Physical weathering by glaciers enhances silicon mobilisation and isotopic fractionation
Affiliations | Corresponding Author | Cite as | Funding information- Share this article
-
Article views:128Cumulative count of HTML views and PDF downloads.
- Download Citation
- Rights & Permissions
top
Abstract
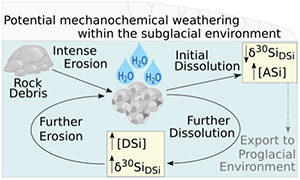
Figures
![]() Figure 1 Illustration of the experimental set up. | ![]() Figure 2 Geochemical results of primary and crushing experiments over time. DSi = black, ASi = red, closed = T2, open = T30. (a) δ30SiDSi and δ30SiASi composition, error bars represent average external error (0.08 ‰, 2 s.d.). Blue horizontal lines mark δ30Si composition of bulk rock (T2 = solid, T30 = dashed), with 2 s.d. external error shaded blue. (b) DSi concentrations. (c) ASi concentrations. Initial and secondary milled data separated by vertical grey area. Glacial river δ30SiDSi range from −0.58 to +1.01 ‰ (grey hashed region) and glacial δ30SiASi ranges from −0.86 to −0.05 ‰ (Hatton et al., 2019b). | ![]() Figure 3 ASi concentrations, grouped as T2, T30 (initial milling and dissolution), and T2-RC, T30-RC (secondary crushing and dissolution), with median (solid) and mean (dashed) shown. Grey dashed line represents discharge weighted mean ASi concentration from proglacial river of Leverett Glacier (LG) in 2015 (Hatton et al., 2019a). | ![]() Figure 4 High-resolution transmission electron microscopy (HR-TEM) photomicrographs and energy-dispersive X-ray spectra (EDS) of particles from T30-720 hr and T2-720 hr. (a1) Representative crystalline edge and EDS spectra, likely plagioclase. (a2) Representative amorphous area, containing Si and O, indicative of ASi. (b) Crystalline silicate phases representative of T2-720 hr. |
Figure 1 | Figure 2 | Figure 3 | Figure 4 |
top
Introduction
Glaciers and ice sheets are dynamic sources of nutrients and reactive particulate material to downstream ecosystems (e.g., Wadham et al., 2010
Wadham, J.L., Tranter, M., Skidmore, M., Hodson, A.J., Priscu, J., Lyons, W.B., Sharp, M., Wynn, P., Jackson, M. (2010) Biogeochemical weathering under ice: Size matters. Global Biogeochemical Cycles 24, GB3025.
; Hawkings et al., 2015Hawkings, J.R., Wadham, J.L., Tranter, M., Lawson, E., Sole, A., Cowton, T., Tedstone, A.J., Bartholomew, I., Nienow, P., Chandler, D., Telling, J. (2015) The effect of warming climate on nutrient and solute export from the Greenland Ice Sheet. Geochemical Perspectives Letters 1, 94–104.
; Sharp and Tranter, 2017Sharp, M., Tranter, M. (2017) Glacier Biogeochemistry. Geochemical Perspectives 6, 173–174.
). There is evidence that silicate mineral weathering is enhanced beneath large ice sheets (Wadham et al., 2010Wadham, J.L., Tranter, M., Skidmore, M., Hodson, A.J., Priscu, J., Lyons, W.B., Sharp, M., Wynn, P., Jackson, M. (2010) Biogeochemical weathering under ice: Size matters. Global Biogeochemical Cycles 24, GB3025.
; Michaud et al., 2016Michaud, A.B., Skidmore, M.L., Mitchell, A.C., Vick-Majors, T.J., Barbante, C., Turetta, C., vanGelder, W., Priscu, J.C. (2016) Solute sources and geochemical processes in Subglacial Lake Whillans, West Antarctica. Geology 44, 347–350.
), which may contribute significant fluxes of dissolved silicon and dissolvable amorphous silica (DSi and ASi respectively) downstream, stimulating productivity of siliceous organisms (Hendry et al., 2019Hendry, K.R., Huvenne, V.A.I., Robinson, L.F., Annett, A., Badger, M., Jacobel, A.W., Ng, H.C., Opher, J., Pickering, R.A., Taylor, M.L., Bates, S.L., Cooper, A., Cushman, G.G., Goodwin, C., Hoy, S., Rowland, G., Samperiz, A., Williams, J.A., Achterberg, E.P., Arrowsmith, C., Alexander Brearley, J., Henley, S.F., Krause, J.W., Leng, M.J., Li, T., McManus, J.F., Meredith, M.P., Perkins, R., Woodward, E.M.S. (2019) The biogeochemical impact of glacial meltwater from Southwest Greenland. Progress in Oceanography 176, 102126.
).The stable silicon isotopic composition (denoted by δ30Si) of glacial meltwaters can aid mechanistic understanding of subglacial weathering processes (Opfergelt et al., 2013
Opfergelt, S., Burton, K.W., Pogge von Strandmann, P.A.E., Gislason, S.R., Halliday, A.N. (2013) Riverine silicon isotope variations in glaciated basaltic terrains: Implications for the Si delivery to the ocean over glacial–interglacial intervals. Earth and Planetary Science Letters 369–370, 211–219.
; Hawkings et al., 2018Hawkings, J.R., Hatton, J.E., Hendry, K.R., de Souza, G.F., Wadham, J.L., Ivanovic, R., Kohler, T.J., Stibal, M., Beaton, A., Lamarche-Gagnon, G., Tedstone, A., Hain, M.P., Bagshaw, E., Pike, J., Tranter, M. (2018) The silicon cycle impacted by past ice sheets. Nature Communications 9, 3210.
; Hatton et al., 2019aHatton, J.E., Hendry, K.R., Hawkings, J.R., Wadham, J.L., Kohler, T.J., Stibal, M., Beaton, A.D., Bagshaw, E.A., Telling, J. (2019a) Investigation of subglacial weathering under the Greenland Ice Sheet using silicon isotopes. Geochimica et Cosmochimica Acta 247, 191–206.
). Previous work has hypothesised that physical weathering in the subglacial environment results in isotopically light DSi in glacial meltwaters when compared to non-glacial riverine waters (Hatton et al., 2019bHatton, J.E., Hendry, K.R., Hawkings, J.R., Wadham, J.L., Opfergelt, S., Kohler, T.J., Yde, J.C., Stibal, M., Zarsky, J.D. (2019b) Silicon Isotopes in Arctic and sub-Arctic Glacial Meltwaters: The Role of Subglacial Weathering in the Silicon Cycle. Proceedings of the Royal Society A. 475, 20190098.
). This is likely due to the creation of highly reactive mineral surfaces combined with chemical precipitation-dissolution reactions, which result in the formation and preferential dissolution of isotopically depleted amorphous weathering crusts (Opfergelt et al., 2013Opfergelt, S., Burton, K.W., Pogge von Strandmann, P.A.E., Gislason, S.R., Halliday, A.N. (2013) Riverine silicon isotope variations in glaciated basaltic terrains: Implications for the Si delivery to the ocean over glacial–interglacial intervals. Earth and Planetary Science Letters 369–370, 211–219.
; Hatton et al., 2019bHatton, J.E., Hendry, K.R., Hawkings, J.R., Wadham, J.L., Opfergelt, S., Kohler, T.J., Yde, J.C., Stibal, M., Zarsky, J.D. (2019b) Silicon Isotopes in Arctic and sub-Arctic Glacial Meltwaters: The Role of Subglacial Weathering in the Silicon Cycle. Proceedings of the Royal Society A. 475, 20190098.
). The high levels of physical erosion and sediment comminution in subglacial environments, combined with chemical weathering act to enhance the export of DSi from subglacial environments. However, a better understanding of subglacial silicate dissolution and associated Si isotopic fractionation is required to constrain the impacts of changing glacial environments on wider associated elemental cycles (e.g., nutrient liberation from silicate minerals and long term carbon drawdown from silicate weathering) and enable the inclusion of glacially exported Si in global biogeochemical models.A large proportion of the glacial Si flux is likely exported as ASi. Field observations from Leverett Glacier, Greenland reveal up to 95 % of Si exported is ASi, which is soluble in downstream marine environments (Hawkings et al., 2017
