The vanadium isotope composition of Mars: implications for planetary differentiation in the early solar system
Affiliations | Corresponding Author | Cite as | Funding information- Share this article
-
Article views:360Cumulative count of HTML views and PDF downloads.
- Download Citation
- Rights & Permissions
top
Abstract
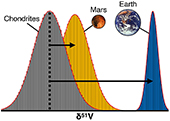
Figures and Tables
![]() Table 1 Vanadium isotope data for martian meteorites. | ![]() Figure 1 Martian meteorite vanadium isotopic data corrected for GCR effects plotted against V/Ce ratios. Error bars are 2 s.d. as listed in Table 1. Given that V and Ce have different partition coefficients during fractional crystallisation and melting, variations in V/Ce likely reflect a combination of these processes. The lack of systematic V isotope variation over the entire range of V/Ce implies that magmatic processes did not induce detectable V isotope variation on Mars. Ce concentrations from Yoshizaki and McDonough (2020); V concentrations from Table 1. Note log scale on y axis. | ![]() Figure 2 Vanadium isotope data for different sample types from the Solar System. Data for terrestrial samples are from Prytulak et al. (2013), Wu et al. (2018) and Qi et al. (2019); chondrite data are from Nielsen et al., (2019) and this study (Table S-2). All data have been corrected for spallation-produced 50V. Error bars for each sample group are 2 s.e. weighted by the individual sample error bars shown in Table 1. Shaded areas denote the average for those objects and error weighted 2 s.e. of the entire data population. Chondrite averages have been weighted by individual measurement errors. | ![]() Figure 3 Modelled V isotope fractionation factors between the core and mantle of Earth (blue) and Mars (red) as a function of the fraction of total V that entered the core. |
Table 1 | Figure 1 | Figure 2 | Figure 3 |
top
Introduction
Vanadium is a refractory and moderately siderophile element with the two naturally occurring isotopes 51V and 50V. Variations of 51V/50V reported for terrestrial and extraterrestrial samples have been attributed to various processes, such as heterogeneous distribution of nucleosynthetically produced V (Nielsen et al., 2019
Nielsen, S.G., Auro, M., Righter, K., Davis, D., Prytulak, J., Wu, F., Owens, J.D. (2019) Nucleosynthetic vanadium isotope heterogeneity of the early solar system recorded in chondritic meteorites. Earth and Planetary Science Letters 505, 131–140.
), kinetic and equilibrium mass dependent isotope fractionation during condensation from the protosolar nebula (Wu et al., 2015Wu, F., Qin, T., Li, X.F., Liu, Y., Huang, J.H., Wu, Z.Q., Huang, F. (2015) First-principles investigation of vanadium isotope fractionation in solution and during adsorption. Earth and Planetary Science Letters 426, 216–224.
; Nielsen et al., 2019Nielsen, S.G., Auro, M., Righter, K., Davis, D., Prytulak, J., Wu, F., Owens, J.D. (2019) Nucleosynthetic vanadium isotope heterogeneity of the early solar system recorded in chondritic meteorites. Earth and Planetary Science Letters 505, 131–140.
), early irradiation by solar irradiation (Lee et al., 1998Lee, T., Shu, F.H., Shang, H., Glassgold, A.E., Rehm, K.E. (1998) Protostellar cosmic rays and extinct radioactivities in meteorites. Astrophysical Journal 506, 898–912.
; Gounelle et al., 2006Gounelle, M., Shu, F.H., Shang, H., Glassgold, A.E., Rehm, K.E., Lee, T. (2006) The irradiation origin of beryllium radioisotopes and other short-lived radionuclides. Astrophysical Journal 640, 1163–1170.
; Sossi et al., 2017Sossi, P.A., Moynier, F., Chaussidon, M., Villeneuve, J., Kato, C., Gounelle, M. (2017) Early Solar System irradiation quantified by linked vanadium and beryllium isotope variations in meteorites. Nature Astronomy 1, 0055.
), exposure of meteoroids to galactic cosmic rays (GCR) during their transition to Earth (Hopkins et al., 2019Hopkins, S.S., Prytulak, J., Barling, J., Russell, S.S., Coles, B.J., Halliday, A.N. (2019) The vanadium isotopic composition of lunar basalts. Earth and Planetary Science Letters 511, 12–24.
), and/or magmatic differentiation (Prytulak et al., 2017Prytulak, J., Sossi, P.A., Halliday, A.N., Plank, T., Savage, P.S., Woodhead, J.D. (2017) Stable vanadium isotopes as a redox proxy in magmatic systems? Geochemical Perspectives Letters 3, 75–84.
). Since V has only two isotopes, it is difficult to discriminate among the different processes that can lead to V isotope variations in geological material. Therefore, additional constraints are needed to interpret V isotope signatures in cosmochemical and geochemical contexts. To further explore the origin of δ51V variations (where δ51Vsample = ((51V/50V)sample/(51V/50V)AA − 1) × 1000, with AA being the Alfa Aesar reference solution) among terrestrial planets, a comprehensive set of V isotope data for martian meteorites was obtained. These data are compared with the value for chondrites of δ51V = −1.089 ± 0.029 ‰ (2 s.e.) (see Supplementary Information) to provide additional constraints on potential accretionary sources for Mars, and to investigate the possible mechanisms of V isotope fractionation during planetary differentiation and igneous processes.top
Results and Discussion
The 24 martian meteorites investigated here represent a petrologic range of shergottites (basaltic, olivine-phyric, lherzolitic), clinopyroxene-rich cumulates (nakhlites) and orthopyroxenite (Table 1). The measured δ51V for martian meteorites display a rather restricted range of values from −1.25 to −0.89 ‰ (Table 1) with a mean of δ51V = −1.043 ± 0.034 ‰ (2 s.e.). Due to their young exposure ages and relatively low Fe/V ratios most samples require very minor, if any, correction for spallation by GCR (see Supplementary Information). Corrected δ51V values are all within error of each other and show no correlation with petrologic type or indicators of melting or fractional crystallisation (Fig. 1). Pooled data for the different martian meteorite groups yield indistinguishable mean δ51V (Table 1; Supplementary Information), further supporting a limited effect of magmatic processes, such as partial melting and fractional crystallisation, on V isotopes. This inference is consistent with the absence of discernible, systematic δ51V variations among terrestrial peridotites and basalts (see Supplementary Information), as well as lunar rock data corrected for GCR effects (Hopkins et al., 2019
Hopkins, S.S., Prytulak, J., Barling, J., Russell, S.S., Coles, B.J., Halliday, A.N. (2019) The vanadium isotopic composition of lunar basalts. Earth and Planetary Science Letters 511, 12–24.
).Table 1 Vanadium isotope data for martian meteorites.
Sample | Fe$ (mg/g) | V$ (μg/g) | CRE age¶ (Ma) | δ51Vmeas | 2 s.d. | splits | n | δ51Vcorr§ |
Basaltic shergottites | ||||||||
Los Angeles 001 | 224 | 258 | 3.0 | −0.95 | 0.12 | 3 | 8 | −0.94 |
NWA 856 | 132 | 273 | 2.6 | −0.94 | 0.13 | 1 | 10 | −0.94 |
NWA 4864 | 153 | 325 | 3.0 | −1.11 | 0.23 | 3 | 8 | −1.10 |
Shergotty | 173 | 310 | 3.0 | −0.89 | 0.19 | 2 | 8 | −0.89 |
Zagami | 141 | 312 | 3.0 | −1.15 | 0.23 | 2 | 14 | −1.14 |
Error weighted group average | −0.97 | |||||||
Olivine-phyric shergottites | ||||||||
EETA 79001 A | 143 | 210 | 0.7 | −1.02 | 0.19 | 2 | 6 | −1.02 |
SaU 005 | 143 | 196 | 1.2 | −1.12 | 0.24 | 2 | 14 | −1.12 |
SaU 051 | 150 | 204 | 1.2 | −1.03 | 0.21 | 2 | 11 | −1.03 |
SaU 094 | 149 | 212 | 1.2 | −1.11 | 0.20 | 2 | 4 | −1.11 |
NWA 1068 | 155 | 207 | 2.8 | −1.11 | 0.16 | 6 | 22 | −1.10 |
LAR 06319 | 159 | 202 | 3.3 | −1.04 | 0.16 | 2 | 5 | −1.03 |
Y-980459 | 144 | 213 | 1.1 | −1.00 | 0.09 | 1 | 4 | −1.00 |
NWA 4925 | 149 | 181 | 0.6 | −0.98 | 0.19 | 3 | 9 | −0.98 |
NWA 6162 | 142 | 112 | 1.0 | −0.90 | 0.15 | 1 | 4 | −0.89 |
DaG476 | 122 | 167 | 1.0 | −1.09 | 0.13 | 3 | 11 | −1.09 |
RBT 04262 | 201 | 212 | 2.1 | −1.04 | 0.06 | 1 | 3 | −1.04 |
Error weighted group average | −1.03 | |||||||
Lherzolitic shergottites | ||||||||
ALH 77005 | 156 | 162 | 4.3 | −1.11 | 0.12 | 3 | 11 | −1.10 |
NWA 1950 | 168 | 140 | 4.1 | −1.00 | 0.10 | 2 | 9 | −0.99 |
Y-000097 | 155 | 180 | 4.6 | −1.01 | 0.14 | 1 | 4 | −1.00 |
Error weighted group average | −1.03 | |||||||
Nakhlites | ||||||||
Nakhla | 160 | 192 | 11.6 | −1.25 | 0.19 | 1 | 5 | −1.23 |
MIL 03346 | 137 | 195 | 9.5 | −1.08 | 0.14 | 2 | 6 | −1.07 |
NWA 817 | 154 | 181 | 10.0 | −0.98 | 0.15 | 1 | 2 | −0.96 |
Y-000593 | 117 | 125 | 11.8 | −1.03 | 0.22 | 1 | 7 | −1.01 |
Error weighted group average | −1.06 | |||||||
Orthopyroxenite | ||||||||
ALH 84001 | 136 | 201 | 14.7 | −1.09 | 0.15 | 1 | 4 | −1.07 |
Error weighted average Mars | −1.026 | |||||||
2 s.e. | 0.027 | |||||||
MSWD | 0.991 |
$ Iron and V concentrations are either from the literature (in italics; Lodders, 1998Lodders, K. (1998) A survey of shergottite, nakhlite and chassigny meteorites whole-rock compositions. Meteoritics & Planetary Science 33, A183–A190.; Dreibus et al., 2000Dreibus, G., Spettel, B., Haubold, R., Jochum, K.P., Palme, H., Wolf, D., Zipfel, J. (2000) Chemistry of a new shergottite: Sayh al Uhaymir 005. Meteoritics and Planetary Science 35, A49.; Sautter et al., 2002Sautter, V., Barrat, J.A., Jambon, A., Lorand, J.P., Gillet, P., Javoy, M., Loron, J.L., Lesourd, M. (2002) A new Martian meteorite from Morocco: the nakhlite North West Africa 817. Earth and Planetary Science Letters 195, 223–238.; Gillet et al., 2005Gillet, P., Barrat, J.A., Beck, P., Marty, B., Greenwood, R.C., Franchi, I.A., Bohn, M., Cotten, J. (2005) Petrology, geochemistry, and cosmic-ray exposure age of lherzolitic shergottite Northwest Africa 1950. Meteoritics & Planetary Science 40, 1175–1184.; Basu Sarbadhikari et al., 2009Basu Sarbadhikari, A., Day, J.M.D., Liu, Y., Rumble, D., Taylor, L.A. (2009) Petrogenesis of olivine-phyric shergottite Larkman Nunatak 06319: implications for enriched components in martian basalts. Geochimica et Cosmochimica Acta 73, 2190–2214.; Shirai and Ebihara, 2009Shirai, N., Ebihara, M. (2009) Chemical characteristics of the lherzolitic shergottite Yamato 000097: magmatism on Mars inferred from the chemical compositions of shergottites. Polar Science 3, 117–133.; Kuehner et al., 2011Kuehner, S.M., Irving, A.J., Herd, C.D.K., Gellissen, M., Lapen, T.J., Rumble, D. (2011) Pristine olivine-phyric shergottite Northwest Africa 6162: a primitive magma with accumulated crystals derived from depleted martian mantle. 42nd Lunar and Planetary Science Conference, The Woodlands, Texas. 1610.), measured by ICPMS at WHOI (bold), or estimated based on yield during column chemistry.
