quenched glasses but distinct from those in the aqueous fluid (Figs. 2, S-3), thus supporting a negligible contribution of the fluid to the melt spectra. The species H2S (and HS−) and




top
In Situ Fluid/Melt Partition Coefficients of Sulfur Species
Our in situ data allow generation of the first direct set of fluid/melt partition coefficients, Dfl/mt(i), of each individual S species (where i = sulfate, sulfide,



Figure 3 Partition coefficients (defined as the ratio of the species concentration in the fluid over its concentration in the silicate melt) at 700 °C and 0.3–1.5 GPa between fluid and melt for each sulfur species (


top
Sulfur and Metal Transfer from Magmas to Ore Deposits in Subduction Zones
Our new findings provide insight into sulfur behaviour in subduction zone settings where fluid saturated melt generation, transfer and degassing can occur across a wide range of T-P and depth.
Firstly, our novel in situ data offer the advantage of direct estimation of sulfide and sulfate Dfl/mt values for a given melt composition across a large fO2 range, because fluid/melt partitioning of an individual sulfur species is independent of fO2. Our extrapolated values for melts of rhyolitic composition equilibrated with aqueous fluids at 0.3–1.5 GPa (Dfl/mt(sulfate) ∼10 and Dfl/mt(sulfide) <10; Figs. 4, S-5) are a factor of 10 lower than those inferred from ex situ experiments performed at pressures of <0.5 GPa (see Supplementary Information) and analyses of natural glass inclusions trapped in volcanic rocks (e.g., Wallace and Edmonds, 2011
Wallace, P.J., Edmonds, M. (2011) The sulfur budget in magmas: evidence from melt inclusions, submarine glasses and volcanic emissions. Reviews in Mineralogy and Geochemistry 73, 215–246.
). Our results imply that felsic magmas, generated at depth and degassing through the lithosphere (10–45 km) both at highly reducing and highly oxidising conditions (i.e. outside the fO2 range within which the radical ions may be abundant), may retain more sulfur than was expected from ex situ analyses.
Figure 4 (Upper panel) In situ fluid/melt partition coefficients of sulfate, sulfide,


Moretti, R., Baker, D.R. (2008) Modeling the interplay of fO2 and fS2 along the FeS-silicate melt equilibrium. Chemical Geology 256, 286–298.
); horizontal dashed line shows partition coefficients of sulfide extrapolated to the more reduced conditions of our in situ measurements. (Lower panel) Cartoon of a subduction zone setting (not to scale) schematically illustrating silicate melt and aqueous fluid generation and evolution, and the associated sulfur redox changes.Secondly, our results demonstrate that both



Pokrovski, G.S., Dubessy, J. (2015) Stability and abundance of the trisulfur radical ion in hydrothermal fluids. Earth and Planetary Science Letters 411, 298–309.
). The fO2 range favourable for both radicals matches that of the mantle wedge above the subducting slab where partial melting occurs. At these depths, back and forth changes in fO2 across the sulfate-sulfide transition window are common (Fig. 4). For instance, sulfate-dominated fluids, derived from subducted oceanic crust at >100 km depth, have the ability to oxidise the sulfide-bearing mantle wedge (e.g., Evans, 2012Evans, K.A. (2012) The redox budget of subduction zones. Earth Science Reviews 113, 11–32.
; Walters et al., 2020Walters, J.B., Cruz-Uribe, A.M., Marschall, H.R. (2020) Sulfur loss from subducted altered crust and implications for mantle oxidation. Geochemical Perspectives Letters 13, 36–41.
), by producing partial melts that rise up further to the mantle-crust boundary (70–40 km depth) and experience multiple phenomena (e.g., Richards, 2003Richards, J.P. (2003) Tectono-magmatic precursors for porphyry Cu-(Mo-Au) deposit formation. Economic Geology 98, 1515–1533.
; Evans, 2012Evans, K.A. (2012) The redox budget of subduction zones. Earth Science Reviews 113, 11–32.
) as outlined in Figure 4. At higher crustal levels (5–15 km depth), mixing between sulfate-bearing felsic and FeS-bearing mafic melts may lead to S-rich magmas and fluids that are the major source of Au, Cu and Mo in porphyry deposits (Audétat and Simon, 2012Audétat, A., Simon, A.C. (2012) Magmatic controls on porphyry copper genesis. Society of Economic Geologists Special Publication 16, 553–572.
). At shallower depths (<5 km), conduit flow modelling shows that degassing magmas rising to the surface in arc settings may evolve from reduced to oxidised, or oxidised to reduced, depending on their S content and initial fO2 (Burgisser and Scaillet, 2007Burgisser, A., Scaillet, B. (2007) Redox evolution of a degassing magma rising to the surface. Nature 445, 194–197.
). In summary, at different stages of their evolution, arc magmas are likely to cross the sulfide-sulfate transition favouring formation of



