Emergence of peraluminous crustal magmas and implications for the early Earth
Affiliations | Corresponding Author | Cite as | Funding information- Share this article
-
Article views:822Cumulative count of HTML views and PDF downloads.
- Download Citation
- Rights & Permissions
top
Abstract

Figures
![]() Figure 1 (a) 207Pb/206Pb JH zircon age distributions from multiple units. (b) Ti in JH zircons with (c) Ti-in-zircon temperatures, calculated assuming aTiO2 = 0.5 and aSiO2 = 0.8 (Ferry and Watson, 2007) with zircons from the LFB (Trail et al., 2017). | ![]() Figure 2 (a) Age versus Al concentration for JH zircons, error bars are 2σ. Zircons with XZrcAl below detection limit (b.d.l.) are indicated at base of the diagram. Horizontal dashed line at 4 ppm Al defines zircons that likely formed from peraluminous melts. (b) Cumulative probability diagram of I- and S-type LFB (Trail et al., 2017) and age binned JH zircons. Numbers in inset are average XZrcAl for XZrcAl > 4 ppm zircon populations from each group. | ![]() Figure 3 (a) Histograms of P/Dy ratios and (b) scatterplot of P and Dy concentrations in zircons from I-type and S-type LFB granitoids compared with likely peraluminous (XZrcAl > 4 ppm) and non-peraluminous JH zircons. On the whole, peraluminous zircons from the JH have higher P/Dy ratios than non-peraluminous JH, and their median value of P/Dy is higher than that of peraluminous I-types. LFB data from (Burnham and Berry, 2017). | ![]() Figure 4 SiO2 versus ASI for common magmatic rocks and melts. LFB and Tuolumne Intrusive Suite (TIS) intrusive rocks binned in 1 wt. % SiO2 increments, error bars are 1σ. I-type granitic rocks from the LFB (Griffin et al., 1978; Chappell and White, 1992) and intrusive rocks from the TIS (Bateman et al., 1984; Ratajeski et al., 2001) become peraluminous at ∼70 wt. % SiO2, whereas hydrous basalts fractionated at depths ≥7 kbar can produce melts that become peraluminous at ∼60 wt. % SiO2, unlike those fractionated at lower pressures (Blatter et al., 2013). |
Figure 1 | Figure 2 | Figure 3 | Figure 4 |
top
Introduction
Continental crust and its derivatives (e.g., detrital zircons) preserve a near continuous record of Earth’s history from ∼4.4. Ga to today (Voice et al., 2011
Voice, P.J., Kowalewski, M., Eriksson, K.A. (2011) Quantifying the Timing and Rate of Crustal Evolution: Global Compilation of Radiometrically Dated Detrital Zircon Grains. The Journal of Geology 119, 109–126.
) that can be used to investigate both individual magmatic systems (e.g., Reimink et al., 2014Reimink, J.R., Chacko, T., Stern, R.A., Heaman, L.M. (2014) Earth’s earliest evolved crust generated in an Iceland-like setting. Nature Geoscience 7, 529–533.
) and secular changes in Earth’s geodynamic and tectonic history (Bauer et al., 2020Bauer, A.B., Reimink, J.R., Chacko, T., Foley, B.J., Shirey, S.B., Pearson, D.G. (2020) Hafnium isotopes in zircons document the gradual onset of mobile-lid tectonics. Geochemical Perspectives Letters 14, 1–6.
). The ∼3 Ga metasedimentary rocks from the JH in Western Australia contain detrital zircons that are the oldest known record of Earth’s continents (Compston and Pidgeon, 1986Compston, W., Pidgeon, R.T. (1986) Jack Hills, evidence of more very old detrital zircons in Western Australia. Nature 321, 766–769.
). These ancient zircons retain multiple chemical fingerprints that have been combined to reconstruct a Hadean Earth that bore oxidised, water-rich silicic continents derived from mafic (±felsic) protoliths that interacted with low temperature (T) surface waters (Cavosie et al., 2005Cavosie, A.J., Valley, J.W., Wilde, S.A., E.I.M.F. (2005) Magmatic δ18O in 4400–3900 Ma detrital zircons: A record of the alteration and recycling of crust in the Early Archean. Earth and Planetary Science Letters 235, 663–681.
; Watson and Harrison, 2005Watson, E.B., Harrison, T.M. (2005) Zircon Thermometer Reveals Minimum Melting Conditions on Earliest Earth. Science 308, 841–844.
; Trail et al., 2011Trail, D., Watson, E.B., Tailby, N.D. (2011) The oxidation state of Hadean magmas and implications for early Earth’s atmosphere. Nature 480, 79–82.
; Burnham and Berry, 2017Burnham, A.D., Berry, A.J. (2017) Formation of Hadean granites by melting of igneous crust. Nature Geoscience 10, 457–461.
).One outstanding problem in Earth’s early history is the nature and timing of the transition from a stagnant lid or vertical tectonic regime (crust thickened by processes akin to modern oceanic plateaus; Van Kranendonk et al., 2004
Van Kranendonk, M.J., Collins, W.J., Hickman, A., Pawley, M.J. (2004) Critical tests of vertical vs. horizontal tectonic models for the Archaean East Pilbara Granite–Greenstone Terrane, Pilbara Craton, Western Australia. Precambrian Research 131, 173–211.
; Reimink et al., 2014Reimink, J.R., Chacko, T., Stern, R.A., Heaman, L.M. (2014) Earth’s earliest evolved crust generated in an Iceland-like setting. Nature Geoscience 7, 529–533.
) to a mobile lid or horizontal tectonic regime where crust can be thickened and rapidly recycled through subduction-like processes and/or shallow thrusting (Bauer et al., 2020Bauer, A.B., Reimink, J.R., Chacko, T., Foley, B.J., Shirey, S.B., Pearson, D.G. (2020) Hafnium isotopes in zircons document the gradual onset of mobile-lid tectonics. Geochemical Perspectives Letters 14, 1–6.
). Horizontal tectonics is associated with an increase in the depth of melt generation. The shift toward a horizontal tectonic regime should produce chemical signatures in the ancient rock record that reflect this geodynamic shift. One potential tool to evaluate this shift is the Al content of zircon crystals (XZrcAl , in ppm by weight), which is strongly dependent on the peraluminosity of the melt from which the zircon crystallised (Wang and Trail, 2019Wang, Y., Trail, D. (2019) Aluminum partitioning between zircon and haplogranitic melts: The influence of temperature and melt composition. Chemical Geology 511, 71–80.
).Experiments and natural observations indicate that peraluminous melts (here defined as those with an aluminum saturation index [ASI = molar Al2O3/(CaO + Na2O + K2O)] > 1 (Shand, 1943
Shand, S.J. (1943) Eruptive rocks, their genesis, composition, and classification, with a chapter on meteorites. J. Wiley & Sons, Inc., New York.
) are the product of a limited number of petrogenetic processes. Here, we investigate XZrcAl from the JH as an indicator of peraluminous melts and relate this to petrogenetic models in the context of geodynamic shifts in the first ∼1.5 billion years of Earth’s history.top
Samples and Results
Zircons were collected from clastic metasedimentary units within the Jack Hills metamorphic belt (Weiss et al., 2015
Weiss, B.P. et al . (2015) Pervasive remagnetization of detrital zircon host rocks in the Jack Hills, Western Australia and implications for records of the early geodynamo. Earth and Planetary Science Letters 430, 115–128.
). All zircons were separated from their host rock and mounted in epoxy using previously described techniques (Trail et al., 2017Trail, D., Tailby, N., Wang, Y., Harrison, T.M., Boehnke, P. (2017) Aluminum in zircon as evidence for peraluminous and metaluminous melts from the Hadean to present. Geochemistry, Geophysics, Geosystems 18, 1580–1593.
; also see Supplementary Information). Care was taken to ensure the trace element compositions reported for zircons are primary structure bound signatures (e.g., not metamict regions, mineral inclusions, secondary alterations). Data were discarded if: 1) Fe > 100 ppm, 2) analyses outside 100 ± 10 % concordance, and/or 3) light rare earth element index (LREE-I) < 50 (Bell et al., 2019Bell, E.A., Boehnke, P., Barboni, M., Harrison, T.M. (2019) Tracking chemical alteration in magmatic zircon using rare earth element abundances. Chemical Geology 510, 56–71.
).The cumulative zircon age distribution is similar to previously reported ages from the JH (e.g., Crowley et al., 2005
Crowley, J.L., Myers, J.S., Sylvester, P.J., Cox, R.A. (2005) Detrital Zircon from the Jack Hills and Mount Narryer, Western Australia: Evidence for Diverse >4.0 Ga Source Rocks. The Journal of Geology 113, 239–263.