¶ CRE ages from Eugster et al. (2002)Eugster, O., Busemann, H., Lorenzetti, S., Terribilini, D. (2002) Ejection ages from krypton-81-krypton-83 dating and pre-atmospheric sizes of martian meteorites. Meteoritics & Planetary Science 37, 1345–1360., Mathew et al. (2003)Mathew, K.J., Marty, B., Marti, K., Zimmermann, L. (2003) Volatiles (nitrogen, noble gases) in recently discovered SNC meteorites, extinct radioactivities and evolution. Earth and Planetary Science Letters 214, 27–42., Christen et al. (2005)Christen, F., Eugster, O., Busemann, H. (2005) Mars ejection times and neutron capture effects of the nakhlites Y000593 and Y000749, the olivine-phyric shergottite Y980459, and the lherzolite NWA1950. Antarctic Meteorite Research 18, 117–132., Nagao and Park (2008)Nagao, K., Park, J. (2008) Noble gases and cosmic-ray exposure ages of two Martian shergottites, RBT 04262 and LAR 06319, recovered in Antarctica. 71st Annual Meteoritical Society Meeting, Matsue, Japan. 5200., Nagao et al. (2008)Nagao, K., Park, J., Choi, H.G. (2008) Noble gases of the Yamato 000027 and Yamato 000097 lherzolitic shergottites from Mars. Polar Science 2, 195–214., Nishiizumi et al. (2011)Nishiizumi, K., Nagao, K., Caffee, M.W., Jull, A.J.T., Irving, A.J. (2011) Cosmic-ray exposure chronologies of depleted olivine-phyric shergottites. 42nd Lunar and Planetary Science Conference, The Woodlands, Texas. 2371. and Wieler et al. (2016)Wieler, R., Huber, L., Busemann, H., Seiler, S., Leya, I., Maden, C., Masarik, J., Meier, M.M.M., Nagao, K., Trappitsch, R., Irving, A.J. (2016) Noble gases in 18 Martian meteorites and angrite Northwest Africa 7812—Exposure ages, trapped gases, and a re-evaluation of the evidence for solar cosmic ray produced neon in shergottites and other achondrites. Meteoritics & Planetary Science 51, 407–428. except for NWA4864, which is assumed similar to the other basaltic shergottites.
§ Vanadium isotope compositions corrected for spallogenic production of 50V using the equation: δ51Vcorr = δ51Vmeas + [Fe] × CRE × 2.1 × 10−6/[V] (Hopkins et al., 2019Hopkins, S.S., Prytulak, J., Barling, J., Russell, S.S., Coles, B.J., Halliday, A.N. (2019) The vanadium isotopic composition of lunar basalts. Earth and Planetary Science Letters 511, 12–24.).
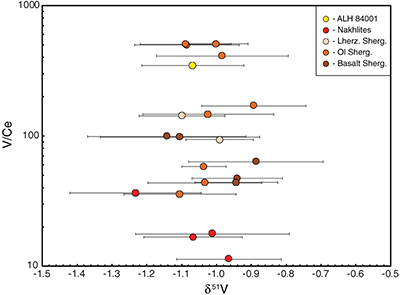
Figure 1 Martian meteorite vanadium isotopic data corrected for GCR effects plotted against V/Ce ratios. Error bars are 2 s.d. as listed in Table 1. Given that V and Ce have different partition coefficients during fractional crystallisation and melting, variations in V/Ce likely reflect a combination of these processes. The lack of systematic V isotope variation over the entire range of V/Ce implies that magmatic processes did not induce detectable V isotope variation on Mars. Ce concentrations from Yoshizaki and McDonough (2020)
Yoshizaki, T., McDonough, W.F. (2020) The composition of Mars. Geochimica et Cosmochimica Acta 273, 137–162.
; V concentrations from Table 1. Note log scale on y axis.Invariant V isotope compositions recorded in martian meteorites collectively indicate that BSM (and by analogy BSE) inherited its δ51V signature during its accretion and/or differentiation history. Here, the GCR corrected and error weighted average V isotope composition of martian meteorites (Table 1) is used to derive the best estimate for the V isotope source signature of BSM, which yields δ51V = −1.026 ± 0.027 ‰ (2 s.e., MSWD = 0.99). This value is markedly different from the mean δ51V of BSE (−0.856 ± 0.020 ‰, 2 s.e.). Understanding the cause(s) of V isotope variations among terrestrial planets could potentially provide constraints on the accretion history and building blocks of Earth and Mars, as well as isotopic effects during planetary differentiation. In the following, we explore possible explanations for the V isotope difference between Mars and chondrites, and discuss the implications for how BSM and BSE could have acquired their V isotope compositions.
Whereas stable isotope systems such as Mg, Si, Ca, and Fe in martian samples (Armytage et al., 2011
Armytage, R.M.G., Georg, R.B., Savage, P.S., Williams, H.M., Halliday, A.N. (2011) Silicon isotopes in meteorites and planetary core formation. Geochimica et Cosmochimica Acta 75, 3662–3676.
; Magna et al., 2015Magna, T., Gussone, N., Mezger, K. (2015) The calcium isotope systematics of Mars. Earth and Planetary Science Letters 430, 86–94.
; Sossi et al., 2016Sossi, P.A., Nebel, O., Anand, M., Poitrasson, F. (2016) On the iron isotope composition of Mars and volatile depletion in the terrestrial planets. Earth and Planetary Science Letters 449, 360–371.
; Hin et al., 2017Hin, R.C., Coath, C.D., Carter, P.J., Nimmo, F., Lai, Y.-J., Pogge von Strandmann, P.A.E., Willbold, M., Leinhardt, Z.M., Walter, M.J., Elliott, T. (2017) Magnesium isotope evidence that accretional vapour loss shapes planetary compositions. Nature 549, 511.
; Magna et al., 2017Magna, T., Hu, Y., Teng, F.-Z., Mezger, K. (2017) Magnesium isotope systematics in Martian meteorites. Earth and Planetary Science Letters 474, 419–426.
) show only limited, if any, deviations from chondritic values, large nucleosynthetic isotope anomalies have been observed for many other elements in chondrites and planetary materials including Mars (e.g., 54Cr, 50Ti, 17O, 62Ni; Warren, 2011Warren, P.H. (2011) Stable-isotopic anomalies and the accretionary assemblage of the Earth and Mars: A subordinate role for carbonaceous chondrites. Earth and Planetary Science Letters 311, 93–100.
). Given that Mars likely accreted very rapidly and early relative to Earth (Dauphas and Pourmand, 2011Dauphas, N., Pourmand, A. (2011) Hf-W-Th evidence for rapid growth of Mars and its status as a planetary embryo. Nature 473, 489–U227.
), the V isotope difference between Mars and Earth could denote primary spatial variability and/or temporal evolution of the nucleosynthetic V isotope composition of planetary accretion source material in the inner Solar System (e.g., Warren, 2011Warren, P.H. (2011) Stable-isotopic anomalies and the accretionary assemblage of the Earth and Mars: A subordinate role for carbonaceous chondrites. Earth and Planetary Science Letters 311, 93–100.
). However, although V isotope compositions of bulk carbonaceous chondrites have been proposed to correlate broadly with nucleosynthetic anomalies of 54Cr (Nielsen et al., 2019Nielsen, S.G., Auro, M., Righter, K., Davis, D., Prytulak, J., Wu, F., Owens, J.D. (2019) Nucleosynthetic vanadium isotope heterogeneity of the early solar system recorded in chondritic meteorites. Earth and Planetary Science Letters 505, 131–140.
), subsequent studies have found that the V isotope variation in bulk chondrites can be ascribed to recent production of 50V from GCR spallation processes (Hopkins et al., 2019Hopkins, S.S., Prytulak, J., Barling, J., Russell, S.S., Coles, B.J., Halliday, A.N. (2019) The vanadium isotopic composition of lunar basalts. Earth and Planetary Science Letters 511, 12–24.
). Carbonaceous, ordinary, enstatite and Rumuruti chondrite data corrected for this effect all display uniform δ51V = −1.089 ± 0.029 ‰ (n = 14, 2 s.e.; see Supplementary Information). The lack of V isotope variation in chondrites compared with the large variations in e.g., 54Cr and 50Ti implies that nucleosynthetic V isotope anomalies, if they exist, are unlikely to induce planetary scale V isotope heterogeneity. Similarly, irradiation processes are unlikely to account for planetary V isotope variations given the large abundance variations of CAIs, the most likely carriers of irradiation-induced V isotope anomalies (Sossi et al., 2017Sossi, P.A., Moynier, F., Chaussidon, M., Villeneuve, J., Kato, C., Gounelle, M. (2017) Early Solar System irradiation quantified by linked vanadium and beryllium isotope variations in meteorites. Nature Astronomy 1, 0055.
), in chondrites that display uniform bulk V isotope compositions. For these reasons, V isotope variations among terrestrial planets most likely do not reflect disparities in the signatures of their accretionary materials, but are the result of planetary processes.Although BSE has a markedly heavier composition than other solar system bodies, there are still analytically significant differences between BSM and chondrites (Fig. 2). Two sample t tests demonstrate that the datasets of the BSM and chondrites are characterised by statistically distinct means (see Supplementary Information). Monte Carlo simulations using the raw datasets of the BSM and chondrites that include the individual sample errors show that there exists a difference of 0.058 ± 0.051 ‰ (1 s.d.) between these two populations, with a probability of ∼87 % that the V isotope composition of the BSM is heavier than that of chondrites. Using the mean δ51V compositions of the BSM and chondrites, instead of the raw data, to compute these Monte Carlo simulations yields a systematic difference of 0.067 ± 0.042 ‰ (1 s.d.) between BSM and chondrites. In the likely absence of mass independent V isotope effects (nucleosynthetic or irradiation-related) on the planetary scale, we propose that the most straightforward explanation for the V isotope disparity between differentiated (Earth, Mars) and non-differentiated (chondrites) bodies corresponds to small but non-negligible isotopic fractionation during core formation.

