Isotopic evidence of sulfur photochemistry during lunar regolith formation
Affiliations | Corresponding Author | Cite as | Funding information- Share this article
Article views:132Cumulative count of HTML views and PDF downloads.
- Download Citation
- Rights & Permissions
top
Abstract

Figures
![]() Figure 1 (a) Δ33S vs. δ34S and (b) Δ36S vs. Δ33S of analysed lunar soils 75081, 690 (red) and 74241, 204 (blue) and literature data from lunar basalts (grey). In (b), we highlight that data from 75081, 690 indicates the photochemistry occurring is different from that in other planetary environments, such as early Earth (e.g., Johnston, 2011; Archean reference slope, grey dashed line) and the early solar nebula (e.g., Antonelli et al., 2014; Lyman-alpha photolysis, black dashed line). | ![]() Figure 2 Hypothesis for the origin of sulfur isotope variations in lunar soils. (a) Micrometeorite impacts result in volatilisation and loss of sulfur at the edge of grains. (b) Vapourised sulfur is added to the tenuous lunar atmosphere and travels up to 3000 km before condensing. Here, gaseous sulfur molecules can escape, which induces mass dependent 34S enrichments, and they can undergo photolytic reactions, resulting in mass independent fractionation (MIF) (seen in 75081, 690). (c) Vapour condenses on regolith soil with isotopic evidence of how sample was processed. |
Figure 1 | Figure 2 |
top
Introduction
The earliest atmospheres on Earth and Mars were optically thin and contained sufficient sulfur-bearing gaseous molecules and penetration of ultraviolet (UV) light that generated mass independent fractionation of sulfur (MIF-S) isotopes (e.g., Farquhar et al., 2000
Farquhar, J., Bao, H., Thiemens, M. (2000) Atmospheric Influence of Earth’s Earliest Sulfur Cycle. Science 289, 756–758. https://doi.org/10.1126/science.289.5480.756
; Franz et al., 2014Franz, H.B., Kim, S.-T., Farquhar, J., Day, J.M.D., Economos, R.C., et al. (2014) Isotopic links between atmospheric chemistry and the deep sulphur cycle on Mars. Nature 508, 364–368. https://doi.org/10.1038/nature13175
). These sulfur isotope records shed light on the geochemical conditions involving sulfur and other elements and provide key information about the evolution of these planets’ fluid envelopes. The early evolution of the Moon (3.8–3.1 Ga) included pyroclastic and effusive volcanism, and large impact events that provided enough gas to produce optically thin transient atmospheres (e.g., Prem et al., 2015Prem, P., Artemieva, N.A., Goldstein, D.B., Varghese, P.L., Trafton, L.M. (2015) Transport of water in a transient impact-generated lunar atmosphere. Icarus 255, 148–158. https://doi.org/10.1016/j.icarus.2014.10.017
; Needham and Kring, 2017Needham, D.H., Kring, D.A. (2017) Lunar volcanism produced a transient atmosphere around the ancient Moon. Earth and Planetary Science Letters 478, 175–178. https://doi.org/10.1016/j.epsl.2017.09.002
) where UV light can penetrate and produce MIF-S. To date, no unambiguous evidence of this process has been found on the Moon.We present new analyses of the quadruple sulfur isotope compositions and sulfur concentrations for 9 and 10 size fractions (<10 to >500 μm and >1000 μm) from lunar basaltic regolith samples 74241, 204 (immature, Is/FeO = 5.1) and 75081, 690 (sub-mature, Is/FeO = 40) (Morris, 1978
Morris, R.V. (1978) The surface exposure (maturity) of lunar soils: Some concepts and Is/FeO compilation. Proceedings of the Ninth Lunar and Planetary Science Conference, 2287–2297. https://articles.adsabs.harvard.edu/pdf/1978LPSC....9.2287M
) (Table S-1). These analyses provide insight into the late stage lunar volatile cycle during surface gardening and the evolution of sulfur isotope compositions of soils of varying maturity.top
Methods
74241, 204 and 75081, 690 were sieved into 9 and 10 grain size fractions, respectively. Sulfur from each sieve fraction was extracted using an HF + CrCl2 digestion method and analysed as SF6 using a ThermoFinnigan MAT253 Dual Inlet isotope ratio mass spectrometer (see Supplementary Information S-1 for details). Isotopic data are reported in per mil using the following notation:
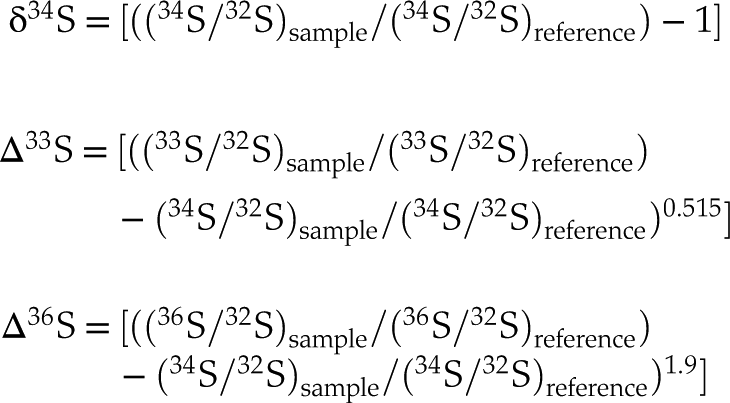
Uncertainties on δ34S and Δ36S (±0.3 ‰) reflect the long term uncertainty on repeated measurements of reference material IAEA-S1. Uncertainty on Δ33S reflects mass spectrometry uncertainty associated with counts on 33S and is similar to our long term uncertainty estimates (±0.016 ‰ and ±0.008 ‰, for short and long counting sessions, respectively; see Supplementary Information S-1).
top
Results and Discussion
We observe non-zero Δ33S and Δ36S values in 75081, 690 (Fig. 1). The same non-zero variability is not observed in 74241, 204. The dichotomy in Δ33S and Δ36S among 74241, 204 and 75081, 690 is unclear, but indicates that there are processes operating on only some locations of the lunar surface.
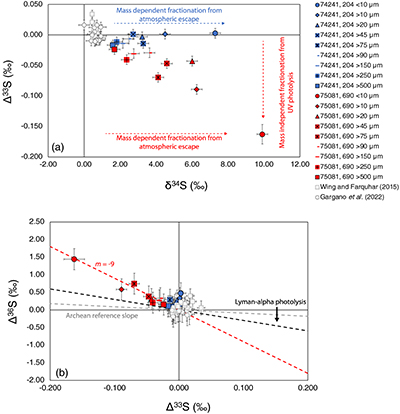
Figure 1 (a) Δ33S vs. δ34S and (b) Δ36S vs. Δ33S of analysed lunar soils 75081, 690 (red) and 74241, 204 (blue) and literature data from lunar basalts (grey). In (b), we highlight that data from 75081, 690 indicates the photochemistry occurring is different from that in other planetary environments, such as early Earth (e.g., Johnston, 2011
Johnston, D.T. (2011) Multiple sulfur isotopes and the evolution of Earth’s surface sulfur cycle. Earth-Science Reviews 106, 161–183. https://doi.org/10.1016/j.earscirev.2011.02.003
; Archean reference slope, grey dashed line) and the early solar nebula (e.g., Antonelli et al., 2014Antonelli, M.A., Kim, S.-T., Peters, M., Labidi, J., Cartigny, P., et al. (2014) Early inner solar system origin for anomalous sulfur isotopes in differentiated protoplanets. Proceedings of the National Academy of Sciences 111, 17749–17754. https://doi.org/10.1073/pnas.1418907111
; Lyman-alpha photolysis, black dashed line).75081, 690 preserves a MIF-S signature. Mass independent isotope effects most commonly arise in gas phase reactions in the presence of UV light because the lifetimes of excited state molecules allow for other isotopically selective factors to come into play (Okabe, 1978
Okabe, H. (1978) Photochemistry of small molecules. Wiley, New York.
) and thus, could have occurred in the lunar atmosphere throughout its evolution. Global and local transient lunar atmospheres may have been produced early (prior to 3.0 Ga) in lunar history through volcanic eruptions and large impact events (Needham and Kring, 2017Needham, D.H., Kring, D.A. (2017) Lunar volcanism produced a transient atmosphere around the ancient Moon. Earth and Planetary Science Letters 478, 175–178. https://doi.org/10.1016/j.epsl.2017.09.002
; Aleinov et al., 2019Aleinov, I., Way, M.J., Harman, C., Tsigaridis, K., Wolf, E.T., Gronoff, G. (2019) Modeling a Transient Secondary Paleolunar Atmosphere: 3-D Simulations and Analysis. Geophysical Research Letters 46, 5107–5116. https://doi.org/10.1029/2019GL082494
; Head et al., 2020Head, J.W., Wilson, L., Deutsch, A.N., Rutherford, M.J., Saal, A.E. (2020) Volcanically Induced Transient Atmospheres on the Moon: Assessment of Duration, Significance, and Contributions to Polar Volatile Traps. Geophysical Research Letters 47, e2020GL089509. https://doi.org/10.1029/2020GL089509