Hawkings, J.R., Wadham, J.L., Benning, L.G., Hendry, K.R., Tranter, M., Tedstone, A., Nienow, P., Raiswell, R. (2017) Ice sheets as a missing source of silica to the polar oceans. Nature Communications 8, 14198.
). The potential ASi formation mechanisms to explain elevated glacial ASi concentrations include dissolution-reprecipitation weathering where ASi forms via supersaturation at the grain boundary (Hellmann et al., 2012Hellmann, R., Wirth, R., Daval, D., Barnes, J.-P., Penisson, J.-M., Tisserand, D., Epicier, T., Florin, B., Hervig, R.L. (2012) Unifying natural and laboratory chemical weathering with interfacial dissolution–reprecipitation: A study based on the nanometer-scale chemistry of fluid–silicate interfaces. Chemical Geology 294–295, 203–216.
), and chemically leached particle surface layers resulting in an amorphous crust of less soluble ions such as Si (Casey et al., 1993Casey, W.H., Westrich, H.R., Banfield, J.F., Ferruzzi, G., Arnold, G.W. (1993) Leaching and reconstructing at the surface of dissolving chain-silicate minerals. Nature 366, 253–256.
). Additionally, silicate dissolution and ASi formation have been linked to physical processes, with ASi hypothesised to form as a disturbed surface layer (Blackburn et al., 2019Blackburn, T., Siman-Tov, S., Coble, M.A., Stock, G.M., Brodsky, E.E., Hallet, B. (2019) Composition and formation age of amorphous silica coating glacially polished surfaces. Geology 47, 347–350.
; Hatton et al., 2019bHatton, J.E., Hendry, K.R., Hawkings, J.R., Wadham, J.L., Opfergelt, S., Kohler, T.J., Yde, J.C., Stibal, M., Zarsky, J.D. (2019b) Silicon Isotopes in Arctic and sub-Arctic Glacial Meltwaters: The Role of Subglacial Weathering in the Silicon Cycle. Proceedings of the Royal Society A. 475, 20190098.
). This combination of physical erosion and chemical weathering (i.e. mechanochemical) could be key to understanding subglacial processes, considering high erosion rates and large surface areas of sediments in subglacial environments.We tested the hypotheses that high physical erosion rates in subglacial environments result in (i) isotopically light DSi via the production of highly reactive fine grained glacial flour (Opfergelt et al., 2013
Opfergelt, S., Burton, K.W., Pogge von Strandmann, P.A.E., Gislason, S.R., Halliday, A.N. (2013) Riverine silicon isotope variations in glaciated basaltic terrains: Implications for the Si delivery to the ocean over glacial–interglacial intervals. Earth and Planetary Science Letters 369–370, 211–219.
; Hatton et al., 2019bHatton, J.E., Hendry, K.R., Hawkings, J.R., Wadham, J.L., Opfergelt, S., Kohler, T.J., Yde, J.C., Stibal, M., Zarsky, J.D. (2019b) Silicon Isotopes in Arctic and sub-Arctic Glacial Meltwaters: The Role of Subglacial Weathering in the Silicon Cycle. Proceedings of the Royal Society A. 475, 20190098.
), and (ii) the formation of ASi. Dissolution experiments (Fig. 1) mimicked mechanochemical processes in the subglacial system to ascertain the role of physical weathering on Si concentrations and δ30Si composition (see Supplementary Information for full methodology). Pre-weathered unsorted glacial till (dominantly plagioclase and quartz; Fig. S-1) from Leverett Glacier proglacial plain (LG; Fig. S-2) was mechanically milled for either 2 (T2) or 30 (T30) minutes in an agate mill (note that ASi concentrations produced when milling in agate or steel mills were within analytical error, showing no significant contamination source from the agate mill, see Supplementary Information) and reacted for up to 720 hours with ultra-pure water (18.2 MΩ cm, Millipore®). The milled and reacted powders were then re-dried, re-crushed and reacted again to mimic ongoing physical erosion within a subglacial environment (Fig. 1). We chose to use field collected samples for these experiments to better mimic real world conditions and compare to existing field data.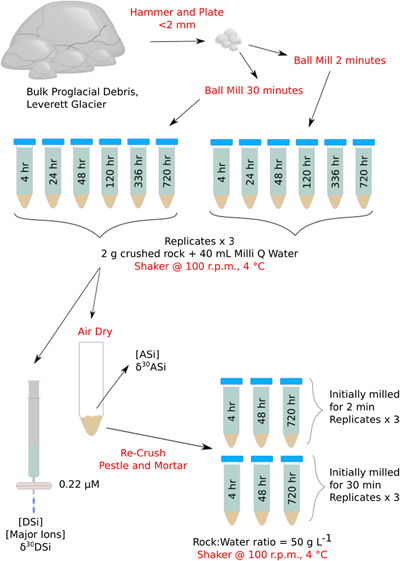
Figure 1 Illustration of the experimental set up.
top
Results and Discussion
Dissolution of freshly exposed mineral surfaces drives isotopically light DSi. Our experiments highlight the likely importance of physical erosion processes in subglacial environments. Rapid dissolution of Si after initial milling and after re-crushing led to δ30SiDSi values (down to −2.12 ‰) more depleted than values recorded in subglacial meltwater sourced DSi (+1.01 to −0.58 ‰; Hatton et al., 2019b
Hatton, J.E., Hendry, K.R., Hawkings, J.R., Wadham, J.L., Opfergelt, S., Kohler, T.J., Yde, J.C., Stibal, M., Zarsky, J.D. (2019b) Silicon Isotopes in Arctic and sub-Arctic Glacial Meltwaters: The Role of Subglacial Weathering in the Silicon Cycle. Proceedings of the Royal Society A. 475, 20190098.
). The lowest δ30SiDSi composition for T2 and T30 was measured after 4 hours, with δ30SiDSi increasing over time (Fig. 2, Table S-1). The preferential release of lighter Si isotopes during dissolution relates to the distribution of 28Si and 29Si on surface layers. Our experiments agree with previous experiments that show physical grinding leads to the preferential release of 28Si during initial dissolution of fresh surface layers, which suggests a higher abundance of 28Si on the surface of freshly ground minerals in the destabilised outer layers of the crystal structure (Ziegler et al., 2005Ziegler, K., Chadwick, O.A., Brzezinski, M.A., Kelly, E.F. (2005) Natural variations of δ30Si ratios during progressive basalt weathering, Hawaiian Islands. Geochimica et Cosmochimica Acta 69, 4597–4610.
). It’s likely that the high surface areas created by physical grinding led to rapid initial dissolution of Si, with the largest isotopic fractionation. As the experiment progressed, the δ30SiDSi composition increased toward δ30SiBulk values (Fig. 2) as proportionally more 29Si was liberated from internal crystalline structures.
Figure 2 Geochemical results of primary and crushing experiments over time. DSi = black, ASi = red, closed = T2, open = T30. (a) δ30SiDSi and δ30SiASi composition, error bars represent average external error (0.08 ‰, 2 s.d.). Blue horizontal lines mark δ30Si composition of bulk rock (T2 = solid, T30 = dashed), with 2 s.d. external error shaded blue. (b) DSi concentrations. (c) ASi concentrations. Initial and secondary milled data separated by vertical grey area. Glacial river δ30SiDSi range from −0.58 to +1.01 ‰ (grey hashed region) and glacial δ30SiASi ranges from −0.86 to −0.05 ‰ (Hatton et al., 2019b