Thirdly, the strong enrichment of the fluid in radical ions compared to melt, coupled with the high affinity of


Pokrovski, G.S., Kokh, M.A., Proux, O., Hazemann, J.-L., Bazarkina, E.F., Testemale, D., Escoda, C., Boiron, M.-C., Blanchard, M., Ajgouy, T., Gouy, S., de Parseval, P., Thibaut, M. (2019) The nature and partitioning of invisible gold in the pyrite-fluid system. Ore Geology Reviews 109, 545–563.
), and potentially for critical metals that have strong chemical bonds with sulfur (Pt, Pd, Mo, Re; e.g., Laskar et al., 2019Laskar, C., Pokrovski, G.S., Kokh, M.A., Hazemann, J.-L., Bazarkina, E.F., Desmaele, E. (2019) The impact of sulfur on the transfer of platinoids by geological fluids. Abstracts of the Goldschmidt 2019 Conference, Barcelona, Spain, 1831 〈hal-02343794〉.
), may be a key factor controlling trace metal transfer from the melt to the S-bearing fluid. Gold solubility in silicate melts displays a maximum at S6+/S2− ratios between 0.1 and 0.9 (Botcharnikov et al., 2011Botcharnikov, R., Linnen, R.L., Wilke, M., Holtz, F., Jugo, P.J., Berndt, J. (2011) High gold concentrations in sulphide-bearing magma under oxidizing conditions. Nature Geoscience 4, 112–115.
; Zajacz et al., 2012Zajacz, Z., Candela, P.A., Piccoli, P.M., Wälle, M., Sanchez-Valle, C. (2012) Gold and copper in volatile saturated mafic to intermediate magmas: Solubilities, partitioning, and implications for ore deposit formation. Geochimica et Cosmochimica Acta 91, 140–159.
), implying enhanced gold extraction from the mantle by magmas generated by partial melting of the mantle wedge at such redox conditions. Upon ascent and degassing of such Au pre-enriched magma, this gold is remobilised and concentrated by the released
Fontboté, L., Kouzmanov, K., Chiaradia, M., Pokrovski, G.S. (2017) Sulfide minerals in hydrothermal deposits. Elements 13, 97–103.
). According to the most conservative estimates of the Dfl/mt(



top
Acknowledgements
This work was funded by the French National Research Agency (grants RadicalS, ANR-16-CE31-0017), the Institut Carnot ISIFoR (grant OrPet), and the French and German embassies (grant PHC Procope SulFluMag). We thank T. Aigouy, P. Besson, J. Buhk, A. Castillo, P. Gisquet, S. Gouy, M. Kokh, J.-F. Ména, F. de Parseval, P. de Parseval, and S. Moyano for their help with sample preparation and analyses, A.-M. Cousin for her help with figure layout, W.A. Bassett for advice on DAC techniques, G. Harlow, J. Newman and B. Ledé for providing lazurite samples, and K. Kiseeva for discussions. Constructive comments of Editor H. Marschall and reviewers Z. Zajacz and C. LaFlamme greatly improved this article. We are grateful to A. Williams and M.-A. Hulshoff for editorial management.
Editor: Horst R. Marschall
top
References
Audétat, A., Simon, A.C. (2012) Magmatic controls on porphyry copper genesis. Society of Economic Geologists Special Publication 16, 553–572.