). Due to the thin nature of these atmospheres that allows ultraviolet light to penetrate, one can hypothesise that MIF-S could occur in these environments and impact the sulfur isotope composition observed in the lunar soils. For large scale transient atmospheres produced by volcanism and impact events, one would expect MIF-S signatures to be ubiquitous among lunar surface materials; however, unambiguous evidence for photochemically derived MIF-S (Δ33S ≠ 0) has not been observed in any other lunar materials (Thode and Rees, 1979Thode, H.G., Rees, C.E. (1979) Sulphur isotopes in lunar and meteorite samples. Proceedings of the Tenth Lunar and Planetary Science Conference, 1629–1636. https://articles.adsabs.harvard.edu/pdf/1979LPSC...10.1629T
; Wing and Farquhar, 2015Wing, B.A., Farquhar, J. (2015) Sulfur isotope homogeneity of lunar mare basalts. Geochimica et Cosmochimica Acta 170, 266–280. https://doi.org/10.1016/j.gca.2015.09.003
). Furthermore, lunar soil production poses a problem for capturing MIF-S from large scale photochemical events: the isotopic composition should homogenise overtime as gardening occurs (i.e. micrometeorite bombardment and solar wind sputtering). Although both samples share the positive δ34S signature associated with sulfur loss during gardening (Thode and Rees, 1976Thode, H.G., Rees, C.E. (1976) Sulphur isotopes in grain size fractions of lunar soils. Proceedings of the Seventh Lunar Science Conference, 459–468. https://articles.adsabs.harvard.edu/pdf/1976LPSC....7..459T
) (Fig. 1), the MIF signature in 75081, 690 overprints the gardening signature and requires MIF-S to have occurred after or during lunar gardening.75081, 690 shows a relationship between δ34S and Δ33S that links the negative Δ33S sulfur to the condensed outer layer material (e.g., Keller and McKay, 1997
Keller, L.P., McKay, D.S. (1997) The nature and origin of rims on lunar soil grains. Geochimica et Cosmochimica Acta 61, 2331–2341. https://doi.org/10.1016/S0016-7037(97)00085-9
, and references within). Effects related to surface/volume ratios result in the strongest negative Δ33S signal seen in the smallest grain size (Fig. 1). Therefore, our observed isotopic signatures are a mixture between the condensed sulfur layer and the indigenous sulfur of the soil grain.Production of the strongly negative Δ33S of the outer layer sulfur associated with 75081, 690 requires a process that does not follow canonical mass dependence (i.e. mass independent). Thus, the associated process is separate from any process associated with sulfur loss during lunar volatilisation processes, which are thought to be strictly mass dependent and only produce variations in δ34S measurements (e.g., Thode and Rees, 1976
Thode, H.G., Rees, C.E. (1976) Sulphur isotopes in grain size fractions of lunar soils. Proceedings of the Seventh Lunar Science Conference, 459–468. https://articles.adsabs.harvard.edu/pdf/1976LPSC....7..459T
). Evidence of such is seen in our analyses of 74241, 204 (immature) that preserve mass dependent (i.e. near-zero) Δ33S and Δ36S, but variable δ34S, supporting a strict mass dependent isotope fractionation associated with sulfur loss.While the exact origin of the variations in Δ33S and Δ36S values in 75081, 690 is not clear, it appears to be different from the shared 34S enrichment with 74241, 204, and likely originates from photolytic reactions of S-bearing gaseous molecular species, such as S, SO, SO2, H2S, and HS. The components of the soils are ancient (Goswami and Lal, 1974
Goswami, J.N., Lal, D. (1974) Cosmic ray irradiation pattern at the Apollo 17 site: Implications to lunar regolith dynamics. Proceedings of the Fifth Lunar Conference 3, 2643–2662. https://articles.adsabs.harvard.edu/pdf/1974LPSC....5.2643G
), and based on 40Ar/36Ar trapped for 74241 (7.4) compared to 75081 (0.7), 74241 may have last been exposed to space weathering at 3.13 Ga compared to 0.25 Ga for 75081 (e.g., Curran et al., 2020Curran, N.M., Nottingham, M., Alexander, L., Crawford, I.A., Füri, E., Joy, K.H. (2020) A database of noble gases in lunar samples in preparation for mass spectrometry on the Moon. Planetary and Space Science 182, 104823. https://doi.org/10.1016/j.pss.2019.104823
) which suggests either MIF-S is not linked to processes occurring >3.0 Ga or length of exposure to space weathering is critical for MIF-S production. Although extra-lunar sulfur is thought to contribute to the total sulfur observed in soils (Kerridge et al., 1975Kerridge, J.F., Kaplan, I.R., Petrowski, C. (1975) Evidence for meteoritic sulfur in the lunar regolith. Proceedings of the Sixth Lunar Science Conference, 2151–2162. https://articles.adsabs.harvard.edu/pdf/1975LPSC....6.2151K
; Thode and Rees, 1979Thode, H.G., Rees, C.E. (1979) Sulphur isotopes in lunar and meteorite samples. Proceedings of the Tenth Lunar and Planetary Science Conference, 1629–1636. https://articles.adsabs.harvard.edu/pdf/1979LPSC...10.1629T
), our data are not consistent with acquisition of the MIF-S signature from these sources: the sulfur isotope compositions observed in the meteorite record (Antonelli et al., 2014Antonelli, M.A., Kim, S.-T., Peters, M., Labidi, J., Cartigny, P., et al. (2014) Early inner solar system origin for anomalous sulfur isotopes in differentiated protoplanets. Proceedings of the National Academy of Sciences 111, 17749–17754. https://doi.org/10.1073/pnas.1418907111
; Labidi et al., 2017Labidi, J., Farquhar, J., Alexander, C.M.O’D., Eldridge, D.L., Oduro, H. (2017) Mass independent sulfur isotope signatures in CMs: Implications for sulfur chemistry in the early solar system. Geochimica et Cosmochimica Acta 196, 326–350. https://doi.org/10.1016/j.gca.2016.09.036
; Dottin et al., 2018Dottin III, J.W., Farquhar, J., Labidi, J. (2018) Multiple sulfur isotopic composition of main group pallasites support genetic links to IIIAB iron meteorites. Geochimica et Cosmochimica Acta 224, 276–281. https://doi.org/10.1016/j.gca.2018.01.013
; Wu et al., 2018Wu, N., Farquhar, J., Dottin III, J.W., Magalhães, N. (2018) Sulfur isotope signatures of eucrites and diogenites. Geochimica et Cosmochimica Acta 233, 1–13. https://doi.org/10.1016/j.gca.2018.05.002
, and references within) do not match our observations. We also exclude MIF-S acquisition from large scale transient atmospheres and sputtering due to the ubiquitous lack of MIF-S signatures among lunar materials: spallation yields are low and require Fe and low sulfur contents of a metal phase to observe evidence for spallation reactions (Gao and Thiemens, 1991Gao, X., Thiemens, M.H. (1991) Systematic study of sulfur isotopic composition in iron meteorites and the occurrence of excess 33S and 36S. Geochimica et Cosmochimica Acta 55, 2671–2679. https://doi.org/10.1016/0016-7037(91)90381-E
). Thus, we suggest the most parsimonious explanation for acquisition of MIF-S in 75081, 690 is linked to gardening events that volatilise sulfur that undergoes UV photochemistry while in the lunar atmosphere (Fig. 2).
Figure 2 Hypothesis for the origin of sulfur isotope variations in lunar soils. (a) Micrometeorite impacts result in volatilisation and loss of sulfur at the edge of grains. (b) Vapourised sulfur is added to the tenuous lunar atmosphere and travels up to 3000 km before condensing. Here, gaseous sulfur molecules can escape, which induces mass dependent 34S enrichments, and they can undergo photolytic reactions, resulting in mass independent fractionation (MIF) (seen in 75081, 690). (c) Vapour condenses on regolith soil with isotopic evidence of how sample was processed.