), with notable age peaks at 3370 and 4050 Ma (Fig. 1). Exceptionally, site T145 contained a high proportion (15.5 %) of unaltered Hadean-aged zircons. Zircon titanium concentrations are similar to other studies of the JH (e.g., Watson and Harrison, 2005Watson, E.B., Harrison, T.M. (2005) Zircon Thermometer Reveals Minimum Melting Conditions on Earliest Earth. Science 308, 841–844.
), and broadly indicate crystallisation from cool, water saturated intrusive igneous systems (Fig. 1). Age versus XZrcAl (Fig. 2) shows an increase in both the number of high Al (>4 ppm Al) and the average Al content of zircons beginning by ∼3.6 Ga (Fig. 2).
Figure 1 (a) 207Pb/206Pb JH zircon age distributions from multiple units. (b) Ti in JH zircons with (c) Ti-in-zircon temperatures, calculated assuming aTiO2 = 0.5 and aSiO2 = 0.8 (Ferry and Watson, 2007
Ferry, J.M., Watson, E.B. (2007) New thermodynamic models and revised calibrations for the Ti-in-zircon and Zr-in-rutile thermometers. Contributions to Mineralogy and Petrology 154, 429–437.
) with zircons from the LFB (Trail et al., 2017Trail, D., Tailby, N., Wang, Y., Harrison, T.M., Boehnke, P. (2017) Aluminum in zircon as evidence for peraluminous and metaluminous melts from the Hadean to present. Geochemistry, Geophysics, Geosystems 18, 1580–1593.
).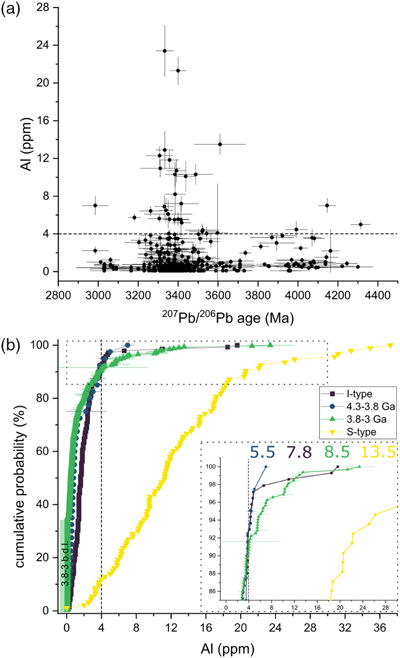
Figure 2 (a) Age versus Al concentration for JH zircons, error bars are 2σ. Zircons with XZrcAl below detection limit (b.d.l.) are indicated at base of the diagram. Horizontal dashed line at 4 ppm Al defines zircons that likely formed from peraluminous melts. (b) Cumulative probability diagram of I- and S-type LFB (Trail et al., 2017
Trail, D., Tailby, N., Wang, Y., Harrison, T.M., Boehnke, P. (2017) Aluminum in zircon as evidence for peraluminous and metaluminous melts from the Hadean to present. Geochemistry, Geophysics, Geosystems 18, 1580–1593.
) and age binned JH zircons. Numbers in inset are average XZrcAl for XZrcAl > 4 ppm zircon populations from each group.top
Discussion
Al-in-zircon as a proxy for peraluminosity. XZrcAl is influenced by melt composition, temperature and water content (Al3+ + H+ = Si4+) (Trail et al., 2017
Trail, D., Tailby, N., Wang, Y., Harrison, T.M., Boehnke, P. (2017) Aluminum in zircon as evidence for peraluminous and metaluminous melts from the Hadean to present. Geochemistry, Geophysics, Geosystems 18, 1580–1593.
; Wang and Trail, 2019Wang, Y., Trail, D. (2019) Aluminum partitioning between zircon and haplogranitic melts: The influence of temperature and melt composition. Chemical Geology 511, 71–80.
). These parameters will co-vary in a magmatic system, and a magmatic liquid cooling along a liquid line of descent will generally increase peraluminosity and water content with decreasing temperature (e.g., Blatter et al., 2013Blatter, D.L., Sisson, T.W., Hankins, W.B. (2013) Crystallization of oxidized, moderately hydrous arc basalt at mid- to lower-crustal pressures: implications for andesite genesis. Contributions to Mineralogy and Petrology 166, 861–886.
). However, JH zircons record a narrow range of temperatures (Fig. 1). These temperatures indicate crystallisation from a cool, water-rich silicic magma which suggests that variations in water activity play a subordinate role in regulating XZrcAl . The weak correlation between Al and Ti (Fig. S-5) suggests T also plays a subordinate role to composition in regulating XZrcAl within this population (Trail et al., 2017Trail, D., Tailby, N., Wang, Y., Harrison, T.M., Boehnke, P. (2017) Aluminum in zircon as evidence for peraluminous and metaluminous melts from the Hadean to present. Geochemistry, Geophysics, Geosystems 18, 1580–1593.
). The dominant role of composition is further demonstrated in granitic zircons from the Lachlan Fold Belt (LFB), the type locality of I- and S-type granitic rocks [defined here as granitic rocks known to primarily form through igneous processes (I-type) versus those formed to a measurable extent by melting of metasedimentary precursors (S-types); e.g., Chappell, 1999Chappell, B.W. (1999) Aluminium saturation in I- and S-type granites and the characterization of fractionated haplogranites. Lithos 46, 535–551.
]. It should be noted that I-type magmas are often weakly peraluminous (ASI < 1.1), and as such bulk peraluminosity is not used here to define I- versus S-type magmas. In the LFB, even though Ti content of I-type zircons is slightly higher on average than S-types, the latter have significantly higher XZrcAl (Fig. S-4).Increasing peraluminosity results in increased alumina activity and a subsequent increase in XZrcAl . For granitic zircons of the LFB, XZrcAl exceeds 4 ppm when the bulk composition becomes peraluminous, likely reflecting this increase in alumina activity in the melt (Trail et al., 2017
Trail, D., Tailby, N., Wang, Y., Harrison, T.M., Boehnke, P. (2017) Aluminum in zircon as evidence for peraluminous and metaluminous melts from the Hadean to present. Geochemistry, Geophysics, Geosystems 18, 1580–1593.
). We use this 4 ppm value to discriminate between zircons that likely crystallised from peraluminous melts. It is worth noting that ∼80 % of JH zircons have Al contents lower than the LFB I-types (Fig. 2b), which reflects the lower crystallisation T observed in the Ti content (Fig. 1c). Thus, this LFB based peraluminosity threshold likely underestimates the number of peraluminous JH zircons.In the JH, there is a subtle but significant increase in XZrcAl with decreasing age (Fig. 2b). The >3.8 Ga population yields XZrcAl similar to I-type granitoids. In the 3.8–3 Ga population, likely peraluminous zircons (>4 ppm Al) have an average XZrcAl = 8.5 ppm compared to XZrcAl = 5.5 ppm in the >3.8 Ga group and XZrcAl = 7.8 ppm in I-types. As the JH is a metasedimentary rock, this increase is akin to sedimentary mixing of metaluminous [ASI < 1, molar Al2O3/(Na2O + K2O) > 1] and peraluminous protoliths seen in modern sedimentary systems (Fig. S-7).
Origin of peraluminous zircons . The limited number of mechanisms to generate peraluminous melts makes their appearance in the JH record a useful event for interpreting tectonic shifts on the early Earth. Experiments and investigations of modern magmatic systems have demonstrated multiple mechanisms to produce peraluminous magmas, including: (1) late stage differentiation of silicic “I-type” granitic magmas, (2) melting and incorporation of metasedimentary material, and (3) partial melting or fractionation of hydrous mafic parent material at >7 kbar.
Late stage differentiation of metaluminous I-type bulk compositions can produce weakly peraluminous melts. However, this peraluminosity yields a negligible amount of zircon (Trail et al., 2017
Trail, D., Tailby, N., Wang, Y., Harrison, T.M., Boehnke, P. (2017) Aluminum in zircon as evidence for peraluminous and metaluminous melts from the Hadean to present. Geochemistry, Geophysics, Geosystems 18, 1580–1593.
). Similarly, I-type systems can also evolve toward weakly peraluminous bulk compositions (Chappell, 1999Chappell, B.W. (1999) Aluminium saturation in I- and S-type granites and the characterization of fractionated haplogranites. Lithos 46, 535–551.
and sources therein), but peraluminous I-type zircons have similar compositions to their metaluminous counterparts (Fig. 3).
Figure 3 (a) Histograms of P/Dy ratios and (b) scatterplot of P and Dy concentrations in zircons from I-type and S-type LFB granitoids compared with likely peraluminous (XZrcAl > 4 ppm) and non-peraluminous JH zircons. On the whole, peraluminous zircons from the JH have higher P/Dy ratios than non-peraluminous JH, and their median value of P/Dy is higher than that of peraluminous I-types. LFB data from (Burnham and Berry, 2017
Burnham, A.D., Berry, A.J. (2017) Formation of Hadean granites by melting of igneous crust. Nature Geoscience 10, 457–461.
).On Earth today, the most volumetrically significant way to produce strongly peraluminous melts is through melting of weathered sediments (e.g., psammites, metapelites) (Patiño Douce and Johnston, 1991
Patiño Douce, A.E., Johnston, A.D. (1991) Phase equilibria and melt productivity in the pelitic system: implications for the origin of peraluminous granitoids and aluminous granulites. Contributions to Mineralogy and Petrology 107, 202–218.