Figure 2 Vanadium isotope data for different sample types from the Solar System. Data for terrestrial samples are from Prytulak et al. (2013)
Prytulak, J., Nielsen, S.G., Ionov, D.A., Halliday, A.N., Harvey, J., Kelley, K.A., Niu, Y., Peate, D.W., Shimizu, K., Sims, K.W.W. (2013) The Stable Vanadium Isotope Composition of the Mantle and Mafic Lavas. Earth and Planetary Science Letters 365, 177–189.
, Wu et al. (2018)Wu, F., Qi, Y., Perfit, M.R., Gao, Y., Langmuir, C.H., Wanless, V.D., Yu, H., Huang, F. (2018) Vanadium isotope compositions of mid-ocean ridge lavas and altered oceanic crust. Earth and Planetary Science Letters 493, 128–139.
and Qi et al. (2019)Qi, Y.H., Wu, F., Ionov, D.A., Puchtel, I.S., Carlson, R.W., Nicklas, R.W., Yu, H.M., Kang, J.T., Li, C.H., Huang, F. (2019) The vanadium isotopic composition of the BSE: constraints from peridotites and komatiites. Geochimica et Cosmochimica Acta 259, 288–301.
; chondrite data are from Nielsen et al., (2019)Nielsen, S.G., Auro, M., Righter, K., Davis, D., Prytulak, J., Wu, F., Owens, J.D. (2019) Nucleosynthetic vanadium isotope heterogeneity of the early solar system recorded in chondritic meteorites. Earth and Planetary Science Letters 505, 131–140.
and this study (Table S-2). All data have been corrected for spallation-produced 50V. Error bars for each sample group are 2 s.e. weighted by the individual sample error bars shown in Table 1. Shaded areas denote the average for those objects and error weighted 2 s.e. of the entire data population. Chondrite averages have been weighted by individual measurement errors.Considering that (i) the martian core comprises ∼18 % by mass of the planet (Yoshizaki and McDonough, 2020
Yoshizaki, T., McDonough, W.F. (2020) The composition of Mars. Geochimica et Cosmochimica Acta 273, 137–162.
), (ii) ∼27–60 % of martian V resides in the core (see Supplementary Information), and (iii) Δ51VBSM-chondrites = 0.067 ± 0.042 ‰, the martian core should be characterised by δ51V ∼ −1.11 to −1.38 ‰. This requires a metal-silicate isotope fractionation factor of Δ51Vcore-mantle = −0.04 to −0.40 ‰ (Fig. 3). Regarding BSE, it is generally assumed that the main phase of metal segregation during terrestrial core formation readily accounts for the depletion of V in the silicate Earth (O’Neill, 1991O’Neill, H.S.C. (1991) The origin of the moon and the early history of the earth – A chemical model. Part 2: The earth. Geochimica et Cosmochimica Acta 55, 1159–1172.
; Chabot and Agee, 2003Chabot, N.L., Agee, C.B. (2003) Core formation in the Earth and Moon: New experimental constraints from V, Cr, and Mn. Geochimica et Cosmochimica Acta 67, 2077–2091.
; Wade and Wood, 2005Wade, J., Wood, B.J. (2005) Core formation and the oxidation state of the Earth. Earth and Planetary Science Letters 236, 78–95.
), with 40–50 % of terrestrial V now residing in the core (e.g., Wade and Wood, 2005Wade, J., Wood, B.J. (2005) Core formation and the oxidation state of the Earth. Earth and Planetary Science Letters 236, 78–95.
). If bulk Earth is taken to be chondritic for V isotopes, then mass balance dictates that the core is characterised by δ51V = −1.39 ± 0.10 ‰, which corresponds to a metal-silicate isotope fractionation factor of Δ51Vcore-mantle = −0.53 ± 0.14 ‰ (using Δ51VBSE-chondrites = 0.233 ± 0.037 ‰, 2 s.e.). This value is somewhat larger than that required to explain the BSM–chondrite V isotope offset (Fig. 3), which could imply variations in V isotope fractionation during planetary differentiation.
Figure 3 Modelled V isotope fractionation factors between the core and mantle of Earth (blue) and Mars (red) as a function of the fraction of total V that entered the core.
The metal-silicate isotope fractionation factor required to satisfy V geochemical constraints (Δ51Vmetal-silicate up to −0.6 ‰) is substantially larger than values found for other redox sensitive stable isotope systems like Mo, Fe and Cr (all <0.1 ‰; Hin et al. (2013)
Hin, R.C., Burkhardt, C., Schmidt, M.W., Bourdon, B., Kleine, T. (2013) Experimental evidence for Mo isotope fractionation between metal and silicate liquids. Earth and Planetary Science Letters 379, 38–48.
, Bonnand et al. (2016)Bonnand, P., Williams, H.M., Parkinson, I.J., Wood, B.J., Halliday, A.N. (2016) Stable chromium isotopic composition of meteorites and metal-silicate experiments: Implications for fractionation during core formation. Earth and Planetary Science Letters 435, 14–21.
, and Elardo et al., (2019)Elardo, S.M., Shahar, A., Mock, T.D., Sio, C.K. (2019) The effect of core composition on iron isotope fractionation between planetary cores and mantles. Earth and Planetary Science Letters 513, 124–134.
). A small core–mantle isotope fractionation factor for V is also suggested by the only available investigation of V isotope fractionation during metal–silicate partitioning, where experiments at 1.5 GPa and ∼1900 K revealed no detectable V isotope fractionation outside the analytical uncertainty (∼±0.2 ‰; Nielsen et al., 2014Nielsen, S.G., Prytulak, J., Wood, B.J., Halliday, A.N. (2014) Vanadium isotopic difference between the silicate Earth and meteorites. Earth and Planetary Science Letters 389, 167–175.
). However, Earth’s core likely formed through a complex, multi-stage process, starting as a reduced body that gradually evolved to more oxidised as Earth grew (e.g., Wade and Wood, 2005Wade, J., Wood, B.J. (2005) Core formation and the oxidation state of the Earth. Earth and Planetary Science Letters 236, 78–95.
), with a final stage of core segregation in the form of immiscible metal and sulfide melts after the Moon-forming giant impact (e.g., O’Neill, 1991O’Neill, H.S.C. (1991) The origin of the moon and the early history of the earth – A chemical model. Part 2: The earth. Geochimica et Cosmochimica Acta 55, 1159–1172.
). Additional experiments of V isotopic partitioning at higher pressure-temperature conditions, for variable oxygen fugacities and/or chemical compositions (e.g., presence or not of light elements and other metal alloys) are needed to test the hypothesis of V isotope fractionation during core formation. Such effects have, for example, been observed for Fe isotopes (Elardo et al., 2019Elardo, S.M., Shahar, A., Mock, T.D., Sio, C.K. (2019) The effect of core composition on iron isotope fractionation between planetary cores and mantles. Earth and Planetary Science Letters 513, 124–134.
). Notably, the sensitivity of V valence state to the oxygen fugacity during core formation offers a promising alternative for potentially inducing significant V isotope fractionation during metal-silicate partitioning. For example, the oxygen fugacity during core formation on Mars and Earth were likely different (Wood et al., 2006Wood, B.J., Walter, M.J., Wade, J. (2006) Accretion of the Earth and segregation of its core. Nature 441, 825–833.
) and may have been a contributing factor in the apparent V isotope fractionation difference between these two planets.One outstanding challenge is to explain the strikingly heavy V isotope composition of BSE compared to BSM. Future experimental investigations may shed light on the possibility for higher temperatures and pressures during terrestrial core formation, and/or late segregation of the Hadean matte (which seemingly did not happen on Mars) to account for this difference. Based on current constraints, it appears that the late stage sulfide segregation on Earth is unlikely to have removed substantial amounts of V to the core (e.g., Wade and Wood, 2005
Wade, J., Wood, B.J. (2005) Core formation and the oxidation state of the Earth. Earth and Planetary Science Letters 236, 78–95.
). We, therefore, consider that the heavy V isotope composition of BSE was most likely established during the main phase of core segregation, before Moon formation. Alternative avenues of investigation to explain the heavy V isotope composition of BSE and BSM may require unusual isotope fractionation processes to operate at the core–mantle boundary, as recently proposed for Fe in the case of thermodiffusion (Lesher et al., 2020Lesher, C.E., Dannberg, J., Barfod, G.H., Bennett, N.R., Glessner, J.J.G., Lacks, D.J., Brenan, J.M. (2020) Iron isotope fractionation at the core–mantle boundary by thermodiffusion. Nature Geoscience 13, 382–386.
). Nonetheless, we speculate that identifying the process(es) responsible for V isotope variations in terrestrial planets will ultimately allow better understanding the conditions of planetary differentiation in the Solar System.top
Acknowledgements
This work was funded by NASA Emerging Worlds grant NNX16AD36G to SGN. Samples were acquired with funds from the Helmholtz Association through the research alliance HA 203 “Planetary Evolution and Life” to KM. TM contributed through the Strategic Research Plan of the Czech Geological Survey (DKRVO/ČGS 2018-2022). KM acknowledges support through NCCR PlanetS supported by the Swiss National Science Foundation. We thank Jurek Blusztajn for support in the WHOI Plasma Facility.
Editor: Anat Shahar
top
References
Armytage, R.M.G., Georg, R.B., Savage, P.S., Williams, H.M., Halliday, A.N. (2011) Silicon isotopes in meteorites and planetary core formation. Geochimica et Cosmochimica Acta 75, 3662–3676.