Assuming the MIF-S observed in 75081, 690 is indeed linked to gardening events, the dichotomy in Δ33S and Δ36S between 75081, 690 and 74241, 204 may reflect (1) differences in the nature of the target relative to sample maturity (also related to timing of exposure at the lunar surface), and/or (2) the processing of volatilised sulfur species in regions with or without sunlight. Mature targets that have more implanted hydrogen from solar wind may have a greater chance for formation of H-bearing gaseous sulfur species that promote photochemical MIF-S. The production of H-bearing gaseous sulfur species would require a more local, rather than regional or global, process to generate the variation observed between sites, and the MIF-S likely represents an accumulated fractionation from consistent gardening events. This process would be widespread, and in future measurements of lunar soils, the MIF-S signature should be observed. Literature analyses by Thode and Rees
Thode, H.G., Rees, C.E. (1979) Sulphur isotopes in lunar and meteorite samples. Proceedings of the Tenth Lunar and Planetary Science Conference, 1629–1636. https://articles.adsabs.harvard.edu/pdf/1979LPSC...10.1629T
(1979Thode, H.G., Rees, C.E. (1979) Sulphur isotopes in lunar and meteorite samples. Proceedings of the Tenth Lunar and Planetary Science Conference, 1629–1636. https://articles.adsabs.harvard.edu/pdf/1979LPSC...10.1629T
) of size fractions from sample 15021 may also show non-zero Δ33S (Fig. S-6) and be broadly consistent with our results. However, the data have been held up as an example of mass dependent isotope effects due to analytical uncertainty. Processing environment of the soils is important to consider because MIF-S via photochemistry requires sunlight. The difference in Δ33S and Δ36S between 75081, 690 and 74241, 204 may reflect processing in sunlit and shadowed parts of the Moon, but such a scenario is difficult to reconcile considering both of our studied sites are on the near side of the Moon and likely share a similar history of illumination.Missing sulfur reservoir. Δ33S in 75081, 690 is consistently negative and presents an issue of mass balance (i.e. a reservoir of sulfur with positive Δ33S is missing). The sulfur with positive Δ33S may have been lost to space, trapped in Permanently Shadowed Regions (PSRs) (Watson et al., 1961
Watson, K., Murray, B.C., Brown, H. (1961) The behavior of volatiles on the lunar surface. Journal of Geophysical Research 66, 3033–3045. https://doi.org/10.1029/JZ066i009p03033
), or trapped in micro cold traps of a nearby crater (Hayne et al., 2021Hayne, P.O., Aharonson, O., Schörghofer, N. (2021) Micro cold traps on the Moon. Nature Astronomy 5, 169–175. https://doi.org/10.1038/s41550-020-1198-9
). As volatile deposits are identified and explored in the upcoming Artemis missions, Δ33S measurements of returned samples can be potentially used (1) to better understand the volatile cycle on the Moon and the transport of volatiles across the lunar surface, and (2) as a fingerprint for identifying evolving PSRs, such as through measurements of Δ33S from a core collected from a PSR.Links among δ34S, sulfur concentration, and grain size. Successfully linking the observed MIF-S signature to UV photolysis of volatiles during lunar gardening events is contingent upon a model that can also explain the observed δ34S and sulfur concentrations of various grain size fractions from 74241, 204 and 75081, 690.
The δ34S values and sulfur concentrations of various grain size fractions could be explained by a grain margin subject to diffusive sulfur loss from the inner grain prior to addition of a condensed layer (Fig. S-7) (Saal et al., 2008
Saal, A.E., Hauri, E.H., Cascio, M.L., Van Orman, J.A., Rutherford, M.C., Cooper, R.F. (2008) Volatile content of lunar volcanic glasses and the presence of water in the Moon’s interior. Nature 454, 192–195. https://doi.org/10.1038/nature07047
). The diffusion model would, however, require diffusion times and/or temperatures that are too long/high to fit the standard understanding of micrometeorite gardening (see Supplementary Information S-2). The data can also be explained with a model involving a degassed melted layer with no isotope fractionation that sits between a homogenous inner grain and an outer isotopically fractionated condensed layer (see Supplementary Information S-2). This model satisfies our observations while relaxing the time/temperature constraints. While various explanations have been proposed to explain the 34S enrichment of the condensed outer layer sulfur (e.g., Clayton et al., 1974Clayton, R.N., Mayeda, T.K., Hurd, J.M. (1974) Loss of oxygen, silicon, sulfur, and potassium from the lunar regolith. Proceedings of the Fifth Lunar Conference 2, 1801–1809. https://articles.adsabs.harvard.edu/pdf/1974LPSC....5.1801C
; Ding et al., 1983Ding, T.P., Thode, H.G., Rees, C.E. (1983) Sulphur content and sulphur isotope composition of orange and black glasses in Apollo 17 drive tube 74002/1. Geochimica et Cosmochimica Acta 47, 491–496. https://doi.org/10.1016/0016-7037(83)90271-5
; Kerridge and Kaplan, 1978Kerridge, J.F., Kaplan, I.R. (1978) Sputtering: Its relationship to isotopic fractionation on the lunar surface. Proceedings of the Ninth Lunar and Planetary Science Conference, 1687–1709. https://articles.adsabs.harvard.edu/pdf/1978LPSC....9.1687K
), given the observed MIF-S in 75081, 690, the most parsimonious explanation is linked to condensed sulfur fractionated by atmospheric escape (e.g., Clayton et al., 1974Clayton, R.N., Mayeda, T.K., Hurd, J.M. (1974) Loss of oxygen, silicon, sulfur, and potassium from the lunar regolith. Proceedings of the Fifth Lunar Conference 2, 1801–1809. https://articles.adsabs.harvard.edu/pdf/1974LPSC....5.1801C
; Switkowski et al., 1977Switkowski, Z.E., Haff, P.K., Tombrello, T.A., Burnett, D.S. (1977) Mass fractionation of the lunar surface by solar wind sputtering. Journal of Geophysical Research: Solid Earth and Planets 82, 3797–3804. https://doi.org/10.1029/JB082i026p03797
; see Supplementary Information S-2).top
Conclusions
We present isotopic evidence that mass independently fractionated sulfur condensed onto lunar soil grains associated with 75081, 690. As illustrated in Figure 2, we hypothesise that sulfur from both soils underwent atmospheric escape to space, producing 34S enrichments. Although 75081, 690 and 74241, 204 share 34S enrichments, the same mass independent signal is not observed in 74241, 204. We suggest that sulfur with MIF that later condensed on 75081, 690 was produced during UV photochemistry in the tenuous lunar atmosphere after sulfur without MIF was volatilised during gardening events. The lack of MIF-S in 74241, 204 may be linked to (1) lower amounts of solar wind implanted hydrogen that can be readily available to form H-bearing sulfur species that undergo photochemistry, and/or (2) processing in a shaded environment.
top
Acknowledgments
We thank Astromaterials Acquisition and Curation (NASA JSC) for granting samples. JD acknowledges the NSF EAR postdoctoral fellowship for salary support while writing this manuscript. We lastly thank Romain Tartèse and one anonymous reviewer for their thoughtful comments that helped improve the quality of our manuscript.
Editor: Maud Boyet
top
References
Aleinov, I., Way, M.J., Harman, C., Tsigaridis, K., Wolf, E.T., Gronoff, G. (2019) Modeling a Transient Secondary Paleolunar Atmosphere: 3-D Simulations and Analysis. Geophysical Research Letters 46, 5107–5116. https://doi.org/10.1029/2019GL082494