). Based on a P versus REE (or Dy) discrimination diagram that records the increase in P content of apatite saturated peraluminous melts, zircons from the Jack Hills have been dismissed as being derived from peraluminous melts (Burnham and Berry, 2017Burnham, A.D., Berry, A.J. (2017) Formation of Hadean granites by melting of igneous crust. Nature Geoscience 10, 457–461.
). However, there is significant overlap at low P content between peraluminous S-type and metaluminous and weakly peraluminous I-type granitoids (Fig. 3). Although the JH zircons do not exhibit high P-Dy signals, the overlap at low P content between S-type and I-type zircons makes it difficult to rule out metasediments as a source of some JH zircons, and does not discount previous work suggesting a potential sedimentary source for some JH zircons (Bell et al., 2015Bell, E.A., Boehnke, P., Harrison, T.M., Mao, W.L. (2015) Potentially biogenic carbon preserved in a 4.1 billion-year-old zircon. Proceedings of the National Academy of Sciences 112, 14518–14521.
).In lieu of metasediment melting, moderate pressure fractionation of hydrous mafic material could produce peraluminous zircons in excess of those found in modern I-type granitoids. At pressures greater than ∼7 kbar, hydrous mafic magmas can produce alumina-rich melts via crystallisation of Ca-rich pyroxene and suppression of plagioclase crystallisation (Müntener and Ulmer, 2006
Müntener, O., Ulmer, P. (2006) Experimentally derived high-pressure cumulates from hydrous arc magmas and consequences for the seismic velocity structure of lower arc crust. Geophysical Research Letters 33, L21308.
; Blatter et al., 2013Blatter, D.L., Sisson, T.W., Hankins, W.B. (2013) Crystallization of oxidized, moderately hydrous arc basalt at mid- to lower-crustal pressures: implications for andesite genesis. Contributions to Mineralogy and Petrology 166, 861–886.
), and at pressures within the garnet stability field (≥10 kbar), incongruent melting of amphibole and biotite in metaluminous bulk compositions can produce alumina-rich melts and pyroxene (Chappell et al., 2012Chappell, B.W., Bryant, C.J., Wyborn, D. (2012) Peraluminous I-type granites. Lithos 153, 142–153.
). These moderate pressure processes lead to the generation of peraluminous melts at lower bulk SiO2 (∼60 wt. % SiO2 versus ∼70 wt. % SiO2) than modern arcs magmas (Fig. 4), which in turn could yield a higher proportion of peraluminous zircons than modern arcs.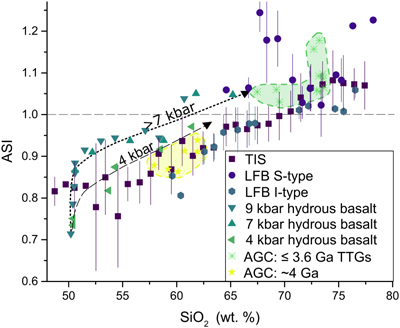
Figure 4 SiO2 versus ASI for common magmatic rocks and melts. LFB and Tuolumne Intrusive Suite (TIS) intrusive rocks binned in 1 wt. % SiO2 increments, error bars are 1σ. I-type granitic rocks from the LFB (Griffin et al., 1978
Griffin, T.J., White, A.J.R., Chappell, B.W. (1978) The Moruya Batholith and geochemical contrasts between the Moruya and Jindabyne suites. Journal of the Geological Society of Australia 25, 235–247.
; Chappell and White, 1992Chappell, B.W., White, A.J.R. (1992) I- and S-type granites in the Lachlan Fold Belt. Earth and Environmental Science Transactions of the Royal Society of Edinburgh 83, 1–26.
) and intrusive rocks from the TIS (Bateman et al., 1984Bateman, P.C., Dodge, F.C.W., Bruggman, P.E. (1984) Major oxide analyses, CIPW norms, modes, and bulk specific gravities of plutonic rocks from the Mariposa 1° × 2° sheet, central Sierra Nevada, California. US Geological Survey Open-File Report 84–162.
; Ratajeski et al., 2001Ratajeski, K., Glazner, A.F., Miller, B.V. (2001) Geology and geochemistry of mafic to felsic plutonic rocks in the Cretaceous intrusive suite of Yosemite Valley, California. Geological Society of America Bulletin 113, 1486–1502.
) become peraluminous at ∼70 wt. % SiO2, whereas hydrous basalts fractionated at depths ≥7 kbar can produce melts that become peraluminous at ∼60 wt. % SiO2, unlike those fractionated at lower pressures (Blatter et al., 2013Blatter, D.L., Sisson, T.W., Hankins, W.B. (2013) Crystallization of oxidized, moderately hydrous arc basalt at mid- to lower-crustal pressures: implications for andesite genesis. Contributions to Mineralogy and Petrology 166, 861–886.
).Implications for early Earth geodynamics . The distribution of XZrcAl of JH zircons >3.8 Ga is similar to modern I-type magmas (Fig. 2), whose intermediate compositions overlap in SiO2-ASI space with >4 Ga granitoids from the Acasta Gneiss Complex (AGC) that have been interpreted as crystallising from an environment akin to modern oceanic plateaus (Reimink et al., 2014
Reimink, J.R., Chacko, T., Stern, R.A., Heaman, L.M. (2014) Earth’s earliest evolved crust generated in an Iceland-like setting. Nature Geoscience 7, 529–533.
) at 3–4 kbar (Fig. 4). On the ancient Earth, this is consistent with crystallisation in a vertical tectonic regime prior to 3.8 Ga, and is further supported by other chemical similarities between pre-4 Ga AGC and JH zircons (Reimink et al., 2019aReimink, J.R., Davies, J.H.F.L., Bauer, A.M., Chacko, T. (2019a) A comparison between zircons from the Acasta Gneiss Complex and the Jack Hills region. Earth and Planetary Science Letters 531, 115975.
).Pre- and post-3.8 Ga JH and I-type populations cross the 4 ppm Al threshold at roughly the same cumulative probability (∼90 %). However, the average Al content of the likely peraluminous post-3.8 Ga zircons is greater than either the pre-3.8 or I-type populations. This potentially indicates a different and strongly peraluminous source to the 3.8–3 Ga group. Regardless of whether the peraluminous zircons are derived from metasedimentary sources or moderate high pressure fractionation processes, both scenarios likely require a geodynamic shift toward horizontal tectonics. For sediment melting, weathered continental material is required to be brought to depths great enough to be melted. For deep mafic melting, hydrated near surface mafic material needs to be brought to moderate pressures (≥7 kbar) in order to melt and fractionate. Although it is possible for hydrated mafic material to be brought to depths through vertical tectonic processes, the relative short timescales invoked to account for the shift in ɛ176Hf observed from JH and other ancient zircons (Bauer et al., 2020
Bauer, A.B., Reimink, J.R., Chacko, T., Foley, B.J., Shirey, S.B., Pearson, D.G. (2020) Hafnium isotopes in zircons document the gradual onset of mobile-lid tectonics. Geochemical Perspectives Letters 14, 1–6.
), similarities in trace element content between JH and AGC zircons (Reimink et al., 2019aReimink, J.R., Davies, J.H.F.L., Bauer, A.M., Chacko, T. (2019a) A comparison between zircons from the Acasta Gneiss Complex and the Jack Hills region. Earth and Planetary Science Letters 531, 115975.
), and the onset of peraluminosity indicate a shift toward a horizontal tectonic regime.Whereas peraluminous granitic rocks dominate the bedrock geology in the AGC at ∼3.6 Ga (Reimink et al., 2019b
Reimink, J.R., Pearson, D.G., Shirey, S.B., Carlson, R.W., Ketchum, J.W.F. (2019b) Onset of new, progressive crustal growth in the central Slave craton at 3.55 Ga. Geochemical Perspectives Letters 10, 8–13.
), the peraluminous zircons that emerge in the JH record at ∼3.6 Ga are only a fraction of the total zircon population (∼10 %). This could indicate preservation bias of the peraluminous rocks of the AGC, preservation of more diverse protoliths in the detrital JH zircons, or a globally heterogeneous distribution of crust-forming processes ca. 3–3.6 Ga. Regardless, the emergence of significant peraluminous zircons in the JH conforms with observations from other ancient crustal systems (e.g., AGC, Pilbara Craton; Bauer et al., 2020Bauer, A.B., Reimink, J.R., Chacko, T., Foley, B.J., Shirey, S.B., Pearson, D.G. (2020) Hafnium isotopes in zircons document the gradual onset of mobile-lid tectonics. Geochemical Perspectives Letters 14, 1–6.
) of a geodynamic shift toward horizontal tectonics by at least ∼3.6 Ga.top
Acknowledgements
We thank J. Reimink, R. Fu, A.C. Maloof, N.D. Tailby, and B.P. Weiss for scientific discussions and T. Freeman, A. Sharma, J.P. Hurtado Padilla and the SI YES! Internship programme for imaging zircons. This work partially supported by NASA PC3 grant 80NSSC19M0069.
Editor: Maud Boyet
top
References
Bateman, P.C., Dodge, F.C.W., Bruggman, P.E. (1984) Major oxide analyses, CIPW norms, modes, and bulk specific gravities of plutonic rocks from the Mariposa 1° × 2° sheet, central Sierra Nevada, California. US Geological Survey Open-File Report 84–162.