Hatton, J.E., Hendry, K.R., Hawkings, J.R., Wadham, J.L., Opfergelt, S., Kohler, T.J., Yde, J.C., Stibal, M., Zarsky, J.D. (2019b) Silicon Isotopes in Arctic and sub-Arctic Glacial Meltwaters: The Role of Subglacial Weathering in the Silicon Cycle. Proceedings of the Royal Society A. 475, 20190098.
).After the reacted material was re-crushed, the δ30SiDSi composition decreased again (down to −1.29 ‰; Fig. 2, Table S-2). The decrease in δ30SiDSi composition after re-crushing, suggests that the regeneration of reactive mineral surface layers by physical action promotes further dissolution of isotopically light Si. This process presents a viable mechanism to explain isotopically light DSi in glacial meltwaters, due to the continuous comminution of bedrock in the subglacial system.
Our findings of initial dissolution resulting in low δ30SiDSi composition are consistent with results of leaching experiments on basaltic lavas (Ziegler et al., 2005
Ziegler, K., Chadwick, O.A., Brzezinski, M.A., Kelly, E.F. (2005) Natural variations of δ30Si ratios during progressive basalt weathering, Hawaiian Islands. Geochimica et Cosmochimica Acta 69, 4597–4610.
) and diatom opal (Demarest et al., 2009Demarest, M.S., Brzezinski, M.A., Beucher, C.P. (2009) Fractionation of silicon isotopes during biogenic silica dissolution. Geochimica et Cosmochimica Acta 73, 5572–5583.
), suggesting this process is independent of sediment lithology. While our experiments do not isolate the exact mechanism driving the apparent fractionation during initial dissolution, they do suggest that continuous comminution of subglacial sediments results in exposure of fresh, large surface areas. This reactive surface layer promotes the dissolution of Si and drives the light δ30SiDSi composition in glacial meltwaters (Opfergelt et al., 2013Opfergelt, S., Burton, K.W., Pogge von Strandmann, P.A.E., Gislason, S.R., Halliday, A.N. (2013) Riverine silicon isotope variations in glaciated basaltic terrains: Implications for the Si delivery to the ocean over glacial–interglacial intervals. Earth and Planetary Science Letters 369–370, 211–219.
; Hatton et al., 2019bHatton, J.E., Hendry, K.R., Hawkings, J.R., Wadham, J.L., Opfergelt, S., Kohler, T.J., Yde, J.C., Stibal, M., Zarsky, J.D. (2019b) Silicon Isotopes in Arctic and sub-Arctic Glacial Meltwaters: The Role of Subglacial Weathering in the Silicon Cycle. Proceedings of the Royal Society A. 475, 20190098.
).The degree of isotopic fractionation does not appear to scale with DSi concentration and the available surface area. T2 experiments had lighter δ30SiDSi composition than T30 for all timepoints (Fig. 2), despite lower DSi concentrations (Fig. 2) and lower specific surface area deduced from mean grain size of material (Fig. S-3; Telling et al., 2015
Telling, J., Boyd, E.S., Bone, N., Jones, E.L., Tranter, M., MacFarlane, J.W., Martin, P.G., Wadham, J.L., Lamarche-Gagnon, G., Skidmore, M.L., Hamilton, T.L., Hill, E., Jackson, M., Hodgson, D.A. (2015) Rock comminution as a source of hydrogen for subglacial ecosystems. Nature Geoscience 8, 851–855.
). There are potential explanations for this, considering i) isotopic heterogeneity within the starting material, ii) natural pre-weathering of rock material, and/or iii) ASi formation and dissolution. The rocks used in this experiment from the proglacial plain, are naturally heterogeneous, and were pre-weathered within the sub- and pro-glacial environment. We see evidence of amorphous SiO2 nanoparticles in all samples via HR-TEM imaging (see Fig. 4), highlighting a degree of environmental pre-weathering prior to sample collection. This pre-weathering of material could have resulted in surface layers with a lower δ30Si composition compared to the bulk rock material due to the fractionation induced by weathering processes (Frings et al., 2016Frings, P.J., Clymans, W., Fontorbe, G., De La Rocha, C., Conley, D.J. (2016) The continental Si cycle and its impact on the ocean Si isotope budget. Chemical Geology 425, 12–36.
). When the rocks were milled for a short time (T2), the fresh mineral surfaces were comparatively enriched with Si from these pre-weathered surfaces (e.g., isotopically light amorphous nanoparticles). Whereas, longer milling in T30 exposed more of the non-weathered crystalline structure from where proportionally more DSi was derived. This could explain why T30 DSi was marginally closer to the bulk rock value (−0.13 ± 0.16 ‰). Alternatively, the higher ASi concentrations in T30 experiments may have impacted the overall δ30SiDSi composition. Previous experiments have shown large isotopic fractionations (up to −7.5 ‰) associated with quartz cement dissolution and precipitation (Basile-Doelsch et al., 2005Basile-Doelsch, I., Meunier, J.D., Parron, C. (2005) Another continental pool in the terrestrial silicon cycle. Nature 433, 399–402.
). If there was proportionally greater ASi dissolution in T30 experiments, when compared to T2 experiments, then this could have resulted in a smaller overall fractionation in T30 and a less depleted δ30SiDSi composition.Our experiments do not allow for a detailed analysis of the difference in δ30SiDSi composition between T2 and T30 resulting from dissolution, particularly with the potential impacts of the environmental pre-weathering. However, the overall differences are small compared to the large fractionation observed after initial dissolution. Therefore, we hypothesise that continuous subglacial physical erosion of bedrock could lead to isotopically low δ30SiDSi after wetting sediments with dilute waters. This process would likely explain the observed low meltwater δ30SiDSi composition (Hatton et al., 2019b
Hatton, J.E., Hendry, K.R., Hawkings, J.R., Wadham, J.L., Opfergelt, S., Kohler, T.J., Yde, J.C., Stibal, M., Zarsky, J.D. (2019b) Silicon Isotopes in Arctic and sub-Arctic Glacial Meltwaters: The Role of Subglacial Weathering in the Silicon Cycle. Proceedings of the Royal Society A. 475, 20190098.
).Mechanochemical reactions may promote ASi formation in subglacial systems. A more robust understanding of the formation process of subglacial ASi is important considering the large flux term of glacial ASi to downstream environments (Hawkings et al., 2017
Hawkings, J.R., Wadham, J.L., Benning, L.G., Hendry, K.R., Tranter, M., Tedstone, A., Nienow, P., Raiswell, R. (2017) Ice sheets as a missing source of silica to the polar oceans. Nature Communications 8, 14198.