Global and local transient lunar atmospheres may have been produced early (prior to 3.0 Ga) in lunar history through volcanic eruptions and large impact events (Needham and Kring, 2017; Aleinov et al., 2019; Head et al., 2020).
View in article
Antonelli, M.A., Kim, S.-T., Peters, M., Labidi, J., Cartigny, P., et al. (2014) Early inner solar system origin for anomalous sulfur isotopes in differentiated protoplanets. Proceedings of the National Academy of Sciences 111, 17749–17754. https://doi.org/10.1073/pnas.1418907111

In (b), we highlight that data from 75081, 690 indicates the photochemistry occurring is different from that in other planetary environments, such as early Earth (e.g., Johnston, 2011; Archean reference slope, grey dashed line) and the early solar nebula (e.g., Antonelli et al., 2014; Lyman-alpha photolysis, black dashed line).
View in article
Although extra-lunar sulfur is thought to contribute to the total sulfur observed in soils (Kerridge et al., 1975; Thode and Rees, 1979), our data are not consistent with acquisition of the MIF-S signature from these sources: the sulfur isotope compositions observed in the meteorite record (Antonelli et al., 2014; Labidi et al., 2017; Dottin et al., 2018; Wu et al., 2018, and references within) do not match our observations.
View in article
Clayton, R.N., Mayeda, T.K., Hurd, J.M. (1974) Loss of oxygen, silicon, sulfur, and potassium from the lunar regolith. Proceedings of the Fifth Lunar Conference 2, 1801–1809. https://articles.adsabs.harvard.edu/pdf/1974LPSC....5.1801C