I-type granitic rocks from the LFB (Griffin et al., 1978; Chappell and White, 1992) and intrusive rocks from the TIS (Bateman et al., 1984; Ratajeski et al., 2001) become peraluminous at ∼70 wt. % SiO2, whereas hydrous basalts fractionated at depths ≥7 kbar can produce melts that become peraluminous at ∼60 wt. % SiO2, unlike those fractionated at lower pressures (Blatter et al., 2013).
View in article
Bauer, A.B., Reimink, J.R., Chacko, T., Foley, B.J., Shirey, S.B., Pearson, D.G. (2020) Hafnium isotopes in zircons document the gradual onset of mobile-lid tectonics. Geochemical Perspectives Letters 14, 1–6.

Ga to today (Voice et al., 2011) that can be used to investigate both individual magmatic systems (e.g., Reimink et al., 2014) and secular changes in Earth’s geodynamic and tectonic history (Bauer et al., 2020).
View in article
Although it is possible for hydrated mafic material to be brought to depths through vertical tectonic processes, the relative short timescales invoked to account for the shift in ɛ176Hf observed from JH and other ancient zircons (Bauer et al., 2020), similarities in trace element content between JH and AGC zircons (Reimink et al., 2019a), and the onset of peraluminosity indicate a shift toward a horizontal tectonic regime.
View in article
Regardless, the emergence of significant peraluminous zircons in the JH conforms with observations from other ancient crustal systems (e.g., AGC, Pilbara Craton; Bauer et al., 2020) of a geodynamic shift toward horizontal tectonics by at least ∼3.6 Ga.
View in article
One outstanding problem in Earth’s early history is the nature and timing of the transition from a stagnant lid or vertical tectonic regime (crust thickened by processes akin to modern oceanic plateaus; Van Kranendonk et al., 2004; Reimink et al., 2014) to a mobile lid or horizontal tectonic regime where crust can be thickened and rapidly recycled through subduction-like processes and/or shallow thrusting (Bauer et al., 2020).
View in article
Bell, E.A., Boehnke, P., Harrison, T.M., Mao, W.L. (2015) Potentially biogenic carbon preserved in a 4.1 billion-year-old zircon. Proceedings of the National Academy of Sciences 112, 14518–14521.