Such melts that become fluid saturated at some depth may carry and release significant amounts of sulfur-bound metals such as Au, Cu and Mo, whose fate strongly depends on the redox state and partitioning of sulfur itself between fluid and melt, during magma storage, evolution and transport across the subduction zone (e.g., Richards, 2003; Audétat and Simon, 2012; Chelle-Michou et al., 2017).
View in article
At higher crustal levels (5–15 km depth), mixing between sulfate-bearing felsic and FeS-bearing mafic melts may lead to S-rich magmas and fluids that are the major source of Au, Cu and Mo in porphyry deposits (Audétat and Simon, 2012).
View in article
Binder, B., Wenzel, T., Keppler, H. (2018) The partitioning of sulfur between multicomponent aqueous fluids and felsic melts. Contribution to Mineralogy and Petrology 173, 18.

Although available natural silicate glass analyses, experimental data, and resulting models for sulfur in hydrous silicate melts provide a robust understanding of sulfur redox and partitioning at such conditions (e.g., Moretti and Baker, 2008; Klimm and Botcharnikov, 2010; Wallace and Edmonds, 2011; Zajacz et al., 2012; Zajacz, 2015; Binder et al., 2018), much less is known about S redox and distribution in fluid-melt systems from lower crustal and upper mantle settings relevant to magma generation in subduction zones from which water, sulfur and metals for these deposits are sourced.
View in article
Botcharnikov, R., Linnen, R.L., Wilke, M., Holtz, F., Jugo, P.J., Berndt, J. (2011) High gold concentrations in sulphide-bearing magma under oxidizing conditions. Nature Geoscience 4, 112–115.

Thirdly, the strong enrichment of the fluid in radical ions compared to melt, coupled with the high affinity of and
for gold (e.g., Pokrovski et al., 2019), and potentially for critical metals that have strong chemical bonds with sulfur (Pt, Pd, Mo, Re; e.g., Laskar et al., 2019), may be a key factor controlling trace metal transfer from the melt to the S-bearing fluid. Gold solubility in silicate melts displays a maximum at S6+/S2− ratios between 0.1 and 0.9 (Botcharnikov et al., 2011; Zajacz et al., 2012), implying enhanced gold extraction from the mantle by magmas generated by partial melting of the mantle wedge at such redox conditions.
View in article
Burgisser, A., Scaillet, B. (2007) Redox evolution of a degassing magma rising to the surface. Nature 445, 194–197.

At shallower depths (<5 km), conduit flow modelling shows that degassing magmas rising to the surface in arc settings may evolve from reduced to oxidised, or oxidised to reduced, depending on their S content and initial fO2 (Burgisser and Scaillet, 2007).
View in article
Chelle-Michou, C., Rottier, B., Caricchi, L., Simpson, G. (2017) Tempo of magma degassing and the genesis of porphyry copper deposits. Scientific Reports 7, 40566.

Such melts that become fluid saturated at some depth may carry and release significant amounts of sulfur-bound metals such as Au, Cu and Mo, whose fate strongly depends on the redox state and partitioning of sulfur itself between fluid and melt, during magma storage, evolution and transport across the subduction zone (e.g., Richards, 2003; Audétat and Simon, 2012; Chelle-Michou et al., 2017).
View in article
Chivers, T., Elder, P.J.W. (2013) Ubiquitous trisulfur radical ion: fundamentals and applications in materials science, electrochemistry, analytical chemistry and geochemistry. Chemical Society Reviews 42, 5996–6005.

In experiments with both sulfate and sulfide, the fluid displays a blue colour under white light illumination (Fig. 1), demonstrating the presence of the blue chromophore ion (Chivers and Elders, 2013).
View in article
Evans, K.A. (2012) The redox budget of subduction zones. Earth Science Reviews 113, 11–32.

For instance, sulfate-dominated fluids, derived from subducted oceanic crust at >100 km depth, have the ability to oxidise the sulfide-bearing mantle wedge (e.g., Evans, 2012; Walters et al., 2020), by producing partial melts that rise up further to the mantle-crust boundary (70–40 km depth) and experience multiple phenomena (e.g., Richards, 2003; Evans, 2012) as outlined in Figure 4.
View in article
Fontboté, L., Kouzmanov, K., Chiaradia, M., Pokrovski, G.S. (2017) Sulfide minerals in hydrothermal deposits. Elements 13, 97–103.