Whereas stable isotope systems such as Mg, Si, Ca, and Fe in martian samples (Armytage et al., 2011; Magna et al., 2015; Sossi et al., 2016; Hin et al., 2017; Magna et al., 2017) show only limited, if any, deviations from chondritic values, large nucleosynthetic isotope anomalies have been observed for many other elements in chondrites and planetary materials including Mars (e.g., 54Cr, 50Ti, 17O, 62Ni; Warren, 2011).
View in article
Basu Sarbadhikari, A., Day, J.M.D., Liu, Y., Rumble, D., Taylor, L.A. (2009) Petrogenesis of olivine-phyric shergottite Larkman Nunatak 06319: implications for enriched components in martian basalts. Geochimica et Cosmochimica Acta 73, 2190–2214.

Iron and V concentrations are either from the literature (in italics; Lodders, 1998; Dreibus et al., 2000; Sautter et al., 2002; Gillet et al., 2005; Basu Sarbadhikari et al., 2009; Shirai and Ebihara, 2009; Kuehner et al., 2011), measured by ICPMS at WHOI (bold), or estimated based on yield during column chemistry.
View in article
Bonnand, P., Williams, H.M., Parkinson, I.J., Wood, B.J., Halliday, A.N. (2016) Stable chromium isotopic composition of meteorites and metal-silicate experiments: Implications for fractionation during core formation. Earth and Planetary Science Letters 435, 14–21.

The metal-silicate isotope fractionation factor required to satisfy V geochemical constraints (Δ51Vmetal-silicate up to −0.6 ‰) is substantially larger than values found for other redox sensitive stable isotope systems like Mo, Fe and Cr (all <0.1 ‰; Hin et al. (2013), Bonnand et al. (2016), and Elardo et al., (2019)).
View in article
Chabot, N.L., Agee, C.B. (2003) Core formation in the Earth and Moon: New experimental constraints from V, Cr, and Mn. Geochimica et Cosmochimica Acta 67, 2077–2091.

Regarding BSE, it is generally assumed that the main phase of metal segregation during terrestrial core formation readily accounts for the depletion of V in the silicate Earth (O’Neill, 1991; Chabot and Agee, 2003; Wade and Wood, 2005), with 40–50 % of terrestrial V now residing in the core (e.g., Wade and Wood, 2005).
View in article
Christen, F., Eugster, O., Busemann, H. (2005) Mars ejection times and neutron capture effects of the nakhlites Y000593 and Y000749, the olivine-phyric shergottite Y980459, and the lherzolite NWA1950. Antarctic Meteorite Research 18, 117–132.