). We use these experiments to deduce the potential role of physical erosion in ASi formation. ASi concentrations were consistently higher in T30 compared to T2 (Fig. 3, Tables S-1, S-2) after both the initial milling (T30 ∼ 0.8 % d.w., T2 ∼ 0.2 % d.w.) and then re-crushing (T30 ∼ 1.2 % d.w., T2 ∼ 0.3 % d.w.), indicating that more intense physical erosion and generation of larger particle surface areas resulted in higher observed ASi concentrations. The increase in ASi from the end of the initial dissolution to when the material was re-crushed also indicates that the secondary crushing regenerates fresh amorphous mineral surfaces. It is likely that the milling process results in greater ASi formation, which is more soluble as a result of a greater surface area from longer milling times. Elevated ASi concentrations from crushing are consistent with field observations of ASi-rich precipitates produced by subglacial physical and chemical weathering processes during the last glaciation (Blackburn et al., 2019Blackburn, T., Siman-Tov, S., Coble, M.A., Stock, G.M., Brodsky, E.E., Hallet, B. (2019) Composition and formation age of amorphous silica coating glacially polished surfaces. Geology 47, 347–350.
), and ASi formation by comminution and associated breakdown of crystalline structures (Yund et al., 1990Yund, R.A., Blanpied, M.L., Tullis, T.E., Weeks, J.D. (1990) Amorphous material in high strain experimental fault gouges. Journal of Geophysical Research 95, 15589–15602.
).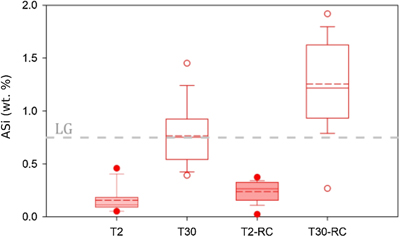
Figure 3 ASi concentrations, grouped as T2, T30 (initial milling and dissolution), and T2-RC, T30-RC (secondary crushing and dissolution), with median (solid) and mean (dashed) shown. Grey dashed line represents discharge weighted mean ASi concentration from proglacial river of Leverett Glacier (LG) in 2015 (Hatton et al., 2019a
Hatton, J.E., Hendry, K.R., Hawkings, J.R., Wadham, J.L., Kohler, T.J., Stibal, M., Beaton, A.D., Bagshaw, E.A., Telling, J. (2019a) Investigation of subglacial weathering under the Greenland Ice Sheet using silicon isotopes. Geochimica et Cosmochimica Acta 247, 191–206.
).A confounding factor is that HR-TEM photomicrographs indicate the presence of amorphous SiO2 nanoparticle aggregates in all samples (Fig. 4). There was also no statistical difference in overall crystallinity between starting material and milled material when completing pair distribution function (PDF) analysis of the X-ray scattering, though observable peak differences were present (Fig. S-4). These small concentrations of chemically sourced ASi observable under HR-TEM, likely result from environmental pre-weathering. Despite this, the low ASi concentrations of unmilled control experiments (avg. 0.11 % d.w.; Table S-6) combined with the elevated ASi concentrations for all T30 experiments (avg. 1.03 % d.w.), provide strong evidence for the importance of intense physical erosion in amorphisation and the mobilisation of pre-existing reactive Si, mimicking the subglacial environment.
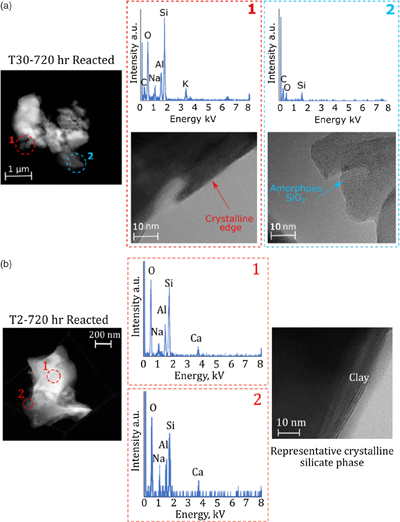
Figure 4 High-resolution transmission electron microscopy (HR-TEM) photomicrographs and energy-dispersive X-ray spectra (EDS) of particles from T30-720 hr and T2-720 hr. (a1) Representative crystalline edge and EDS spectra, likely plagioclase. (a2) Representative amorphous area, containing Si and O, indicative of ASi. (b) Crystalline silicate phases representative of T2-720 hr.
The δ30SiASi composition (Fig. 2) was not significantly different over the incubation timescale (ANOVA, p value 0.06), between T2 and T30 (ANOVA, p value 0.83), and after re-crushing (ANOVA, p value 0.87), with an overall average δ30SiASi of −0.22 ± 0.15 ‰ (2 s.d.). It is likely that this relatively consistent δ30SiASi composition is linked to the large ASi reservoir compared to DSi. Our experimental values are similar in composition to field measurements over a summer melt season at LG (−0.32 to −0.11 ‰; Hatton et al., 2019a
Hatton, J.E., Hendry, K.R., Hawkings, J.R., Wadham, J.L., Kohler, T.J., Stibal, M., Beaton, A.D., Bagshaw, E.A., Telling, J. (2019a) Investigation of subglacial weathering under the Greenland Ice Sheet using silicon isotopes. Geochimica et Cosmochimica Acta 247, 191–206.
), demonstrating the likely similarities between the subglacial processes forming ASi in the field and in our experiments.top
Implications for Subglacial Silicon Cycling
Our findings highlight the importance of mechanochemical processes beneath glaciers for silicon cycling despite near freezing water temperatures. Our study improves understanding of the mechanistic drivers of subglacial Si cycling, which will help inform wider biogeochemical models. Our experiments highlight the potential of glacial physical weathering in creating high concentrations of labile ASi and a distinct isotopic signature for DSi. Physical erosion appears essential in creating fresh reactive mineral surfaces that lead to the dissolution of isotopically light DSi in subglacial environments. It is therefore likely that actively eroding glaciers with high suspended sediment concentrations and an active subglacial hydrological system will export meltwaters with isotopically light DSi compared to non-glacial rivers. The variation in δ30SiDSi composition and ASi concentrations between glacierised catchments could be explained by differences in erosional processes, such as heterogeneity within and between glacial ice flow rates, subglacial hydrological conditions, starting bedrock composition, and resulting mobilised sediment surface areas. Further work considering mechanistic processes using pristine starting minerals could be beneficial. Such work, combined with our findings would be key to informing a developed conceptual model of glacial biogeochemical weathering processes to consider the sensitivity of glacier and ice sheet melt to climatic warming, and the potential role of these systems in global elemental cycling.
top
Author Contributions
JEH and KRH conceived the study. JEH, RB, VR, LGB, HCN and TW completed laboratory analysis. All authors were involved in writing the manuscript, data interpretation and discussion.
top
Acknowledgements
The authors thank the fieldwork team at Leverett Glacier for all the hard work during the 2015 field season. The authors are also grateful for laboratory support within the Bristol Isotope Group (BIG), with particular thanks Dr. Christopher D. Coath and Dr. Lucie Cassarino. Thanks also goes to laboratory support from the LowTex and Microbeam laboratories at the University of Bristol, especially Dr. Fotis Sgouridis, Miss Ioanna Petropoulou and Dr. Stuart Kearns.
JEH and KRH were funded by ERC Starting Grant ICY-LAB (grant agreement 678371). NERC standard grant DELVE (NE/I008845/1), Leverhulme Research Grant RPG-2016-439. JRH was further supported by an EC Horizon 2020 Marie Skłodowska-Curie Actions fellowship (grant agreement 793962) and JLW was supported by a Royal Society Wolfson Merit Award. The GFZ team acknowledges financial support from the Helmholtz Recruiting Initiative (Award No. I-044-16-0).
Editor: Gavin Foster
top
References
Basile-Doelsch, I., Meunier, J.D., Parron, C. (2005) Another continental pool in the terrestrial silicon cycle. Nature 433, 399–402.