Although the JH zircons do not exhibit high P-Dy signals, the overlap at low P content between S-type and I-type zircons makes it difficult to rule out metasediments as a source of some JH zircons, and does not discount previous work suggesting a potential sedimentary source for some JH zircons (Bell et al., 2015).
View in article
Bell, E.A., Boehnke, P., Barboni, M., Harrison, T.M. (2019) Tracking chemical alteration in magmatic zircon using rare earth element abundances. Chemical Geology 510, 56–71.

Data were discarded if: 1) Fe > 100 ppm, 2) analyses outside 100 ± 10 % concordance, and/or 3) light rare earth element index (LREE-I) < 50 (Bell et al., 2019).
View in article
Blatter, D.L., Sisson, T.W., Hankins, W.B. (2013) Crystallization of oxidized, moderately hydrous arc basalt at mid- to lower-crustal pressures: implications for andesite genesis. Contributions to Mineralogy and Petrology 166, 861–886.

These parameters will co-vary in a magmatic system, and a magmatic liquid cooling along a liquid line of descent will generally increase peraluminosity and water content with decreasing temperature (e.g., Blatter et al., 2013).
View in article
At pressures greater than ∼7 kbar, hydrous mafic magmas can produce alumina-rich melts via crystallisation of Ca-rich pyroxene and suppression of plagioclase crystallisation (Müntener and Ulmer, 2006; Blatter et al., 2013), and at pressures within the garnet stability field (≥10 kbar), incongruent melting of amphibole and biotite in metaluminous bulk compositions can produce alumina-rich melts and pyroxene (Chappell et al., 2012).
View in article
I-type granitic rocks from the LFB (Griffin et al., 1978; Chappell and White, 1992) and intrusive rocks from the TIS (Bateman et al., 1984; Ratajeski et al., 2001) become peraluminous at ∼70 wt. % SiO2, whereas hydrous basalts fractionated at depths ≥7 kbar can produce melts that become peraluminous at ∼60 wt. % SiO2, unlike those fractionated at lower pressures (Blatter et al., 2013).
View in article
Burnham, A.D., Berry, A.J. (2017) Formation of Hadean granites by melting of igneous crust. Nature Geoscience 10, 457–461.