CRE ages from Eugster et al. (2002), Mathew et al. (2003), Christen et al. (2005), Nagao and Park (2008), Nagao et al. (2008), Nishiizumi et al. (2011) and Wieler et al. (2016) except for NWA4864, which is assumed similar to the other basaltic shergottites.
View in article
Dauphas, N., Pourmand, A. (2011) Hf-W-Th evidence for rapid growth of Mars and its status as a planetary embryo. Nature 473, 489–U227.

Given that Mars likely accreted very rapidly and early relative to Earth (Dauphas and Pourmand, 2011), the V isotope difference between Mars and Earth could denote primary spatial variability and/or temporal evolution of the nucleosynthetic V isotope composition of planetary accretion source material in the inner Solar System (e.g., Warren, 2011).
View in article
Dreibus, G., Spettel, B., Haubold, R., Jochum, K.P., Palme, H., Wolf, D., Zipfel, J. (2000) Chemistry of a new shergottite: Sayh al Uhaymir 005. Meteoritics and Planetary Science 35, A49.

Iron and V concentrations are either from the literature (in italics; Lodders, 1998; Dreibus et al., 2000; Sautter et al., 2002; Gillet et al., 2005; Basu Sarbadhikari et al., 2009; Shirai and Ebihara, 2009; Kuehner et al., 2011), measured by ICPMS at WHOI (bold), or estimated based on yield during column chemistry.
View in article
Elardo, S.M., Shahar, A., Mock, T.D., Sio, C.K. (2019) The effect of core composition on iron isotope fractionation between planetary cores and mantles. Earth and Planetary Science Letters 513, 124–134.

The metal-silicate isotope fractionation factor required to satisfy V geochemical constraints (Δ51Vmetal-silicate up to −0.6 ‰) is substantially larger than values found for other redox sensitive stable isotope systems like Mo, Fe and Cr (all <0.1 ‰; Hin et al. (2013), Bonnand et al. (2016), and Elardo et al., (2019)).
View in article
Such effects have, for example, been observed for Fe isotopes (Elardo et al., 2019).
View in article
Eugster, O., Busemann, H., Lorenzetti, S., Terribilini, D. (2002) Ejection ages from krypton-81-krypton-83 dating and pre-atmospheric sizes of martian meteorites. Meteoritics & Planetary Science 37, 1345–1360.

CRE ages from Eugster et al. (2002), Mathew et al. (2003), Christen et al. (2005), Nagao and Park (2008), Nagao et al. (2008), Nishiizumi et al. (2011) and Wieler et al. (2016) except for NWA4864, which is assumed similar to the other basaltic shergottites.
View in article
Gillet, P., Barrat, J.A., Beck, P., Marty, B., Greenwood, R.C., Franchi, I.A., Bohn, M., Cotten, J. (2005) Petrology, geochemistry, and cosmic-ray exposure age of lherzolitic shergottite Northwest Africa 1950. Meteoritics & Planetary Science 40, 1175–1184.

Iron and V concentrations are either from the literature (in italics; Lodders, 1998; Dreibus et al., 2000; Sautter et al., 2002; Gillet et al., 2005; Basu Sarbadhikari et al., 2009; Shirai and Ebihara, 2009; Kuehner et al., 2011), measured by ICPMS at WHOI (bold), or estimated based on yield during column chemistry.
View in article
Gounelle, M., Shu, F.H., Shang, H., Glassgold, A.E., Rehm, K.E., Lee, T. (2006) The irradiation origin of beryllium radioisotopes and other short-lived radionuclides. Astrophysical Journal 640, 1163–1170.

Variations of 51V/50V reported for terrestrial and extraterrestrial samples have been attributed to various processes, such as heterogeneous distribution of nucleosynthetically produced V (Nielsen et al., 2019), kinetic and equilibrium mass dependent isotope fractionation during condensation from the protosolar nebula (Wu et al., 2015; Nielsen et al., 2019), early irradiation by solar irradiation (Lee et al., 1998; Gounelle et al., 2006; Sossi et al., 2017), exposure of meteoroids to galactic cosmic rays (GCR) during their transition to Earth (Hopkins et al., 2019), and/or magmatic differentiation (Prytulak et al., 2017).
View in article
Hin, R.C., Burkhardt, C., Schmidt, M.W., Bourdon, B., Kleine, T. (2013) Experimental evidence for Mo isotope fractionation between metal and silicate liquids. Earth and Planetary Science Letters 379, 38–48.

The metal-silicate isotope fractionation factor required to satisfy V geochemical constraints (Δ51Vmetal-silicate up to −0.6 ‰) is substantially larger than values found for other redox sensitive stable isotope systems like Mo, Fe and Cr (all <0.1 ‰; Hin et al. (2013), Bonnand et al. (2016), and Elardo et al., (2019)).
View in article
Hin, R.C., Coath, C.D., Carter, P.J., Nimmo, F., Lai, Y.-J., Pogge von Strandmann, P.A.E., Willbold, M., Leinhardt, Z.M., Walter, M.J., Elliott, T. (2017) Magnesium isotope evidence that accretional vapour loss shapes planetary compositions. Nature 549, 511.

Whereas stable isotope systems such as Mg, Si, Ca, and Fe in martian samples (Armytage et al., 2011; Magna et al., 2015; Sossi et al., 2016; Hin et al., 2017; Magna et al., 2017) show only limited, if any, deviations from chondritic values, large nucleosynthetic isotope anomalies have been observed for many other elements in chondrites and planetary materials including Mars (e.g., 54Cr, 50Ti, 17O, 62Ni; Warren, 2011).
View in article
Hopkins, S.S., Prytulak, J., Barling, J., Russell, S.S., Coles, B.J., Halliday, A.N. (2019) The vanadium isotopic composition of lunar basalts. Earth and Planetary Science Letters 511, 12–24.

Variations of 51V/50V reported for terrestrial and extraterrestrial samples have been attributed to various processes, such as heterogeneous distribution of nucleosynthetically produced V (Nielsen et al., 2019), kinetic and equilibrium mass dependent isotope fractionation during condensation from the protosolar nebula (Wu et al., 2015; Nielsen et al., 2019), early irradiation by solar irradiation (Lee et al., 1998; Gounelle et al., 2006; Sossi et al., 2017), exposure of meteoroids to galactic cosmic rays (GCR) during their transition to Earth (Hopkins et al., 2019), and/or magmatic differentiation (Prytulak et al., 2017).
View in article
This inference is consistent with the absence of discernible, systematic δ51V variations among terrestrial peridotites and basalts (see Supplementary Information), as well as lunar rock data corrected for GCR effects (Hopkins et al., 2019).
View in article
Vanadium isotope compositions corrected for spallogenic production of 50V using the equation: δ51Vcorr = δ51Vmeas + [Fe] × CRE × 2.1 × 10−6/[V] (Hopkins et al., 2019).
View in article
However, although V isotope compositions of bulk carbonaceous chondrites have been proposed to correlate broadly with nucleosynthetic anomalies of 54Cr (Nielsen et al., 2019), subsequent studies have found that the V isotope variation in bulk chondrites can be ascribed to recent production of 50V from GCR spallation processes (Hopkins et al., 2019).
View in article
Kuehner, S.M., Irving, A.J., Herd, C.D.K., Gellissen, M., Lapen, T.J., Rumble, D. (2011) Pristine olivine-phyric shergottite Northwest Africa 6162: a primitive magma with accumulated crystals derived from depleted martian mantle. 42nd Lunar and Planetary Science Conference, The Woodlands, Texas. 1610.

Iron and V concentrations are either from the literature (in italics; Lodders, 1998; Dreibus et al., 2000; Sautter et al., 2002; Gillet et al., 2005; Basu Sarbadhikari et al., 2009; Shirai and Ebihara, 2009; Kuehner et al., 2011), measured by ICPMS at WHOI (bold), or estimated based on yield during column chemistry.
View in article
Lee, T., Shu, F.H., Shang, H., Glassgold, A.E., Rehm, K.E. (1998) Protostellar cosmic rays and extinct radioactivities in meteorites. Astrophysical Journal 506, 898–912.