Previous experiments have shown large isotopic fractionations (up to −7.5 ‰) associated with quartz cement dissolution and precipitation (Basile-Doelsch et al., 2005).
View in article
Blackburn, T., Siman-Tov, S., Coble, M.A., Stock, G.M., Brodsky, E.E., Hallet, B. (2019) Composition and formation age of amorphous silica coating glacially polished surfaces. Geology 47, 347–350.

Additionally, silicate dissolution and ASi formation have been linked to physical processes, with ASi hypothesised to form as a disturbed surface layer (Blackburn et al., 2019; Hatton et al., 2019b).
View in article
Elevated ASi concentrations from crushing are consistent with field observations of ASi-rich precipitates produced by subglacial physical and chemical weathering processes during the last glaciation (Blackburn et al., 2019), and ASi formation by comminution and associated breakdown of crystalline structures (Yund et al., 1990).
View in article
Casey, W.H., Westrich, H.R., Banfield, J.F., Ferruzzi, G., Arnold, G.W. (1993) Leaching and reconstructing at the surface of dissolving chain-silicate minerals. Nature 366, 253–256.

The potential ASi formation mechanisms to explain elevated glacial ASi concentrations include dissolution-reprecipitation weathering where ASi forms via supersaturation at the grain boundary (Hellmann et al., 2012), and chemically leached particle surface layers resulting in an amorphous crust of less soluble ions such as Si (Casey et al., 1993).
View in article
Demarest, M.S., Brzezinski, M.A., Beucher, C.P. (2009) Fractionation of silicon isotopes during biogenic silica dissolution. Geochimica et Cosmochimica Acta 73, 5572–5583.