While various explanations have been proposed to explain the 34S enrichment of the condensed outer layer sulfur (e.g., Clayton et al., 1974; Ding et al., 1983; Kerridge and Kaplan, 1978), given the observed MIF-S in 75081, 690, the most parsimonious explanation is linked to condensed sulfur fractionated by atmospheric escape (e.g., Clayton et al., 1974; Switkowski et al., 1977; see Supplementary Information S-2).
View in article
While various explanations have been proposed to explain the 34S enrichment of the condensed outer layer sulfur (e.g., Clayton et al., 1974; Ding et al., 1983; Kerridge and Kaplan, 1978), given the observed MIF-S in 75081, 690, the most parsimonious explanation is linked to condensed sulfur fractionated by atmospheric escape (e.g., Clayton et al., 1974; Switkowski et al., 1977; see Supplementary Information S-2).
View in article
Curran, N.M., Nottingham, M., Alexander, L., Crawford, I.A., Füri, E., Joy, K.H. (2020) A database of noble gases in lunar samples in preparation for mass spectrometry on the Moon. Planetary and Space Science 182, 104823. https://doi.org/10.1016/j.pss.2019.104823

The components of the soils are ancient (Goswami and Lal, 1974), and based on 40Ar/36Ar trapped for 74241 (7.4) compared to 75081 (0.7), 74241 may have last been exposed to space weathering at 3.13 Ga compared to 0.25 Ga for 75081 (e.g., Curran et al., 2020) which suggests either MIF-S is not linked to processes occurring >3.0 Ga or length of exposure to space weathering is critical for MIF-S production.
View in article
Ding, T.P., Thode, H.G., Rees, C.E. (1983) Sulphur content and sulphur isotope composition of orange and black glasses in Apollo 17 drive tube 74002/1. Geochimica et Cosmochimica Acta 47, 491–496. https://doi.org/10.1016/0016-7037(83)90271-5

While various explanations have been proposed to explain the 34S enrichment of the condensed outer layer sulfur (e.g., Clayton et al., 1974; Ding et al., 1983; Kerridge and Kaplan, 1978), given the observed MIF-S in 75081, 690, the most parsimonious explanation is linked to condensed sulfur fractionated by atmospheric escape (e.g., Clayton et al., 1974; Switkowski et al., 1977; see Supplementary Information S-2).
View in article
Dottin III, J.W., Farquhar, J., Labidi, J. (2018) Multiple sulfur isotopic composition of main group pallasites support genetic links to IIIAB iron meteorites. Geochimica et Cosmochimica Acta 224, 276–281. https://doi.org/10.1016/j.gca.2018.01.013