At shallow crust conditions (<0.3 GPa, <10 km depth), porphyry Cu-Mo-Re-Au and associated epithermal and skarn deposits, which are among the most significant resources of those metals on Earth, form in the vicinity of degassing magma bodies emplaced in the upper continental crust of arcs and back arc settings (Hedenquist and Lowenstern, 1994; Fontboté et al., 2017).
View in article
Such sulfide-dominated, yet moderately oxidised, systems are common in arc magma settings that host major porphyry-epithermal Cu-Mo-Au deposits (Fontboté et al., 2017).
View in article
Hedenquist, J.W., Lowenstern, J.B. (1994) The role of magmas in the formation of hydrothermal ore deposits. Nature 370, 519–527.

At shallow crust conditions (<0.3 GPa, <10 km depth), porphyry Cu-Mo-Re-Au and associated epithermal and skarn deposits, which are among the most significant resources of those metals on Earth, form in the vicinity of degassing magma bodies emplaced in the upper continental crust of arcs and back arc settings (Hedenquist and Lowenstern, 1994; Fontboté et al., 2017).
View in article
At such depths (30–100 km, ∼1–3 GPa), dehydration of the subducting slab generates aqueous fluids that can cause partial melting of the overlying mantle wedge (Hedenquist and Lowenstern, 1994; Richards, 2003).
View in article
Klimm, K., Botcharnikov, R.E. (2010) The determination of sulfate and sulfide species in hydrous silicate glasses using Raman spectroscopy. American Mineralogist 95, 1574–1579.

Although available natural silicate glass analyses, experimental data, and resulting models for sulfur in hydrous silicate melts provide a robust understanding of sulfur redox and partitioning at such conditions (e.g., Moretti and Baker, 2008; Klimm and Botcharnikov, 2010; Wallace and Edmonds, 2011; Zajacz et al., 2012; Zajacz, 2015; Binder et al., 2018), much less is known about S redox and distribution in fluid-melt systems from lower crustal and upper mantle settings relevant to magma generation in subduction zones from which water, sulfur and metals for these deposits are sourced.
View in article
Laskar, C., Pokrovski, G.S., Kokh, M.A., Hazemann, J.-L., Bazarkina, E.F., Desmaele, E. (2019) The impact of sulfur on the transfer of platinoids by geological fluids. Abstracts of the Goldschmidt 2019 Conference, Barcelona, Spain, 1831 〈hal-02343794〉.

Thirdly, the strong enrichment of the fluid in radical ions compared to melt, coupled with the high affinity of and
for gold (e.g., Pokrovski et al., 2019), and potentially for critical metals that have strong chemical bonds with sulfur (Pt, Pd, Mo, Re; e.g., Laskar et al., 2019), may be a key factor controlling trace metal transfer from the melt to the S-bearing fluid. Gold solubility in silicate melts displays a maximum at S6+/S2− ratios between 0.1 and 0.9 (Botcharnikov et al., 2011; Zajacz et al., 2012), implying enhanced gold extraction from the mantle by magmas generated by partial melting of the mantle wedge at such redox conditions.
View in article
Moretti, R., Baker, D.R. (2008) Modeling the interplay of fO2 and fS2 along the FeS-silicate melt equilibrium. Chemical Geology 256, 286–298.

Although available natural silicate glass analyses, experimental data, and resulting models for sulfur in hydrous silicate melts provide a robust understanding of sulfur redox and partitioning at such conditions (e.g., Moretti and Baker, 2008; Klimm and Botcharnikov, 2010; Wallace and Edmonds, 2011; Zajacz et al., 2012; Zajacz, 2015; Binder et al., 2018), much less is known about S redox and distribution in fluid-melt systems from lower crustal and upper mantle settings relevant to magma generation in subduction zones from which water, sulfur and metals for these deposits are sourced.
View in article
Blue area outlines the sulfate-sulfide boundary in water saturated melt (Moretti and Baker, 2008); horizontal dashed line shows partition coefficients of sulfide extrapolated to the more reduced conditions of our in situ measurements.
View in article
Pokrovski, G.S., Dubrovinsky, L.S. (2011) The S3– ion is stable in geological fluids at elevated temperatures and pressures. Science 331, 1052–1054.