Our findings of initial dissolution resulting in low δ30SiDSi composition are consistent with results of leaching experiments on basaltic lavas (Ziegler et al., 2005) and diatom opal (Demarest et al., 2009), suggesting this process is independent of sediment lithology.
View in article
Frings, P.J., Clymans, W., Fontorbe, G., De La Rocha, C., Conley, D.J. (2016) The continental Si cycle and its impact on the ocean Si isotope budget. Chemical Geology 425, 12–36.

This pre-weathering of material could have resulted in surface layers with a lower δ30Si composition compared to the bulk rock material due to the fractionation induced by weathering processes (Frings et al., 2016).
View in article
Hatton, J.E., Hendry, K.R., Hawkings, J.R., Wadham, J.L., Kohler, T.J., Stibal, M., Beaton, A.D., Bagshaw, E.A., Telling, J. (2019a) Investigation of subglacial weathering under the Greenland Ice Sheet using silicon isotopes. Geochimica et Cosmochimica Acta 247, 191–206.

Grey dashed line represents discharge weighted mean ASi concentration from proglacial river of Leverett Glacier (LG) in 2015 (Hatton et al., 2019a).
View in article
Our experimental values are similar in composition to field measurements over a summer melt season at LG (−0.32 to −0.11 ‰; (Hatton et al., 2019a)), demonstrating the likely similarities between the subglacial processes forming ASi in the field and in our experiments.
View in article
The stable silicon isotopic composition (denoted by δ30Si) of glacial meltwaters can aid mechanistic understanding of subglacial weathering processes (Opfergelt et al., 2013; Hawkings et al., 2018; Hatton et al., 2019a).
View in article
Hatton, J.E., Hendry, K.R., Hawkings, J.R., Wadham, J.L., Opfergelt, S., Kohler, T.J., Yde, J.C., Stibal, M., Zarsky, J.D. (2019b) Silicon Isotopes in Arctic and sub-Arctic Glacial Meltwaters: The Role of Subglacial Weathering in the Silicon Cycle. Proceedings of the Royal Society A. 475, 20190098.

Previous work has hypothesised that physical weathering in the subglacial environment results in isotopically light DSi in glacial meltwaters when compared to non-glacial riverine waters (Hatton et al., 2019b).
View in article
This is likely due to the creation of highly reactive mineral surfaces combined with chemical precipitation-dissolution reactions, which result in the formation and preferential dissolution of isotopically depleted amorphous weathering crusts (Opfergelt et al., 2013; Hatton et al., 2019b).
View in article
Additionally, silicate dissolution and ASi formation have been linked to physical processes, with ASi hypothesised to form as a disturbed surface layer (Blackburn et al., 2019; Hatton et al., 2019b).
View in article
Rapid dissolution of Si after initial milling and after re-crushing led to δ30SiDSi values (down to −2.12 ‰) more depleted than values recorded in subglacial meltwater sourced DSi (+1.01 to −0.58 ‰; Hatton et al., 2019b).
View in article
Glacial river δ30SiDSi range from −0.58 to +1.01 ‰ (grey hashed region) and glacial δ30SiASi ranges from −0.86 to −0.05 ‰ (Hatton et al., 2019b).
View in article
We tested the hypotheses that high physical erosion rates in subglacial environments result in (i) isotopically light DSi via the production of highly reactive fine grained glacial flour (Opfergelt et al., 2013; Hatton et al., 2019b), and (ii) the formation of ASi. Dissolution experiments (Fig. 1) mimicked mechanochemical processes in the subglacial system to ascertain the role of physical weathering on Si concentrations and δ30Si composition (see Supplementary Information for full methodology).
View in article
This process would likely explain the observed low meltwater δ30SiDSi composition (Hatton et al., 2019b).
View in article
This reactive surface layer promotes the dissolution of Si and drives the light δ30SiDSi composition in glacial meltwaters (Opfergelt et al., 2013; Hatton et al., 2019b).
View in article
Hawkings, J.R., Wadham, J.L., Tranter, M., Lawson, E., Sole, A., Cowton, T., Tedstone, A.J., Bartholomew, I., Nienow, P., Chandler, D., Telling, J. (2015) The effect of warming climate on nutrient and solute export from the Greenland Ice Sheet. Geochemical Perspectives Letters 1, 94–104.

Glaciers and ice sheets are dynamic sources of nutrients and reactive particulate material to downstream ecosystems (e.g., Wadham et al., 2010; Hawkings et al., 2015; Sharp and Tranter, 2017).
View in article
Hawkings, J.R., Wadham, J.L., Benning, L.G., Hendry, K.R., Tranter, M., Tedstone, A., Nienow, P., Raiswell, R. (2017) Ice sheets as a missing source of silica to the polar oceans. Nature Communications 8, 14198.

Field observations from Leverett Glacier, Greenland reveal up to 95 % of Si exported is ASi, which is soluble in downstream marine environments (Hawkings et al., 2017).
View in article
A more robust understanding of the formation process of subglacial ASi is important considering the large flux term of glacial ASi to downstream environments (Hawkings et al., 2017).
View in article
Hawkings, J.R., Hatton, J.E., Hendry, K.R., de Souza, G.F., Wadham, J.L., Ivanovic, R., Kohler, T.J., Stibal, M., Beaton, A., Lamarche-Gagnon, G., Tedstone, A., Hain, M.P., Bagshaw, E., Pike, J., Tranter, M. (2018) The silicon cycle impacted by past ice sheets. Nature Communications 9, 3210.

The stable silicon isotopic composition (denoted by δ30Si) of glacial meltwaters can aid mechanistic understanding of subglacial weathering processes (Opfergelt et al., 2013; Hawkings et al., 2018; Hatton et al., 2019a).
View in article
Hellmann, R., Wirth, R., Daval, D., Barnes, J.-P., Penisson, J.-M., Tisserand, D., Epicier, T., Florin, B., Hervig, R.L. (2012) Unifying natural and laboratory chemical weathering with interfacial dissolution–reprecipitation: A study based on the nanometer-scale chemistry of fluid–silicate interfaces. Chemical Geology 294–295, 203–216.