Variations of 51V/50V reported for terrestrial and extraterrestrial samples have been attributed to various processes, such as heterogeneous distribution of nucleosynthetically produced V (Nielsen et al., 2019), kinetic and equilibrium mass dependent isotope fractionation during condensation from the protosolar nebula (Wu et al., 2015; Nielsen et al., 2019), early irradiation by solar irradiation (Lee et al., 1998; Gounelle et al., 2006; Sossi et al., 2017), exposure of meteoroids to galactic cosmic rays (GCR) during their transition to Earth (Hopkins et al., 2019), and/or magmatic differentiation (Prytulak et al., 2017).
View in article
Lesher, C.E., Dannberg, J., Barfod, G.H., Bennett, N.R., Glessner, J.J.G., Lacks, D.J., Brenan, J.M. (2020) Iron isotope fractionation at the core–mantle boundary by thermodiffusion. Nature Geoscience 13, 382–386.

Alternative avenues of investigation to explain the heavy V isotope composition of BSE and BSM may require unusual isotope fractionation processes to operate at the core–mantle boundary, as recently proposed for Fe in the case of thermodiffusion (Lesher et al., 2020).
View in article
Lodders, K. (1998) A survey of shergottite, nakhlite and chassigny meteorites whole-rock compositions. Meteoritics & Planetary Science 33, A183–A190.

Iron and V concentrations are either from the literature (in italics; Lodders, 1998; Dreibus et al., 2000; Sautter et al., 2002; Gillet et al., 2005; Basu Sarbadhikari et al., 2009; Shirai and Ebihara, 2009; Kuehner et al., 2011), measured by ICPMS at WHOI (bold), or estimated based on yield during column chemistry.
View in article
Magna, T., Gussone, N., Mezger, K. (2015) The calcium isotope systematics of Mars. Earth and Planetary Science Letters 430, 86–94.

Whereas stable isotope systems such as Mg, Si, Ca, and Fe in martian samples (Armytage et al., 2011; Magna et al., 2015; Sossi et al., 2016; Hin et al., 2017; Magna et al., 2017) show only limited, if any, deviations from chondritic values, large nucleosynthetic isotope anomalies have been observed for many other elements in chondrites and planetary materials including Mars (e.g., 54Cr, 50Ti, 17O, 62Ni; Warren, 2011).
View in article
Magna, T., Hu, Y., Teng, F.-Z., Mezger, K. (2017) Magnesium isotope systematics in Martian meteorites. Earth and Planetary Science Letters 474, 419–426.

Whereas stable isotope systems such as Mg, Si, Ca, and Fe in martian samples (Armytage et al., 2011; Magna et al., 2015; Sossi et al., 2016; Hin et al., 2017; Magna et al., 2017) show only limited, if any, deviations from chondritic values, large nucleosynthetic isotope anomalies have been observed for many other elements in chondrites and planetary materials including Mars (e.g., 54Cr, 50Ti, 17O, 62Ni; Warren, 2011).
View in article
Mathew, K.J., Marty, B., Marti, K., Zimmermann, L. (2003) Volatiles (nitrogen, noble gases) in recently discovered SNC meteorites, extinct radioactivities and evolution. Earth and Planetary Science Letters 214, 27–42.

CRE ages from Eugster et al. (2002), Mathew et al. (2003), Christen et al. (2005), Nagao and Park (2008), Nagao et al. (2008), Nishiizumi et al. (2011) and Wieler et al. (2016) except for NWA4864, which is assumed similar to the other basaltic shergottites.
View in article
Nagao, K., Park, J. (2008) Noble gases and cosmic-ray exposure ages of two Martian shergottites, RBT 04262 and LAR 06319, recovered in Antarctica. 71st Annual Meteoritical Society Meeting, Matsue, Japan. 5200.

CRE ages from Eugster et al. (2002), Mathew et al. (2003), Christen et al. (2005), Nagao and Park (2008), Nagao et al. (2008), Nishiizumi et al. (2011) and Wieler et al. (2016) except for NWA4864, which is assumed similar to the other basaltic shergottites.
View in article
Nagao, K., Park, J., Choi, H.G. (2008) Noble gases of the Yamato 000027 and Yamato 000097 lherzolitic shergottites from Mars. Polar Science 2, 195–214.

CRE ages from Eugster et al. (2002), Mathew et al. (2003), Christen et al. (2005), Nagao and Park (2008), Nagao et al. (2008), Nishiizumi et al. (2011) and Wieler et al. (2016) except for NWA4864, which is assumed similar to the other basaltic shergottites.
View in article
Nielsen, S.G., Prytulak, J., Wood, B.J., Halliday, A.N. (2014) Vanadium isotopic difference between the silicate Earth and meteorites. Earth and Planetary Science Letters 389, 167–175.

A small core–mantle isotope fractionation factor for V is also suggested by the only available investigation of V isotope fractionation during metal–silicate partitioning, where experiments at 1.5 GPa and ∼1900 K revealed no detectable V isotope fractionation outside the analytical uncertainty (∼±0.2 ‰; Nielsen et al., 2014).
View in article
Nielsen, S.G., Auro, M., Righter, K., Davis, D., Prytulak, J., Wu, F., Owens, J.D. (2019) Nucleosynthetic vanadium isotope heterogeneity of the early solar system recorded in chondritic meteorites. Earth and Planetary Science Letters 505, 131–140.

Variations of 51V/50V reported for terrestrial and extraterrestrial samples have been attributed to various processes, such as heterogeneous distribution of nucleosynthetically produced V (Nielsen et al., 2019), kinetic and equilibrium mass dependent isotope fractionation during condensation from the protosolar nebula (Wu et al., 2015; Nielsen et al., 2019), early irradiation by solar irradiation (Lee et al., 1998; Gounelle et al., 2006; Sossi et al., 2017), exposure of meteoroids to galactic cosmic rays (GCR) during their transition to Earth (Hopkins et al., 2019), and/or magmatic differentiation (Prytulak et al., 2017).
View in article
However, although V isotope compositions of bulk carbonaceous chondrites have been proposed to correlate broadly with nucleosynthetic anomalies of 54Cr (Nielsen et al., 2019), subsequent studies have found that the V isotope variation in bulk chondrites can be ascribed to recent production of 50V from GCR spallation processes (Hopkins et al., 2019).
View in article
Vanadium isotope data for different sample types from the Solar System. Data for terrestrial samples are from Prytulak et al. (2013), Wu et al. (2018) and Qi et al. (2019); chondrite data are from Nielsen et al., (2019) and this study (Table S-2).
View in article
Nishiizumi, K., Nagao, K., Caffee, M.W., Jull, A.J.T., Irving, A.J. (2011) Cosmic-ray exposure chronologies of depleted olivine-phyric shergottites. 42nd Lunar and Planetary Science Conference, The Woodlands, Texas. 2371.

CRE ages from Eugster et al. (2002), Mathew et al. (2003), Christen et al. (2005), Nagao and Park (2008), Nagao et al. (2008), Nishiizumi et al. (2011) and Wieler et al. (2016) except for NWA4864, which is assumed similar to the other basaltic shergottites.
View in article
O’Neill, H.S.C. (1991) The origin of the moon and the early history of the earth – A chemical model. Part 2: The earth. Geochimica et Cosmochimica Acta 55, 1159–1172.

Regarding BSE, it is generally assumed that the main phase of metal segregation during terrestrial core formation readily accounts for the depletion of V in the silicate Earth (O’Neill, 1991; Chabot and Agee, 2003; Wade and Wood, 2005), with 40–50 % of terrestrial V now residing in the core (e.g., Wade and Wood, 2005).
View in article
However, Earth’s core likely formed through a complex, multi-stage process, starting as a reduced body that gradually evolved to more oxidised as Earth grew (e.g., Wade and Wood, 2005), with a final stage of core segregation in the form of immiscible metal and sulfide melts after the Moon-forming giant impact (e.g., O’Neill, 1991).
View in article
Prytulak, J., Nielsen, S.G., Ionov, D.A., Halliday, A.N., Harvey, J., Kelley, K.A., Niu, Y., Peate, D.W., Shimizu, K., Sims, K.W.W. (2013) The Stable Vanadium Isotope Composition of the Mantle and Mafic Lavas. Earth and Planetary Science Letters 365, 177–189.

Vanadium isotope data for different sample types from the Solar System. Data for terrestrial samples are from Prytulak et al. (2013), Wu et al. (2018) and Qi et al. (2019); chondrite data are from Nielsen et al., (2019) and this study (Table S-2).
View in article
Prytulak, J., Sossi, P.A., Halliday, A.N., Plank, T., Savage, P.S., Woodhead, J.D. (2017) Stable vanadium isotopes as a redox proxy in magmatic systems? Geochemical Perspectives Letters 3, 75–84.