Although extra-lunar sulfur is thought to contribute to the total sulfur observed in soils (Kerridge et al., 1975; Thode and Rees, 1979), our data are not consistent with acquisition of the MIF-S signature from these sources: the sulfur isotope compositions observed in the meteorite record (Antonelli et al., 2014; Labidi et al., 2017; Dottin et al., 2018; Wu et al., 2018, and references within) do not match our observations.
View in article
Farquhar, J., Bao, H., Thiemens, M. (2000) Atmospheric Influence of Earth’s Earliest Sulfur Cycle. Science 289, 756–758. https://doi.org/10.1126/science.289.5480.756

The earliest atmospheres on Earth and Mars were optically thin and contained sufficient sulfur-bearing gaseous molecules and penetration of ultraviolet (UV) light that generated mass independent fractionation of sulfur (MIF-S) isotopes (e.g., Farquhar et al., 2000; Franz et al., 2014).
View in article
Franz, H.B., Kim, S.-T., Farquhar, J., Day, J.M.D., Economos, R.C., et al. (2014) Isotopic links between atmospheric chemistry and the deep sulphur cycle on Mars. Nature 508, 364–368. https://doi.org/10.1038/nature13175

The earliest atmospheres on Earth and Mars were optically thin and contained sufficient sulfur-bearing gaseous molecules and penetration of ultraviolet (UV) light that generated mass independent fractionation of sulfur (MIF-S) isotopes (e.g., Farquhar et al., 2000; Franz et al., 2014).
View in article
Gao, X., Thiemens, M.H. (1991) Systematic study of sulfur isotopic composition in iron meteorites and the occurrence of excess 33S and 36S. Geochimica et Cosmochimica Acta 55, 2671–2679. https://doi.org/10.1016/0016-7037(91)90381-E

We also exclude MIF-S acquisition from large scale transient atmospheres and sputtering due to the ubiquitous lack of MIF-S signatures among lunar materials: spallation yields are low and require Fe and low sulfur contents of a metal phase to observe evidence for spallation reactions (Gao and Thiemens, 1991).
View in article
Gargano, A., Dottin, J., Hopkins, S.S., Sharp, Z., Shearer, C.K., et al. (2022) The Zn, S, and Cl isotope compositions of mare basalts: implications for the effects of eruption style and pressure on volatile element stable isotope fractionation on the Moon. American Mineralogist, in press. https://doi.org/10.2138/am-2022-8290
Goswami, J.N., Lal, D. (1974) Cosmic ray irradiation pattern at the Apollo 17 site: Implications to lunar regolith dynamics. Proceedings of the Fifth Lunar Conference 3, 2643–2662. https://articles.adsabs.harvard.edu/pdf/1974LPSC....5.2643G

The components of the soils are ancient (Goswami and Lal, 1974), and based on 40Ar/36Ar trapped for 74241 (7.4) compared to 75081 (0.7), 74241 may have last been exposed to space weathering at 3.13 Ga compared to 0.25 Ga for 75081 (e.g., Curran et al., 2020) which suggests either MIF-S is not linked to processes occurring >3.0 Ga or length of exposure to space weathering is critical for MIF-S production.
View in article
Hayne, P.O., Aharonson, O., Schörghofer, N. (2021) Micro cold traps on the Moon. Nature Astronomy 5, 169–175. https://doi.org/10.1038/s41550-020-1198-9

The sulfur with positive Δ33S may have been lost to space, trapped in Permanently Shadowed Regions (PSRs) (Watson et al., 1961), or trapped in micro cold traps of a nearby crater (Hayne et al., 2021).
View in article
Head, J.W., Wilson, L., Deutsch, A.N., Rutherford, M.J., Saal, A.E. (2020) Volcanically Induced Transient Atmospheres on the Moon: Assessment of Duration, Significance, and Contributions to Polar Volatile Traps. Geophysical Research Letters 47, e2020GL089509. https://doi.org/10.1029/2020GL089509

Global and local transient lunar atmospheres may have been produced early (prior to 3.0 Ga) in lunar history through volcanic eruptions and large impact events (Needham and Kring, 2017; Aleinov et al., 2019; Head et al., 2020).
View in article
Johnston, D.T. (2011) Multiple sulfur isotopes and the evolution of Earth’s surface sulfur cycle. Earth-Science Reviews 106, 161–183. https://doi.org/10.1016/j.earscirev.2011.02.003

In (b), we highlight that data from 75081, 690 indicates the photochemistry occurring is different from that in other planetary environments, such as early Earth (e.g., Johnston, 2011; Archean reference slope, grey dashed line) and the early solar nebula (e.g., Antonelli et al., 2014; Lyman-alpha photolysis, black dashed line).
View in article
Keller, L.P., McKay, D.S. (1997) The nature and origin of rims on lunar soil grains. Geochimica et Cosmochimica Acta 61, 2331–2341. https://doi.org/10.1016/S0016-7037(97)00085-9

75081, 690 shows a relationship between δ34S and Δ33S that links the negative Δ33S sulfur to the condensed outer layer material (e.g., Keller and McKay, 1997, and references within). Effects related to surface/volume ratios result in the strongest negative Δ33S signal seen in the smallest grain size (Fig. 1).
View in article
Kerridge, J.F., Kaplan, I.R. (1978) Sputtering: Its relationship to isotopic fractionation on the lunar surface. Proceedings of the Ninth Lunar and Planetary Science Conference, 1687–1709. https://articles.adsabs.harvard.edu/pdf/1978LPSC....9.1687K