The potential ASi formation mechanisms to explain elevated glacial ASi concentrations include dissolution-reprecipitation weathering where ASi forms via supersaturation at the grain boundary (Hellmann et al., 2012), and chemically leached particle surface layers resulting in an amorphous crust of less soluble ions such as Si (Casey et al., 1993).
View in article
Hendry, K.R., Huvenne, V.A.I., Robinson, L.F., Annett, A., Badger, M., Jacobel, A.W., Ng, H.C., Opher, J., Pickering, R.A., Taylor, M.L., Bates, S.L., Cooper, A., Cushman, G.G., Goodwin, C., Hoy, S., Rowland, G., Samperiz, A., Williams, J.A., Achterberg, E.P., Arrowsmith, C., Alexander Brearley, J., Henley, S.F., Krause, J.W., Leng, M.J., Li, T., McManus, J.F., Meredith, M.P., Perkins, R., Woodward, E.M.S. (2019) The biogeochemical impact of glacial meltwater from Southwest Greenland. Progress in Oceanography 176, 102126.

There is evidence that silicate mineral weathering is enhanced beneath large ice sheets (Wadham et al., 2010; Michaud et al., 2016), which may contribute significant fluxes of dissolved silicon and dissolvable amorphous silica (DSi and ASi respectively) downstream, stimulating productivity of siliceous organisms (Hendry et al., 2019).
View in article
Michaud, A.B., Skidmore, M.L., Mitchell, A.C., Vick-Majors, T.J., Barbante, C., Turetta, C., vanGelder, W., Priscu, J.C. (2016) Solute sources and geochemical processes in Subglacial Lake Whillans, West Antarctica. Geology 44, 347–350.

There is evidence that silicate mineral weathering is enhanced beneath large ice sheets (Wadham et al., 2010; Michaud et al., 2016), which may contribute significant fluxes of dissolved silicon and dissolvable amorphous silica (DSi and ASi respectively) downstream, stimulating productivity of siliceous organisms (Hendry et al., 2019).
View in article
Opfergelt, S., Burton, K.W., Pogge von Strandmann, P.A.E., Gislason, S.R., Halliday, A.N. (2013) Riverine silicon isotope variations in glaciated basaltic terrains: Implications for the Si delivery to the ocean over glacial–interglacial intervals. Earth and Planetary Science Letters 369–370, 211–219.

The stable silicon isotopic composition (denoted by δ30Si) of glacial meltwaters can aid mechanistic understanding of subglacial weathering processes (Opfergelt et al., 2013; Hawkings et al., 2018; Hatton et al., 2019a).
View in article
This is likely due to the creation of highly reactive mineral surfaces combined with chemical precipitation-dissolution reactions, which result in the formation and preferential dissolution of isotopically depleted amorphous weathering crusts (Opfergelt et al., 2013; Hatton et al., 2019b).
View in article
We tested the hypotheses that high physical erosion rates in subglacial environments result in (i) isotopically light DSi via the production of highly reactive fine grained glacial flour (Opfergelt et al., 2013; Hatton et al., 2019b), and (ii) the formation of ASi. Dissolution experiments (Fig. 1) mimicked mechanochemical processes in the subglacial system to ascertain the role of physical weathering on Si concentrations and δ30Si composition (see Supplementary Information for full methodology).
View in article
This reactive surface layer promotes the dissolution of Si and drives the light δ30SiDSi composition in glacial meltwaters (Opfergelt et al., 2013; Hatton et al., 2019b).
View in article
Sharp, M., Tranter, M. (2017) Glacier Biogeochemistry. Geochemical Perspectives 6, 173–174.

Glaciers and ice sheets are dynamic sources of nutrients and reactive particulate material to downstream ecosystems (e.g., Wadham et al., 2010; Hawkings et al., 2015; Sharp and Tranter, 2017).
View in article
Telling, J., Boyd, E.S., Bone, N., Jones, E.L., Tranter, M., MacFarlane, J.W., Martin, P.G., Wadham, J.L., Lamarche-Gagnon, G., Skidmore, M.L., Hamilton, T.L., Hill, E., Jackson, M., Hodgson, D.A. (2015) Rock comminution as a source of hydrogen for subglacial ecosystems. Nature Geoscience 8, 851–855.

T2 experiments had lighter δ30SiDSi composition than T30 for all timepoints (Fig. 2), despite lower DSi concentrations (Fig. 2) and lower specific surface area deduced from mean grain size of material (Fig. S-3; Telling et al., 2015).
View in article
Wadham, J.L., Tranter, M., Skidmore, M., Hodson, A.J., Priscu, J., Lyons, W.B., Sharp, M., Wynn, P., Jackson, M. (2010) Biogeochemical weathering under ice: Size matters. Global Biogeochemical Cycles 24, GB3025.

Glaciers and ice sheets are dynamic sources of nutrients and reactive particulate material to downstream ecosystems (e.g., Wadham et al., 2010; Hawkings et al., 2015; Sharp and Tranter, 2017).
View in article
There is evidence that silicate mineral weathering is enhanced beneath large ice sheets (Wadham et al., 2010; Michaud et al., 2016), which may contribute significant fluxes of dissolved silicon and dissolvable amorphous silica (DSi and ASi respectively) downstream, stimulating productivity of siliceous organisms (Hendry et al., 2019).
View in article
Yund, R.A., Blanpied, M.L., Tullis, T.E., Weeks, J.D. (1990) Amorphous material in high strain experimental fault gouges. Journal of Geophysical Research 95, 15589–15602.

Elevated ASi concentrations from crushing are consistent with field observations of ASi-rich precipitates produced by subglacial physical and chemical weathering processes during the last glaciation (Blackburn et al., 2019), and ASi formation by comminution and associated breakdown of crystalline structures (Yund et al., 1990).
View in article
Ziegler, K., Chadwick, O.A., Brzezinski, M.A., Kelly, E.F. (2005) Natural variations of δ30Si ratios during progressive basalt weathering, Hawaiian Islands. Geochimica et Cosmochimica Acta 69, 4597–4610.

Our experiments agree with previous experiments that show physical grinding leads to the preferential release of 28Si during initial dissolution of fresh surface layers, which suggests a higher abundance of 28Si on the surface of freshly ground minerals in the destabilised outer layers of the crystal structure (Ziegler et al., 2005).
View in article
Our findings of initial dissolution resulting in low δ30SiDSi composition are consistent with results of leaching experiments on basaltic lavas (Ziegler et al., 2005) and diatom opal (Demarest et al., 2009), suggesting this process is independent of sediment lithology.
View in article
top
Supplementary Information
The Supplementary Information includes:
- Sample Description
- Detailed Experimental Set-Up
- Agate Mill Tests
- Laboratory Analyses
- Tables S-1 to S-6
- Figures S-1 to S-6
- Supplementary Information References
Download the Supplementary Information (PDF).
Figures

Figure 1 Illustration of the experimental set up.