Indeed, along with coexisting sulfate and sulfide commonly observed in quenched samples, other previously disregarded species such as the tri- and disulfur radical ions, and
, become increasingly abundant in aqueous fluids above 200 °C but cannot be preserved upon cooling being unstable at ambient conditions (Pokrovski and Dubrovinsky, 2011; Schmidt and Seward, 2017).
View in article
Pokrovski, G.S., Dubessy, J. (2015) Stability and abundance of the trisulfur radical ion in hydrothermal fluids. Earth and Planetary Science Letters 411, 298–309.

First order estimates of the fraction in the experimental fluids of this study (700 °C, 1 GPa, 1–4 molal Na2S2O3), amounting from 5 to 50 % of Stot, are in good agreement with that predicted (Fig. S-8) using recent thermodynamic models based on in situ data at ≤500 °C (Pokrovski and Dubessy, 2015).
View in article
Pokrovski, G.S., Kokh, M.A., Guillaume, D., Borisova, A.Y., Gisquet, P., Hazemann, J.-L., Lahera, E., Del Net, W., Proux, O., Testemale, D., Haigis, V., Jonchière, R., Seitsonen, A.P., Ferlat, G., Vuilleumier, R., Saitta, A.M., Boiron, M.-C., Dubessy, J. (2015) Sulfur radical species form gold deposits on Earth. Proceeding of the National Academy of Sciences of the U.S.A. 112, 13484–13489.

On the other hand, these radicals can bind to gold in hydrothermal fluids thereby enhancing the efficiency of gold supply to ore deposits (Pokrovski et al., 2015; 2019).
View in article
Pokrovski, G.S., Kokh, M.A., Proux, O., Hazemann, J.-L., Bazarkina, E.F., Testemale, D., Escoda, C., Boiron, M.-C., Blanchard, M., Ajgouy, T., Gouy, S., de Parseval, P., Thibaut, M. (2019) The nature and partitioning of invisible gold in the pyrite-fluid system. Ore Geology Reviews 109, 545–563.

On the other hand, these radicals can bind to gold in hydrothermal fluids thereby enhancing the efficiency of gold supply to ore deposits (Pokrovski et al., 2015; 2019).
View in article
Thirdly, the strong enrichment of the fluid in radical ions compared to melt, coupled with the high affinity of and
for gold (e.g., Pokrovski et al., 2019), and potentially for critical metals that have strong chemical bonds with sulfur (Pt, Pd, Mo, Re; e.g., Laskar et al., 2019), may be a key factor controlling trace metal transfer from the melt to the S-bearing fluid. Gold solubility in silicate melts displays a maximum at S6+/S2− ratios between 0.1 and 0.9 (Botcharnikov et al., 2011; Zajacz et al., 2012), implying enhanced gold extraction from the mantle by magmas generated by partial melting of the mantle wedge at such redox conditions.
View in article
Richards, J.P. (2003) Tectono-magmatic precursors for porphyry Cu-(Mo-Au) deposit formation. Economic Geology 98, 1515–1533.

At such depths (30–100 km, ∼1–3 GPa), dehydration of the subducting slab generates aqueous fluids that can cause partial melting of the overlying mantle wedge (Hedenquist and Lowenstern, 1994; Richards, 2003).
View in article
Such melts that become fluid saturated at some depth may carry and release significant amounts of sulfur-bound metals such as Au, Cu and Mo, whose fate strongly depends on the redox state and partitioning of sulfur itself between fluid and melt, during magma storage, evolution and transport across the subduction zone (e.g., Richards, 2003; Audétat and Simon, 2012; Chelle-Michou et al., 2017).
View in article
For instance, sulfate-dominated fluids, derived from subducted oceanic crust at >100 km depth, have the ability to oxidise the sulfide-bearing mantle wedge (e.g., Evans, 2012; Walters et al., 2020), by producing partial melts that rise up further to the mantle-crust boundary (70–40 km depth) and experience multiple phenomena (e.g., Richards, 2003; Evans, 2012) as outlined in Figure 4.
View in article
Schmidt, C., Seward, T.M. (2017) Raman spectroscopic quantification of sulfur species in aqueous fluids: Ratios of relative molar scattering factors of Raman bands of H2S, HS−, SO2, HSO4−, SO42−, S2O32−, S3− and H2O at ambient conditions and information on changes with pressure and temperature. Chemical Geology 467, 64–75.