While various explanations have been proposed to explain the 34S enrichment of the condensed outer layer sulfur (e.g., Clayton et al., 1974; Ding et al., 1983; Kerridge and Kaplan, 1978), given the observed MIF-S in 75081, 690, the most parsimonious explanation is linked to condensed sulfur fractionated by atmospheric escape (e.g., Clayton et al., 1974; Switkowski et al., 1977; see Supplementary Information S-2).
View in article
Kerridge, J.F., Kaplan, I.R., Petrowski, C. (1975) Evidence for meteoritic sulfur in the lunar regolith. Proceedings of the Sixth Lunar Science Conference, 2151–2162. https://articles.adsabs.harvard.edu/pdf/1975LPSC....6.2151K

Although extra-lunar sulfur is thought to contribute to the total sulfur observed in soils (Kerridge et al., 1975; Thode and Rees, 1979), our data are not consistent with acquisition of the MIF-S signature from these sources: the sulfur isotope compositions observed in the meteorite record (Antonelli et al., 2014; Labidi et al., 2017; Dottin et al., 2018; Wu et al., 2018, and references within) do not match our observations.
View in article
Labidi, J., Farquhar, J., Alexander, C.M.O’D., Eldridge, D.L., Oduro, H. (2017) Mass independent sulfur isotope signatures in CMs: Implications for sulfur chemistry in the early solar system. Geochimica et Cosmochimica Acta 196, 326–350. https://doi.org/10.1016/j.gca.2016.09.036

Although extra-lunar sulfur is thought to contribute to the total sulfur observed in soils (Kerridge et al., 1975; Thode and Rees, 1979), our data are not consistent with acquisition of the MIF-S signature from these sources: the sulfur isotope compositions observed in the meteorite record (Antonelli et al., 2014; Labidi et al., 2017; Dottin et al., 2018; Wu et al., 2018, and references within) do not match our observations.
View in article
Morris, R.V. (1978) The surface exposure (maturity) of lunar soils: Some concepts and Is/FeO compilation. Proceedings of the Ninth Lunar and Planetary Science Conference, 2287–2297. https://articles.adsabs.harvard.edu/pdf/1978LPSC....9.2287M

We present new analyses of the quadruple sulfur isotope compositions and sulfur concentrations for 9 and 10 size fractions (<10 to >500 μm and >1000 μm) from lunar basaltic regolith samples 74241, 204 (immature, Is/FeO = 5.1) and 75081, 690 (sub-mature, Is/FeO = 40) (Morris, 1978) (Table S-1).
View in article
Needham, D.H., Kring, D.A. (2017) Lunar volcanism produced a transient atmosphere around the ancient Moon. Earth and Planetary Science Letters 478, 175–178. https://doi.org/10.1016/j.epsl.2017.09.002

Global and local transient lunar atmospheres may have been produced early (prior to 3.0 Ga) in lunar history through volcanic eruptions and large impact events (Needham and Kring, 2017; Aleinov et al., 2019; Head et al., 2020).
View in article
The early evolution of the Moon (3.8–3.1 Ga) included pyroclastic and effusive volcanism, and large impact events that provided enough gas to produce optically thin transient atmospheres (e.g., Prem et al., 2015; Needham and Kring, 2017) where UV light can penetrate and produce MIF-S.
View in article
Okabe, H. (1978) Photochemistry of small molecules. Wiley, New York.

Mass independent isotope effects most commonly arise in gas phase reactions in the presence of UV light because the lifetimes of excited state molecules allow for other isotopically selective factors to come into play (Okabe, 1978) and thus, could have occurred in the lunar atmosphere throughout its evolution.
View in article
Prem, P., Artemieva, N.A., Goldstein, D.B., Varghese, P.L., Trafton, L.M. (2015) Transport of water in a transient impact-generated lunar atmosphere. Icarus 255, 148–158. https://doi.org/10.1016/j.icarus.2014.10.017

The early evolution of the Moon (3.8–3.1 Ga) included pyroclastic and effusive volcanism, and large impact events that provided enough gas to produce optically thin transient atmospheres (e.g., Prem et al., 2015; Needham and Kring, 2017) where UV light can penetrate and produce MIF-S.
View in article
Saal, A.E., Hauri, E.H., Cascio, M.L., Van Orman, J.A., Rutherford, M.C., Cooper, R.F. (2008) Volatile content of lunar volcanic glasses and the presence of water in the Moon’s interior. Nature 454, 192–195. https://doi.org/10.1038/nature07047

The δ34S values and sulfur concentrations of various grain size fractions could be explained by a grain margin subject to diffusive sulfur loss from the inner grain prior to addition of a condensed layer (Fig. S-7) (Saal et al., 2008).
View in article
Switkowski, Z.E., Haff, P.K., Tombrello, T.A., Burnett, D.S. (1977) Mass fractionation of the lunar surface by solar wind sputtering. Journal of Geophysical Research: Solid Earth and Planets 82, 3797–3804. https://doi.org/10.1029/JB082i026p03797

While various explanations have been proposed to explain the 34S enrichment of the condensed outer layer sulfur (e.g., Clayton et al., 1974; Ding et al., 1983; Kerridge and Kaplan, 1978), given the observed MIF-S in 75081, 690, the most parsimonious explanation is linked to condensed sulfur fractionated by atmospheric escape (e.g., Clayton et al., 1974; Switkowski et al., 1977; see Supplementary Information S-2).
View in article
Thode, H.G., Rees, C.E. (1976) Sulphur isotopes in grain size fractions of lunar soils. Proceedings of the Seventh Lunar Science Conference, 459–468. https://articles.adsabs.harvard.edu/pdf/1976LPSC....7..459T