LFB data from (Burnham and Berry, 2017).
View in article
Based on a P versus REE (or Dy) discrimination diagram that records the increase in P content of apatite saturated peraluminous melts, zircons from the Jack Hills have been dismissed as being derived from peraluminous melts (Burnham and Berry, 2017).
View in article
These ancient zircons retain multiple chemical fingerprints that have been combined to reconstruct a Hadean Earth that bore oxidised, water-rich silicic continents derived from mafic (±felsic) protoliths that interacted with low temperature (T) surface waters (Cavosie et al., 2005; Watson and Harrison, 2005; Trail et al., 2011; Burnham and Berry, 2017).
View in article
Cavosie, A.J., Valley, J.W., Wilde, S.A., E.I.M.F. (2005) Magmatic δ18O in 4400–3900 Ma detrital zircons: A record of the alteration and recycling of crust in the Early Archean. Earth and Planetary Science Letters 235, 663–681.

These ancient zircons retain multiple chemical fingerprints that have been combined to reconstruct a Hadean Earth that bore oxidised, water-rich silicic continents derived from mafic (±felsic) protoliths that interacted with low temperature (T) surface waters (Cavosie et al., 2005; Watson and Harrison, 2005; Trail et al., 2011; Burnham and Berry, 2017).
View in article
Chappell, B.W. (1999) Aluminium saturation in I- and S-type granites and the characterization of fractionated haplogranites. Lithos 46, 535–551.

The dominant role of composition is further demonstrated in granitic zircons from the Lachlan Fold Belt (LFB), the type locality of I- and S-type granitic rocks [defined here as granitic rocks known to primarily form through igneous processes (I-type) versus those formed to a measurable extent by melting of metasedimentary precursors (S-types); e.g., Chappell, 1999]
View in article
Similarly, I-type systems can also evolve toward weakly peraluminous bulk compositions (Chappell, 1999 and sources therein), but peraluminous I-type zircons have similar compositions to their metaluminous counterparts (Fig. 3).
View in article
Chappell, B.W., White, A.J.R. (1992) I- and S-type granites in the Lachlan Fold Belt. Earth and Environmental Science Transactions of the Royal Society of Edinburgh 83, 1–26.

I-type granitic rocks from the LFB (Griffin et al., 1978; Chappell and White, 1992) and intrusive rocks from the TIS (Bateman et al., 1984; Ratajeski et al., 2001) become peraluminous at ∼70 wt. % SiO2, whereas hydrous basalts fractionated at depths ≥7 kbar can produce melts that become peraluminous at ∼60 wt. % SiO2, unlike those fractionated at lower pressures (Blatter et al., 2013).
View in article
Chappell, B.W., Bryant, C.J., Wyborn, D. (2012) Peraluminous I-type granites. Lithos 153, 142–153.

At pressures greater than ∼7 kbar, hydrous mafic magmas can produce alumina-rich melts via crystallisation of Ca-rich pyroxene and suppression of plagioclase crystallisation (Müntener and Ulmer, 2006; Blatter et al., 2013), and at pressures within the garnet stability field (≥10 kbar), incongruent melting of amphibole and biotite in metaluminous bulk compositions can produce alumina-rich melts and pyroxene (Chappell et al., 2012).
View in article
Compston, W., Pidgeon, R.T. (1986) Jack Hills, evidence of more very old detrital zircons in Western Australia. Nature 321, 766–769.

The ∼3 Ga metasedimentary rocks from the JH in Western Australia contain detrital zircons that are the oldest known record of Earth’s continents (Compston and Pidgeon, 1986).
View in article
Crowley, J.L., Myers, J.S., Sylvester, P.J., Cox, R.A. (2005) Detrital Zircon from the Jack Hills and Mount Narryer, Western Australia: Evidence for Diverse >4.0 Ga Source Rocks. The Journal of Geology 113, 239–263.

The cumulative zircon age distribution is similar to previously reported ages from the JH (e.g., Crowley et al., 2005), with notable age peaks at 3370 and 4050 Ma (Fig. 1).
View in article
Ferry, J.M., Watson, E.B. (2007) New thermodynamic models and revised calibrations for the Ti-in-zircon and Zr-in-rutile thermometers. Contributions to Mineralogy and Petrology 154, 429–437.

(c) Ti-in-zircon temperatures, calculated assuming aTiO2 = 0.5 and aSiO2 = 0.8 (Ferry and Watson, 2007) with zircons from the LFB (Trail et al., 2017).
View in article
Griffin, T.J., White, A.J.R., Chappell, B.W. (1978) The Moruya Batholith and geochemical contrasts between the Moruya and Jindabyne suites. Journal of the Geological Society of Australia 25, 235–247.

I-type granitic rocks from the LFB (Griffin et al., 1978; Chappell and White, 1992) and intrusive rocks from the TIS (Bateman et al., 1984; Ratajeski et al., 2001) become peraluminous at ∼70 wt. % SiO2, whereas hydrous basalts fractionated at depths ≥7 kbar can produce melts that become peraluminous at ∼60 wt. % SiO2, unlike those fractionated at lower pressures (Blatter et al., 2013).
View in article
Müntener, O., Ulmer, P. (2006) Experimentally derived high-pressure cumulates from hydrous arc magmas and consequences for the seismic velocity structure of lower arc crust. Geophysical Research Letters 33, L21308.

At pressures greater than ∼7 kbar, hydrous mafic magmas can produce alumina-rich melts via crystallisation of Ca-rich pyroxene and suppression of plagioclase crystallisation (Müntener and Ulmer, 2006; Blatter et al., 2013), and at pressures within the garnet stability field (≥10 kbar), incongruent melting of amphibole and biotite in metaluminous bulk compositions can produce alumina-rich melts and pyroxene (Chappell et al., 2012).
View in article
Patiño Douce, A.E., Johnston, A.D. (1991) Phase equilibria and melt productivity in the pelitic system: implications for the origin of peraluminous granitoids and aluminous granulites. Contributions to Mineralogy and Petrology 107, 202–218.

On Earth today, the most volumetrically significant way to produce strongly peraluminous melts is through melting of weathered sediments (e.g., psammites, metapelites) (Patiño Douce and Johnston, 1991).
View in article
Ratajeski, K., Glazner, A.F., Miller, B.V. (2001) Geology and geochemistry of mafic to felsic plutonic rocks in the Cretaceous intrusive suite of Yosemite Valley, California. Geological Society of America Bulletin 113, 1486–1502.