Indeed, along with coexisting sulfate and sulfide commonly observed in quenched samples, other previously disregarded species such as the tri- and disulfur radical ions, and
, become increasingly abundant in aqueous fluids above 200 °C but cannot be preserved upon cooling being unstable at ambient conditions (Pokrovski and Dubrovinsky, 2011; Schmidt and Seward, 2017).
View in article
Raman spectroscopy is the method of choice to study different sulfur species in fluids and melts owing to their characteristic and intense Raman scattering bands (Schmidt and Seward, 2017).
View in article
Wallace, P.J., Edmonds, M. (2011) The sulfur budget in magmas: evidence from melt inclusions, submarine glasses and volcanic emissions. Reviews in Mineralogy and Geochemistry 73, 215–246.

Although available natural silicate glass analyses, experimental data, and resulting models for sulfur in hydrous silicate melts provide a robust understanding of sulfur redox and partitioning at such conditions (e.g., Moretti and Baker, 2008; Klimm and Botcharnikov, 2010; Wallace and Edmonds, 2011; Zajacz et al., 2012; Zajacz, 2015; Binder et al., 2018), much less is known about S redox and distribution in fluid-melt systems from lower crustal and upper mantle settings relevant to magma generation in subduction zones from which water, sulfur and metals for these deposits are sourced.
View in article
Our extrapolated values for melts of rhyolitic composition equilibrated with aqueous fluids at 0.3–1.5 GPa (Dfl/mt(sulfate) ∼10 and Dfl/mt(sulfide) <10; Figs. 4, S-5) are a factor of 10 lower than those inferred from ex situ experiments performed at pressures of <0.5 GPa (see Supplementary Information) and analyses of natural glass inclusions trapped in volcanic rocks (e.g., Wallace and Edmonds, 2011).
View in article
Walters, J.B., Cruz-Uribe, A.M., Marschall, H.R. (2020) Sulfur loss from subducted altered crust and implications for mantle oxidation. Geochemical Perspectives Letters 13, 36–41.

For instance, sulfate-dominated fluids, derived from subducted oceanic crust at >100 km depth, have the ability to oxidise the sulfide-bearing mantle wedge (e.g., Evans, 2012; Walters et al., 2020), by producing partial melts that rise up further to the mantle-crust boundary (70–40 km depth) and experience multiple phenomena (e.g., Richards, 2003; Evans, 2012) as outlined in Figure 4.
View in article
Zajacz, Z. (2015) The effect of melt composition on the partitioning of oxidized sulfur between silicate melts and magmatic volatiles. Geochimica et Cosmochimica Acta 158, 223–244.

Although available natural silicate glass analyses, experimental data, and resulting models for sulfur in hydrous silicate melts provide a robust understanding of sulfur redox and partitioning at such conditions (e.g., Moretti and Baker, 2008; Klimm and Botcharnikov, 2010; Wallace and Edmonds, 2011; Zajacz et al., 2012; Zajacz, 2015; Binder et al., 2018), much less is known about S redox and distribution in fluid-melt systems from lower crustal and upper mantle settings relevant to magma generation in subduction zones from which water, sulfur and metals for these deposits are sourced.
View in article
Zajacz, Z., Candela, P.A., Piccoli, P.M., Wälle, M., Sanchez-Valle, C. (2012) Gold and copper in volatile saturated mafic to intermediate magmas: Solubilities, partitioning, and implications for ore deposit formation. Geochimica et Cosmochimica Acta 91, 140–159.

Although available natural silicate glass analyses, experimental data, and resulting models for sulfur in hydrous silicate melts provide a robust understanding of sulfur redox and partitioning at such conditions (e.g., Moretti and Baker, 2008; Klimm and Botcharnikov, 2010; Wallace and Edmonds, 2011; Zajacz et al., 2012; Zajacz, 2015; Binder et al., 2018), much less is known about S redox and distribution in fluid-melt systems from lower crustal and upper mantle settings relevant to magma generation in subduction zones from which water, sulfur and metals for these deposits are sourced.
View in article
Thirdly, the strong enrichment of the fluid in radical ions compared to melt, coupled with the high affinity of and
for gold (e.g., Pokrovski et al., 2019), and potentially for critical metals that have strong chemical bonds with sulfur (Pt, Pd, Mo, Re; e.g., Laskar et al., 2019), may be a key factor controlling trace metal transfer from the melt to the S-bearing fluid. Gold solubility in silicate melts displays a maximum at S6+/S2− ratios between 0.1 and 0.9 (Botcharnikov et al., 2011; Zajacz et al., 2012), implying enhanced gold extraction from the mantle by magmas generated by partial melting of the mantle wedge at such redox conditions.
View in article
top
Supplementary Information
The Supplementary Information includes:
- Materials and Methods
- Supplementary Text: Additional Notes
- Tables S-1 to S-8
- Figures S-1 to S-8
- Supplementary Information References
Download the Supplementary Information (PDF).
Figures