Although both samples share the positive δ34S signature associated with sulfur loss during gardening (Thode and Rees, 1976) (Fig. 1), the MIF signature in 75081, 690 overprints the gardening signature and requires MIF-S to have occurred after or during lunar gardening.
View in article
Thus, the associated process is separate from any process associated with sulfur loss during lunar volatilisation processes, which are thought to be strictly mass dependent and only produce variations in δ34S measurements (e.g., Thode and Rees, 1976).
View in article
Thode, H.G., Rees, C.E. (1979) Sulphur isotopes in lunar and meteorite samples. Proceedings of the Tenth Lunar and Planetary Science Conference, 1629–1636. https://articles.adsabs.harvard.edu/pdf/1979LPSC...10.1629T

For large scale transient atmospheres produced by volcanism and impact events, one would expect MIF-S signatures to be ubiquitous among lunar surface materials; however, unambiguous evidence for photochemically derived MIF-S (Δ33S ≠ 0) has not been observed in any other lunar materials (Thode and Rees, 1979; Wing and Farquhar, 2015).
View in article
Literature analyses by Thode and Rees (1979) of size fractions from sample 15021 may also show non-zero Δ33S (Fig. S-6) and be broadly consistent with our results.
View in article
Although extra-lunar sulfur is thought to contribute to the total sulfur observed in soils (Kerridge et al., 1975; Thode and Rees, 1979), our data are not consistent with acquisition of the MIF-S signature from these sources: the sulfur isotope compositions observed in the meteorite record (Antonelli et al., 2014; Labidi et al., 2017; Dottin et al., 2018; Wu et al., 2018, and references within) do not match our observations.
View in article
Watson, K., Murray, B.C., Brown, H. (1961) The behavior of volatiles on the lunar surface. Journal of Geophysical Research 66, 3033–3045. https://doi.org/10.1029/JZ066i009p03033

The sulfur with positive Δ33S may have been lost to space, trapped in Permanently Shadowed Regions (PSRs) (Watson et al., 1961), or trapped in micro cold traps of a nearby crater (Hayne et al., 2021).
View in article
Wing, B.A., Farquhar, J. (2015) Sulfur isotope homogeneity of lunar mare basalts. Geochimica et Cosmochimica Acta 170, 266–280. https://doi.org/10.1016/j.gca.2015.09.003

For large scale transient atmospheres produced by volcanism and impact events, one would expect MIF-S signatures to be ubiquitous among lunar surface materials; however, unambiguous evidence for photochemically derived MIF-S (Δ33S ≠ 0) has not been observed in any other lunar materials (Thode and Rees, 1979; Wing and Farquhar, 2015).
View in article
Wu, N., Farquhar, J., Dottin III, J.W., Magalhães, N. (2018) Sulfur isotope signatures of eucrites and diogenites. Geochimica et Cosmochimica Acta 233, 1–13. https://doi.org/10.1016/j.gca.2018.05.002

Although extra-lunar sulfur is thought to contribute to the total sulfur observed in soils (Kerridge et al., 1975; Thode and Rees, 1979), our data are not consistent with acquisition of the MIF-S signature from these sources: the sulfur isotope compositions observed in the meteorite record (Antonelli et al., 2014; Labidi et al., 2017; Dottin et al., 2018; Wu et al., 2018, and references within) do not match our observations.
View in article
top
Supplementary Information
The Supplementary Information includes:
Download the Supplementary Information (PDF)
Figures

Figure 1 (a) Δ33S vs. δ34S and (b) Δ36S vs. Δ33S of analysed lunar soils 75081, 690 (red) and 74241, 204 (blue) and literature data from lunar basalts (grey). In (b), we highlight that data from 75081, 690 indicates the photochemistry occurring is different from that in other planetary environments, such as early Earth (e.g., Johnston, 2011
Johnston, D.T. (2011) Multiple sulfur isotopes and the evolution of Earth’s surface sulfur cycle. Earth-Science Reviews 106, 161–183. https://doi.org/10.1016/j.earscirev.2011.02.003
; Archean reference slope, grey dashed line) and the early solar nebula (e.g., Antonelli et al., 2014Antonelli, M.A., Kim, S.-T., Peters, M., Labidi, J., Cartigny, P., et al. (2014) Early inner solar system origin for anomalous sulfur isotopes in differentiated protoplanets. Proceedings of the National Academy of Sciences 111, 17749–17754. https://doi.org/10.1073/pnas.1418907111
; Lyman-alpha photolysis, black dashed line).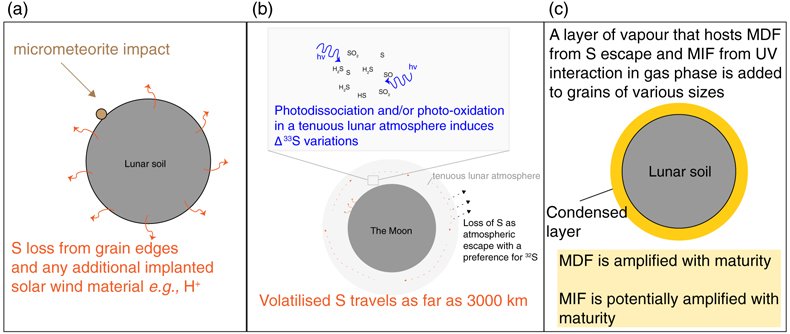
Figure 2 Hypothesis for the origin of sulfur isotope variations in lunar soils. (a) Micrometeorite impacts result in volatilisation and loss of sulfur at the edge of grains. (b) Vapourised sulfur is added to the tenuous lunar atmosphere and travels up to 3000 km before condensing. Here, gaseous sulfur molecules can escape, which induces mass dependent 34S enrichments, and they can undergo photolytic reactions, resulting in mass independent fractionation (MIF) (seen in 75081, 690). (c) Vapour condenses on regolith soil with isotopic evidence of how sample was processed.