Figure 2 Geochemical results of primary and crushing experiments over time. DSi = black, ASi = red, closed = T2, open = T30. (a) δ30SiDSi and δ30SiASi composition, error bars represent average external error (0.08 ‰, 2 s.d.). Blue horizontal lines mark δ30Si composition of bulk rock (T2 = solid, T30 = dashed), with 2 s.d. external error shaded blue. (b) DSi concentrations. (c) ASi concentrations. Initial and secondary milled data separated by vertical grey area. Glacial river δ30SiDSi range from −0.58 to +1.01 ‰ (grey hashed region) and glacial δ30SiASi ranges from −0.86 to −0.05 ‰ (Hatton et al., 2019b
Hatton, J.E., Hendry, K.R., Hawkings, J.R., Wadham, J.L., Opfergelt, S., Kohler, T.J., Yde, J.C., Stibal, M., Zarsky, J.D. (2019b) Silicon Isotopes in Arctic and sub-Arctic Glacial Meltwaters: The Role of Subglacial Weathering in the Silicon Cycle. Proceedings of the Royal Society A. 475, 20190098.
).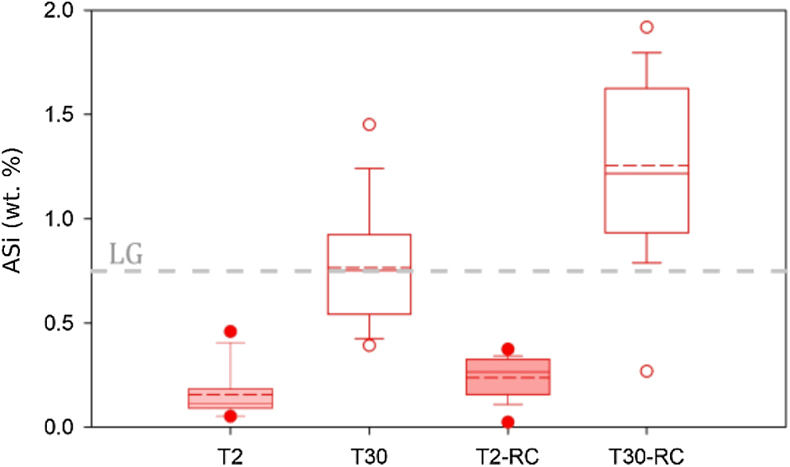
Figure 3 ASi concentrations, grouped as T2, T30 (initial milling and dissolution), and T2-RC, T30-RC (secondary crushing and dissolution), with median (solid) and mean (dashed) shown. Grey dashed line represents discharge weighted mean ASi concentration from proglacial river of Leverett Glacier (LG) in 2015 (Hatton et al., 2019a
Hatton, J.E., Hendry, K.R., Hawkings, J.R., Wadham, J.L., Kohler, T.J., Stibal, M., Beaton, A.D., Bagshaw, E.A., Telling, J. (2019a) Investigation of subglacial weathering under the Greenland Ice Sheet using silicon isotopes. Geochimica et Cosmochimica Acta 247, 191–206.
).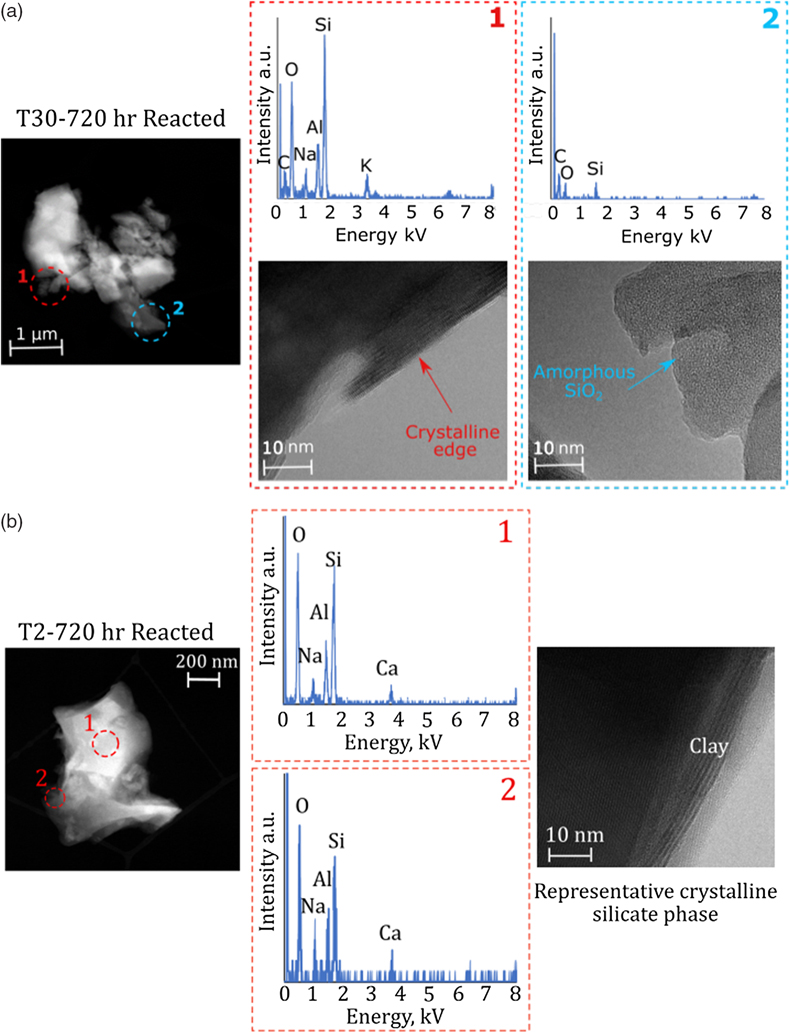
Figure 4 High-resolution transmission electron microscopy (HR-TEM) photomicrographs and energy-dispersive X-ray spectra (EDS) of particles from T30-720 hr and T2-720 hr. (a1) Representative crystalline edge and EDS spectra, likely plagioclase. (a2) Representative amorphous area, containing Si and O, indicative of ASi. (b) Crystalline silicate phases representative of T2-720 hr.