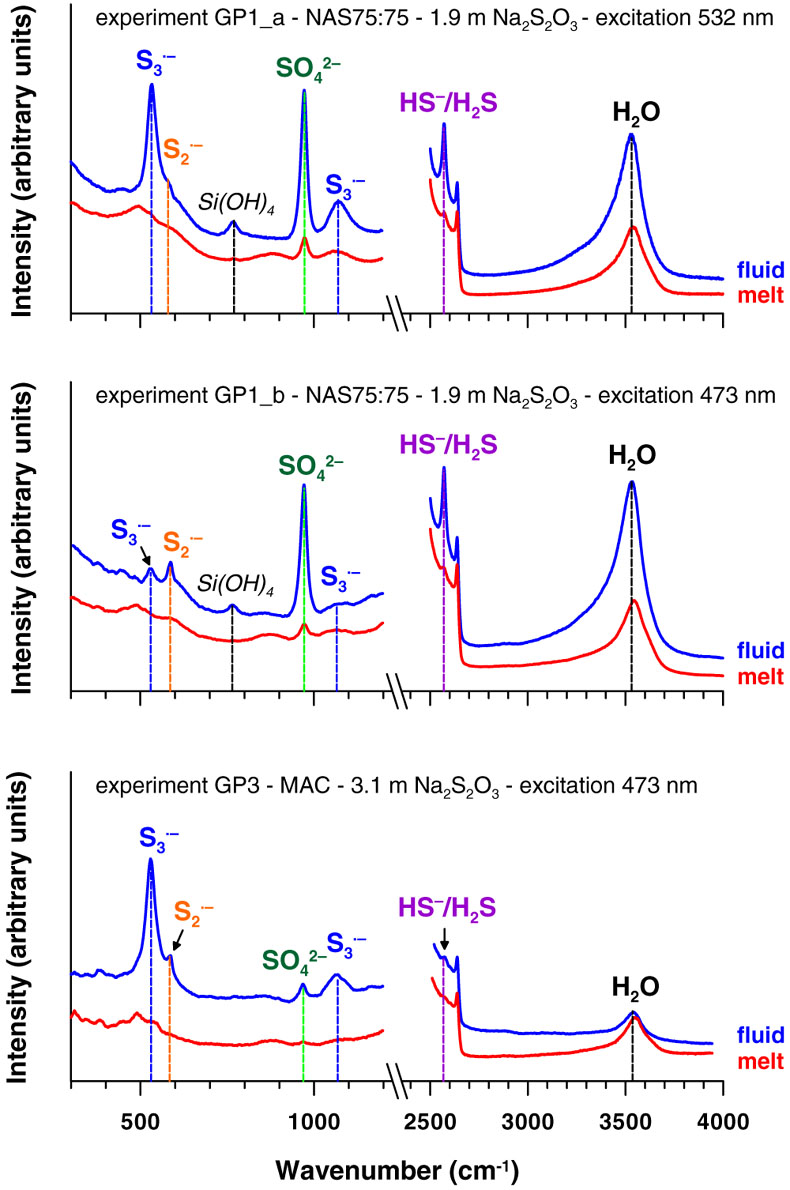






Moretti, R., Baker, D.R. (2008) Modeling the interplay of fO2 and fS2 along the FeS-silicate melt equilibrium. Chemical Geology 256, 286–298.
); horizontal dashed line shows partition coefficients of sulfide extrapolated to the more reduced conditions of our in situ measurements. (Lower panel) Cartoon of a subduction zone setting (not to scale) schematically illustrating silicate melt and aqueous fluid generation and evolution, and the associated sulfur redox changes.