I-type granitic rocks from the LFB (Griffin et al., 1978; Chappell and White, 1992) and intrusive rocks from the TIS (Bateman et al., 1984; Ratajeski et al., 2001) become peraluminous at ∼70 wt. % SiO2, whereas hydrous basalts fractionated at depths ≥7 kbar can produce melts that become peraluminous at ∼60 wt. % SiO2, unlike those fractionated at lower pressures (Blatter et al., 2013).
View in article
Reimink, J.R., Chacko, T., Stern, R.A., Heaman, L.M. (2014) Earth’s earliest evolved crust generated in an Iceland-like setting. Nature Geoscience 7, 529–533.

Ga to today (Voice et al., 2011) that can be used to investigate both individual magmatic systems (e.g., Reimink et al., 2014) and secular changes in Earth’s geodynamic and tectonic history (Bauer et al., 2020).
View in article
The distribution of XZrcAl of JH zircons >3.8 Ga is similar to modern I-type magmas (Fig. 2), whose intermediate compositions overlap in SiO2-ASI space with >4 Ga granitoids from the Acasta Gneiss Complex (AGC) that have been interpreted as crystallising from an environment akin to modern oceanic plateaus (Reimink et al., 2014) at 3–4 kbar (Fig. 4).
View in article
One outstanding problem in Earth’s early history is the nature and timing of the transition from a stagnant lid or vertical tectonic regime (crust thickened by processes akin to modern oceanic plateaus; Van Kranendonk et al., 2004; Reimink et al., 2014) to a mobile lid or horizontal tectonic regime where crust can be thickened and rapidly recycled through subduction-like processes and/or shallow thrusting (Bauer et al., 2020).
View in article
Reimink, J.R., Davies, J.H.F.L., Bauer, A.M., Chacko, T. (2019a) A comparison between zircons from the Acasta Gneiss Complex and the Jack Hills region. Earth and Planetary Science Letters 531, 115975.

On the ancient Earth, this is consistent with crystallisation in a vertical tectonic regime prior to 3.8 Ga, and is further supported by other chemical similarities between pre-4 Ga AGC and JH zircons (Reimink et al., 2019a).
View in article
Although it is possible for hydrated mafic material to be brought to depths through vertical tectonic processes, the relative short timescales invoked to account for the shift in ɛ176Hf observed from JH and other ancient zircons (Bauer et al., 2020), similarities in trace element content between JH and AGC zircons (Reimink et al., 2019a), and the onset of peraluminosity indicate a shift toward a horizontal tectonic regime.
View in article
Reimink, J.R., Pearson, D.G., Shirey, S.B., Carlson, R.W., Ketchum, J.W.F. (2019b) Onset of new, progressive crustal growth in the central Slave craton at 3.55 Ga. Geochemical Perspectives Letters 10, 8–13.

Whereas peraluminous granitic rocks dominate the bedrock geology in the AGC at ∼3.6 Ga (Reimink et al., 2019b), the peraluminous zircons that emerge in the JH record at ∼3.6 Ga are only a fraction of the total zircon population (∼10 %).
View in article
Shand, S.J. (1943) Eruptive rocks, their genesis, composition, and classification, with a chapter on meteorites. J. Wiley & Sons, Inc., New York.

Experiments and natural observations indicate that peraluminous melts (here defined as those with an aluminum saturation index [ASI = molar Al2O3/(CaO + Na2O + K2O)] > 1 (Shand, 1943) are the product of a limited number of petrogenetic processes. Here, we investigate XZrcAl from the JH as an indicator of peraluminous melts and relate this to petrogenetic models in the context of geodynamic shifts in the first ∼1.5 billion years of Earth’s history.
View in article
Trail, D., Watson, E.B., Tailby, N.D. (2011) The oxidation state of Hadean magmas and implications for early Earth’s atmosphere. Nature 480, 79–82.

These ancient zircons retain multiple chemical fingerprints that have been combined to reconstruct a Hadean Earth that bore oxidised, water-rich silicic continents derived from mafic (±felsic) protoliths that interacted with low temperature (T) surface waters (Cavosie et al., 2005; Watson and Harrison, 2005; Trail et al., 2011; Burnham and Berry, 2017).
View in article
Trail, D., Tailby, N., Wang, Y., Harrison, T.M., Boehnke, P. (2017) Aluminum in zircon as evidence for peraluminous and metaluminous melts from the Hadean to present. Geochemistry, Geophysics, Geosystems 18, 1580–1593.

All zircons were separated from their host rock and mounted in epoxy using previously described techniques (Trail et al., 2017; also see Supplementary Information).
View in article
(b) Cumulative probability diagram of I- and S-type LFB (Trail et al., 2017) and age binned JH zircons. Numbers in inset are average XZrcAl for XZrcAl > 4 ppm zircon populations from each group.
View in article
XZrcAl is influenced by melt composition, temperature and water content (Al3+ + H+ = Si4+) (Trail et al., 2017; Wang and Trail, 2019).
View in article
The weak correlation between Al and Ti (Fig. S-5) suggests T also plays a subordinate role to composition in regulating XZrcAl within this population (Trail et al., 2017).
View in article
For granitic zircons of the LFB, XZrcAl exceeds 4 ppm when the bulk composition becomes peraluminous, likely reflecting this increase in alumina activity in the melt (Trail et al., 2017).
View in article
However, this peraluminosity yields a negligible amount of zircon (Trail et al., 2017).
View in article
(c) Ti-in-zircon temperatures, calculated assuming aTiO2 = 0.5 and aSiO2 = 0.8 (Ferry and Watson, 2007) with zircons from the LFB (Trail et al., 2017).
View in article
Van Kranendonk, M.J., Collins, W.J., Hickman, A., Pawley, M.J. (2004) Critical tests of vertical vs. horizontal tectonic models for the Archaean East Pilbara Granite–Greenstone Terrane, Pilbara Craton, Western Australia. Precambrian Research 131, 173–211.

One outstanding problem in Earth’s early history is the nature and timing of the transition from a stagnant lid or vertical tectonic regime (crust thickened by processes akin to modern oceanic plateaus; Van Kranendonk et al., 2004; Reimink et al., 2014) to a mobile lid or horizontal tectonic regime where crust can be thickened and rapidly recycled through subduction-like processes and/or shallow thrusting (Bauer et al., 2020).
View in article
Voice, P.J., Kowalewski, M., Eriksson, K.A. (2011) Quantifying the Timing and Rate of Crustal Evolution: Global Compilation of Radiometrically Dated Detrital Zircon Grains. The Journal of Geology 119, 109–126.