Variations of 51V/50V reported for terrestrial and extraterrestrial samples have been attributed to various processes, such as heterogeneous distribution of nucleosynthetically produced V (Nielsen et al., 2019), kinetic and equilibrium mass dependent isotope fractionation during condensation from the protosolar nebula (Wu et al., 2015; Nielsen et al., 2019), early irradiation by solar irradiation (Lee et al., 1998; Gounelle et al., 2006; Sossi et al., 2017), exposure of meteoroids to galactic cosmic rays (GCR) during their transition to Earth (Hopkins et al., 2019), and/or magmatic differentiation (Prytulak et al., 2017).
View in article
Qi, Y.H., Wu, F., Ionov, D.A., Puchtel, I.S., Carlson, R.W., Nicklas, R.W., Yu, H.M., Kang, J.T., Li, C.H., Huang, F. (2019) The vanadium isotopic composition of the BSE: constraints from peridotites and komatiites. Geochimica et Cosmochimica Acta 259, 288–301.

Vanadium isotope data for different sample types from the Solar System. Data for terrestrial samples are from Prytulak et al. (2013), Wu et al. (2018) and Qi et al. (2019); chondrite data are from Nielsen et al., (2019) and this study (Table S-2).
View in article
Sautter, V., Barrat, J.A., Jambon, A., Lorand, J.P., Gillet, P., Javoy, M., Loron, J.L., Lesourd, M. (2002) A new Martian meteorite from Morocco: the nakhlite North West Africa 817. Earth and Planetary Science Letters 195, 223–238.

Iron and V concentrations are either from the literature (in italics; Lodders, 1998; Dreibus et al., 2000; Sautter et al., 2002; Gillet et al., 2005; Basu Sarbadhikari et al., 2009; Shirai and Ebihara, 2009; Kuehner et al., 2011), measured by ICPMS at WHOI (bold), or estimated based on yield during column chemistry.
View in article
Shirai, N., Ebihara, M. (2009) Chemical characteristics of the lherzolitic shergottite Yamato 000097: magmatism on Mars inferred from the chemical compositions of shergottites. Polar Science 3, 117–133.

Iron and V concentrations are either from the literature (in italics; Lodders, 1998; Dreibus et al., 2000; Sautter et al., 2002; Gillet et al., 2005; Basu Sarbadhikari et al., 2009; Shirai and Ebihara, 2009; Kuehner et al., 2011), measured by ICPMS at WHOI (bold), or estimated based on yield during column chemistry.
View in article
Sossi, P.A., Nebel, O., Anand, M., Poitrasson, F. (2016) On the iron isotope composition of Mars and volatile depletion in the terrestrial planets. Earth and Planetary Science Letters 449, 360–371.

Whereas stable isotope systems such as Mg, Si, Ca, and Fe in martian samples (Armytage et al., 2011; Magna et al., 2015; Sossi et al., 2016; Hin et al., 2017; Magna et al., 2017) show only limited, if any, deviations from chondritic values, large nucleosynthetic isotope anomalies have been observed for many other elements in chondrites and planetary materials including Mars (e.g., 54Cr, 50Ti, 17O, 62Ni; Warren, 2011).
View in article
Sossi, P.A., Moynier, F., Chaussidon, M., Villeneuve, J., Kato, C., Gounelle, M. (2017) Early Solar System irradiation quantified by linked vanadium and beryllium isotope variations in meteorites. Nature Astronomy 1, 0055.

Variations of 51V/50V reported for terrestrial and extraterrestrial samples have been attributed to various processes, such as heterogeneous distribution of nucleosynthetically produced V (Nielsen et al., 2019), kinetic and equilibrium mass dependent isotope fractionation during condensation from the protosolar nebula (Wu et al., 2015; Nielsen et al., 2019), early irradiation by solar irradiation (Lee et al., 1998; Gounelle et al., 2006; Sossi et al., 2017), exposure of meteoroids to galactic cosmic rays (GCR) during their transition to Earth (Hopkins et al., 2019), and/or magmatic differentiation (Prytulak et al., 2017).
View in article
Similarly, irradiation processes are unlikely to account for planetary V isotope variations given the large abundance variations of CAIs, the most likely carriers of irradiation-induced V isotope anomalies (Sossi et al., 2017), in chondrites that display uniform bulk V isotope compositions.
View in article
Wade, J., Wood, B.J. (2005) Core formation and the oxidation state of the Earth. Earth and Planetary Science Letters 236, 78–95.

Regarding BSE, it is generally assumed that the main phase of metal segregation during terrestrial core formation readily accounts for the depletion of V in the silicate Earth (O’Neill, 1991; Chabot and Agee, 2003; Wade and Wood, 2005), with 40–50 % of terrestrial V now residing in the core (e.g., Wade and Wood, 2005).
View in article
However, Earth’s core likely formed through a complex, multi-stage process, starting as a reduced body that gradually evolved to more oxidised as Earth grew (e.g., Wade and Wood, 2005), with a final stage of core segregation in the form of immiscible metal and sulfide melts after the Moon-forming giant impact (e.g., O’Neill, 1991).
View in article
Based on current constraints, it appears that the late stage sulfide segregation on Earth is unlikely to have removed substantial amounts of V to the core (e.g., Wade and Wood, 2005).
View in article
Warren, P.H. (2011) Stable-isotopic anomalies and the accretionary assemblage of the Earth and Mars: A subordinate role for carbonaceous chondrites. Earth and Planetary Science Letters 311, 93–100.

Whereas stable isotope systems such as Mg, Si, Ca, and Fe in martian samples (Armytage et al., 2011; Magna et al., 2015; Sossi et al., 2016; Hin et al., 2017; Magna et al., 2017) show only limited, if any, deviations from chondritic values, large nucleosynthetic isotope anomalies have been observed for many other elements in chondrites and planetary materials including Mars (e.g., 54Cr, 50Ti, 17O, 62Ni; Warren, 2011).
View in article
Given that Mars likely accreted very rapidly and early relative to Earth (Dauphas and Pourmand, 2011), the V isotope difference between Mars and Earth could denote primary spatial variability and/or temporal evolution of the nucleosynthetic V isotope composition of planetary accretion source material in the inner Solar System (e.g., Warren, 2011).
View in article
Wieler, R., Huber, L., Busemann, H., Seiler, S., Leya, I., Maden, C., Masarik, J., Meier, M.M.M., Nagao, K., Trappitsch, R., Irving, A.J. (2016) Noble gases in 18 Martian meteorites and angrite Northwest Africa 7812—Exposure ages, trapped gases, and a re-evaluation of the evidence for solar cosmic ray produced neon in shergottites and other achondrites. Meteoritics & Planetary Science 51, 407–428.

CRE ages from Eugster et al. (2002), Mathew et al. (2003), Christen et al. (2005), Nagao and Park (2008), Nagao et al. (2008), Nishiizumi et al. (2011) and Wieler et al. (2016) except for NWA4864, which is assumed similar to the other basaltic shergottites.
View in article
Wood, B.J., Walter, M.J., Wade, J. (2006) Accretion of the Earth and segregation of its core. Nature 441, 825–833.

For example, the oxygen fugacity during core formation on Mars and Earth were likely different (Wood et al., 2006) and may have been a contributing factor in the apparent V isotope fractionation difference between these two planets.
View in article
Wu, F., Qi, Y., Perfit, M.R., Gao, Y., Langmuir, C.H., Wanless, V.D., Yu, H., Huang, F. (2018) Vanadium isotope compositions of mid-ocean ridge lavas and altered oceanic crust. Earth and Planetary Science Letters 493, 128–139.

Vanadium isotope data for different sample types from the Solar System. Data for terrestrial samples are from Prytulak et al. (2013), Wu et al. (2018) and Qi et al. (2019); chondrite data are from Nielsen et al., (2019) and this study (Table S-2).
View in article
Wu, F., Qin, T., Li, X.F., Liu, Y., Huang, J.H., Wu, Z.Q., Huang, F. (2015) First-principles investigation of vanadium isotope fractionation in solution and during adsorption. Earth and Planetary Science Letters 426, 216–224.

Variations of 51V/50V reported for terrestrial and extraterrestrial samples have been attributed to various processes, such as heterogeneous distribution of nucleosynthetically produced V (Nielsen et al., 2019), kinetic and equilibrium mass dependent isotope fractionation during condensation from the protosolar nebula (Wu et al., 2015; Nielsen et al., 2019), early irradiation by solar irradiation (Lee et al., 1998; Gounelle et al., 2006; Sossi et al., 2017), exposure of meteoroids to galactic cosmic rays (GCR) during their transition to Earth (Hopkins et al., 2019), and/or magmatic differentiation (Prytulak et al., 2017).
View in article
Yoshizaki, T., McDonough, W.F. (2020) The composition of Mars. Geochimica et Cosmochimica Acta 273, 137–162.