Ga to today (Voice et al., 2011) that can be used to investigate both individual magmatic systems (e.g., Reimink et al., 2014) and secular changes in Earth’s geodynamic and tectonic history (Bauer et al., 2020).
View in article
Wang, Y., Trail, D. (2019) Aluminum partitioning between zircon and haplogranitic melts: The influence of temperature and melt composition. Chemical Geology 511, 71–80.

One potential tool to evaluate this shift is the Al content of zircon crystals (XZrcAl , in ppm by weight), which is strongly dependent on the peraluminosity of the melt from which the zircon crystallised (Wang and Trail, 2019).
View in article
XZrcAl is influenced by melt composition, temperature and water content (Al3+ + H+ = Si4+) (Trail et al., 2017; Wang and Trail, 2019).
View in article
Watson, E.B., Harrison, T.M. (2005) Zircon Thermometer Reveals Minimum Melting Conditions on Earliest Earth. Science 308, 841–844.

Zircon titanium concentrations are similar to other studies of the JH (e.g., Watson and Harrison, 2005), and broadly indicate crystallisation from cool, water saturated intrusive igneous systems (Fig. 1). Age versus XZrcAl (Fig. 2) shows an increase in both the number of high Al (>4 ppm Al) and the average Al content of zircons beginning by ∼3.6 Ga (Fig. 2).
View in article
These ancient zircons retain multiple chemical fingerprints that have been combined to reconstruct a Hadean Earth that bore oxidised, water-rich silicic continents derived from mafic (±felsic) protoliths that interacted with low temperature (T) surface waters (Cavosie et al., 2005; Watson and Harrison, 2005; Trail et al., 2011; Burnham and Berry, 2017).
View in article
Weiss, B.P. et al . (2015) Pervasive remagnetization of detrital zircon host rocks in the Jack Hills, Western Australia and implications for records of the early geodynamo. Earth and Planetary Science Letters 430, 115–128.

Zircons were collected from clastic metasedimentary units within the Jack Hills metamorphic belt (Weiss et al., 2015).
View in article
top
Supplementary Information
The Supplementary Information includes:
Download Table S-2 (Excel).
Download the Supplementary Information (PDF).
Figures

Figure 1 (a) 207Pb/206Pb JH zircon age distributions from multiple units. (b) Ti in JH zircons with (c) Ti-in-zircon temperatures, calculated assuming aTiO2 = 0.5 and aSiO2 = 0.8 (Ferry and Watson, 2007
Ferry, J.M., Watson, E.B. (2007) New thermodynamic models and revised calibrations for the Ti-in-zircon and Zr-in-rutile thermometers. Contributions to Mineralogy and Petrology 154, 429–437.
) with zircons from the LFB (Trail et al., 2017Trail, D., Tailby, N., Wang, Y., Harrison, T.M., Boehnke, P. (2017) Aluminum in zircon as evidence for peraluminous and metaluminous melts from the Hadean to present. Geochemistry, Geophysics, Geosystems 18, 1580–1593.
).
Figure 2 (a) Age versus Al concentration for JH zircons, error bars are 2σ. Zircons with XZrcAl below detection limit (b.d.l.) are indicated at base of the diagram. Horizontal dashed line at 4 ppm Al defines zircons that likely formed from peraluminous melts. (b) Cumulative probability diagram of I- and S-type LFB (Trail et al., 2017
Trail, D., Tailby, N., Wang, Y., Harrison, T.M., Boehnke, P. (2017) Aluminum in zircon as evidence for peraluminous and metaluminous melts from the Hadean to present. Geochemistry, Geophysics, Geosystems 18, 1580–1593.
) and age binned JH zircons. Numbers in inset are average XZrcAl for XZrcAl > 4 ppm zircon populations from each group.
Figure 3 (a) Histograms of P/Dy ratios and (b) scatterplot of P and Dy concentrations in zircons from I-type and S-type LFB granitoids compared with likely peraluminous (XZrcAl > 4 ppm) and non-peraluminous JH zircons. On the whole, peraluminous zircons from the JH have higher P/Dy ratios than non-peraluminous JH, and their median value of P/Dy is higher than that of peraluminous I-types. LFB data from (Burnham and Berry, 2017
Burnham, A.D., Berry, A.J. (2017) Formation of Hadean granites by melting of igneous crust. Nature Geoscience 10, 457–461.
).
Figure 4 SiO2 versus ASI for common magmatic rocks and melts. LFB and Tuolumne Intrusive Suite (TIS) intrusive rocks binned in 1 wt. % SiO2 increments, error bars are 1σ. I-type granitic rocks from the LFB (Griffin et al., 1978
Griffin, T.J., White, A.J.R., Chappell, B.W. (1978) The Moruya Batholith and geochemical contrasts between the Moruya and Jindabyne suites. Journal of the Geological Society of Australia 25, 235–247.
; Chappell and White, 1992Chappell, B.W., White, A.J.R. (1992) I- and S-type granites in the Lachlan Fold Belt. Earth and Environmental Science Transactions of the Royal Society of Edinburgh 83, 1–26.
) and intrusive rocks from the TIS (Bateman et al., 1984Bateman, P.C., Dodge, F.C.W., Bruggman, P.E. (1984) Major oxide analyses, CIPW norms, modes, and bulk specific gravities of plutonic rocks from the Mariposa 1° × 2° sheet, central Sierra Nevada, California. US Geological Survey Open-File Report 84–162.
; Ratajeski et al., 2001Ratajeski, K., Glazner, A.F., Miller, B.V. (2001) Geology and geochemistry of mafic to felsic plutonic rocks in the Cretaceous intrusive suite of Yosemite Valley, California. Geological Society of America Bulletin 113, 1486–1502.
) become peraluminous at ∼70 wt. % SiO2, whereas hydrous basalts fractionated at depths ≥7 kbar can produce melts that become peraluminous at ∼60 wt. % SiO2, unlike those fractionated at lower pressures (Blatter et al., 2013Blatter, D.L., Sisson, T.W., Hankins, W.B. (2013) Crystallization of oxidized, moderately hydrous arc basalt at mid- to lower-crustal pressures: implications for andesite genesis. Contributions to Mineralogy and Petrology 166, 861–886.
).