Considering that (i) the martian core comprises ∼18 % by mass of the planet (Yoshizaki and McDonough, 2020), (ii) ∼27–60 % of martian V resides in the core (see Supplementary Information), and (iii) Δ51VBSM-chondrites = 0.067 ± 0.042 ‰, the martian core should be characterised by δ51V ∼ −1.11 to −1.38 ‰
View in article
Ce concentrations from Yoshizaki and McDonough (2020); V concentrations from Table 1.
View in article
top
Supplementary Information
The Supplementary Information includes:
- 1. Methods
- 2. Uniformity of the V Isotope Composition of Martian Rocks
- 3. V Isotope Composition of BSE
- 4. V Isotope Composition of Chondrites
- 5. V Isotope Difference between the BSE, BSM and Chondrites
- 6. Assessment of Vanadium Depletion in Bulk Silicate Mars
- Tables S-1 and S-2
- Figures S-1 to S-8
- Supplementary Information References
Download the Supplementary Information (PDF).
Figures and Tables
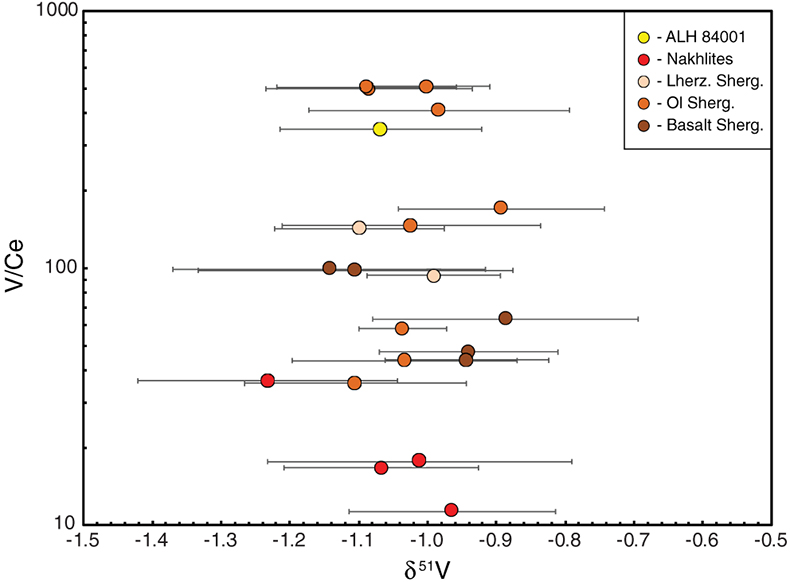
Figure 1 Martian meteorite vanadium isotopic data corrected for GCR effects plotted against V/Ce ratios. Error bars are 2 s.d. as listed in Table 1. Given that V and Ce have different partition coefficients during fractional crystallisation and melting, variations in V/Ce likely reflect a combination of these processes. The lack of systematic V isotope variation over the entire range of V/Ce implies that magmatic processes did not induce detectable V isotope variation on Mars. Ce concentrations from Yoshizaki and McDonough (2020)
Yoshizaki, T., McDonough, W.F. (2020) The composition of Mars. Geochimica et Cosmochimica Acta 273, 137–162.;
V concentrations from Table 1. Note log scale on y axis.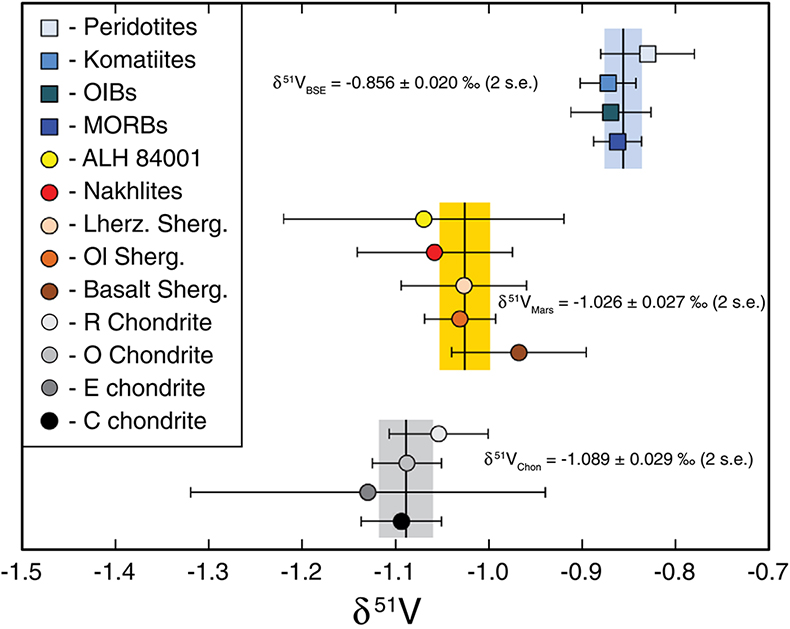
Figure 2 Vanadium isotope data for different sample types from the Solar System. Data for terrestrial samples are from Prytulak et al. (2013)
Prytulak, J., Nielsen, S.G., Ionov, D.A., Halliday, A.N., Harvey, J., Kelley, K.A., Niu, Y., Peate, D.W., Shimizu, K., Sims, K.W.W. (2013) The Stable Vanadium Isotope Composition of the Mantle and Mafic Lavas. Earth and Planetary Science Letters 365, 177–189.
, Wu et al. (2018)Wu, F., Qi, Y., Perfit, M.R., Gao, Y., Langmuir, C.H., Wanless, V.D., Yu, H., Huang, F. (2018) Vanadium isotope compositions of mid-ocean ridge lavas and altered oceanic crust. Earth and Planetary Science Letters 493, 128–139.
and Qi et al. (2019)Qi, Y.H., Wu, F., Ionov, D.A., Puchtel, I.S., Carlson, R.W., Nicklas, R.W., Yu, H.M., Kang, J.T., Li, C.H., Huang, F. (2019) The vanadium isotopic composition of the BSE: constraints from peridotites and komatiites. Geochimica et Cosmochimica Acta 259, 288–301.
; chondrite data are from Nielsen et al., (2019)Nielsen, S.G., Auro, M., Righter, K., Davis, D., Prytulak, J., Wu, F., Owens, J.D. (2019) Nucleosynthetic vanadium isotope heterogeneity of the early solar system recorded in chondritic meteorites. Earth and Planetary Science Letters 505, 131–140.
and this study (Table S-2). All data have been corrected for spallation-produced 50V. Error bars for each sample group are 2 s.e. weighted by the individual sample error bars shown in Table 1. Shaded areas denote the average for those objects and error weighted 2 s.e. of the entire data population. Chondrite averages have been weighted by individual measurement errors.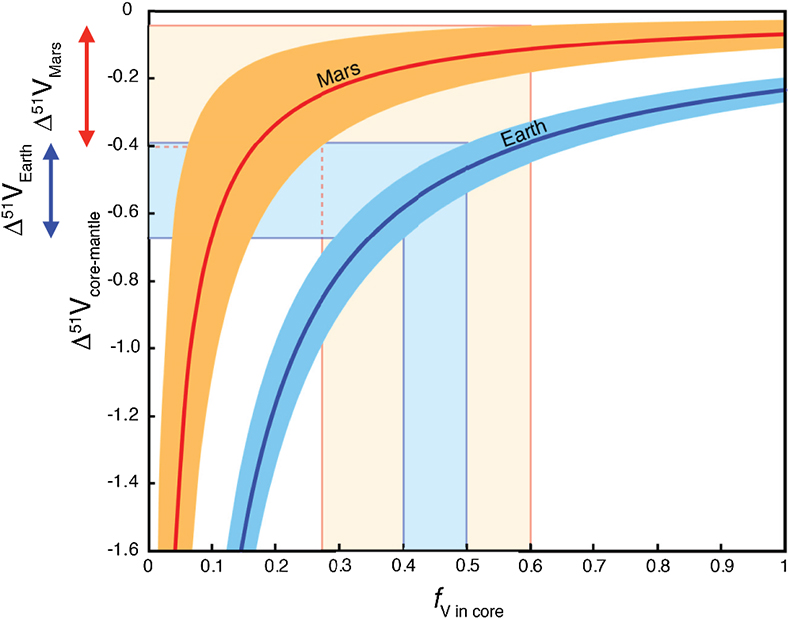
Figure 3 Modelled V isotope fractionation factors between the core and mantle of Earth (blue) and Mars (red) as a function of the fraction of total V that entered the core.