The Solar System calcium isotopic composition inferred from Ryugu samples
Affiliations | Corresponding Author | Cite as | Funding information- Share this article
Article views:176Cumulative count of HTML views and PDF downloads.
- Download Citation
- Rights & Permissions
top
Abstract

Figures and Tables
![]() Figure 1 The δ44/42Ca values plotted against δ44/40Ca values for the various samples analysed in this study, including the Ryugu samples. All the samples fall on a mass dependent line within error. Error bars represent 2 sigma standard deviation. | ![]() Figure 2 A comparison of age corrected δ44/40Ca values for the samples analysed here (in colour, see Fig. 1) and from the literature (grey). Ryugu samples from Chambers A and C are similar within error and fall within the range defined by the CI chondrites. The literature data are from Table 1, BSE estimate from Kang et al. (2017) and chondrules data from Amsellem et al. (2017). Error bars are 2 x standard deviation. | ![]() Figure 3 δ44/40Ca plotted against the Ca/Al ratio of the samples. The Ca/Al ratios of bulk Chamber A and Chamber C samples from Yokoyama et al. (2022) are used for Ryugu samples in this work and are taken from the average value in Barrat et al. (2012) for Orgueil, as it was not available for specific samples used here. | ![]() Table 1 Calcium isotopic data from this study and literature (Simon and DePaolo, 2010; Valdes et al., 2014; Amsellem et al., 2017; Huang and Jacobsen, 2017). 2 s.d. = 2 x standard deviation and 2 s.e. = 2 x standard error (2sd/√n). n is number of measurements. |
Figure 1 | Figure 2 | Figure 3 | Table 1 |
top
Introduction
In December 2020, the JAXA Hayabusa2 spacecraft returned to Earth with the first samples collected from a Cb-type asteroid, (162173) Ryugu (Tachibana et al., 2022
Tachibana, S., Sawada, H., Okazaki, R., Takano, Y., Sakamoto, K. et al. (2022) Pebbles and sand on asteroid (162173) Ryugu: In situ observation and particles returned to Earth. Science 375, 1011–1016. https://doi.org/10.1126/science.abj8624
; Yada et al., 2022Yada, T., Abe, M., Okada, T., Nakato, A., Yogata, K. et al. (2022) Preliminary analysis of the Hayabusa2 samples returned from C-type asteroid Ryugu. Nature Astronomy 6, 214–220. https://doi.org/10.1038/s41550-021-01550-6
). Chemical, mineralogical, petrological, and isotopic analyses of these samples suggest that they are closely related to CI chondrites. In particular, their bulk Cr and Ti isotopic signatures and the chemical abundances of most elements are within the range of CI chondrites (Nakamura E. et al., 2022Nakamura, E., Kobayashi, K., Tanaka, R., Kunihiro, T., Kitagawa, H. et al. (2022) On the origin and evolution of the asteroid Ryugu: A comprehensive geochemical perspective. Proceedings of the Japan Academy, Series B. 6, 227–282. https://doi.org/10.2183/pjab.98.015
; Yokoyama et al., 2022Yokoyama, T., Nagashima, K., Nakai, I., Young, E.D., Abe, Y. et al. (2022) Samples returned from the asteroid Ryugu are similar to Ivuna-type carbonaceous meteorites. Science https://doi.org/10.1126/science.abn7850
). Among meteorites, CI chondrites have chemical compositions that most closely resemble the Sun; therefore, they are the most representative samples of the solar nebula composition with the exception of volatile elements (Palme et al., 2014Palme, H., Lodders, K., Jones, A. (2014) 2.2 - Solar System abundances of the elements. In: Holland, H.D., Turekian, K.K. (Exec. Eds.) Treatise on Geochemistry (Second Edition). Elsevier, Oxford, 15–36. https://doi.org/10.1016/B978-0-08-095975-7.00118-2
). Given that the Ryugu samples did not experience any terrestrial alteration, they are likely the chemically most pristine Solar System samples available estimating the original isotopic compositions of most elements in the Solar System (Yokoyama et al., 2022Yokoyama, T., Nagashima, K., Nakai, I., Young, E.D., Abe, Y. et al. (2022) Samples returned from the asteroid Ryugu are similar to Ivuna-type carbonaceous meteorites. Science https://doi.org/10.1126/science.abn7850
).Despite many aspects of similarities with the CIs, the Ryugu samples display an apparent excess of Ca by over 50 %, which may be related to a heterogeneous distribution of carbonates (dolomite and calcite) (Nakamura T. et al., 2022
Nakamura, T., Matsumoto, M., Amano, K., Enokido, Y., Zolensky, M. et al. (2022) Formation and evolution of carbonaceous asteroid Ryugu: Direct evidence from returned samples. Science. https://doi.org/10.1126/science.abn8671
; Yokoyama et al., 2022Yokoyama, T., Nagashima, K., Nakai, I., Young, E.D., Abe, Y. et al. (2022) Samples returned from the asteroid Ryugu are similar to Ivuna-type carbonaceous meteorites. Science https://doi.org/10.1126/science.abn7850
) between Ryugu, Orgueil and other CIs. Calcium is a major constituent of carbonates and can be isotopically fractionated during aqueous alteration and carbonate precipitation, leading to more than 1 ‰ variations in the 44Ca/40Ca ratio in terrestrial carbonates (e.g., Fantle and Tipper, 2014Fantle, M.S., Tipper, E.T. (2014) Calcium isotopes in the global biogeochemical Ca cycle: Implications for development of a Ca isotope proxy. Earth-Science Reviews 129, 148–177. https://doi.org/10.1016/j.earscirev.2013.10.004
; Blättler and Higgins, 2017Blättler, C., Higgins, J. (2017) Testing Urey’s carbonate–silicate cycle using the calcium isotopic composition of sedimentary carbonates. Earth and Planetary Science Letters 479, 241–251. https://doi.org/10.1016/j.epsl.2017.09.033
). In addition, Ca exhibits large isotopic variations among bulk carbonaceous chondrites (CC), with the 44Ca/40Ca ratio spanning a range of 1 ‰. This range is likely related to a combination of the variable modal abundances of refractory inclusions among CC (Hezel et al., 2008Hezel, DC., Russell, S.S., Ross, A.J., Kearsley, A.T. (2008) Modal Abundances of CAIs: Implications for bulk chondrite element abundances and fractionations. Meteoritics & Planetary Science 43, 1879–1894. https://doi.org/10.1111/j.1945-5100.2008.tb00649.x
) that can be enriched in the lighter Ca isotopes by several per mille (Niederer and Papanastassiou, 1984Niederer, F.R., Papanastassiou, D.A. (1984) Ca isotopes in refractory inclusions. Geochimica et Cosmochimica Acta 48, 1279–1293. https://doi.org/10.1016/0016-7037(84)90062-0
; Huang et al., 2012Huang, S., Farkas, J., Yu, G., Petaev, M.I., Jacobsen, S.B. (2012) Calcium isotopic ratios and rare earth elements abundances from refractory inclusions from the Allende CV3 chondrite. Geochimica et Cosmochimica Acta 77, 252–265. https://doi.org/10.1016/j.gca.2011.11.002
) and the heterogeneous distribution of carbonates (Simon and DePaolo, 2010Simon, J.I., DePaolo, D.J. (2010) Stable calcium isotopic composition of meteorites and rocky planets. Earth and Planetary Science Letters 289, 457–466. https://doi.org/10.1016/j.epsl.2009.11.035
; Valdes et al., 2014Valdes, M., Moreira, M., Foriel, J., Moynier, F. (2014) The nature of Earth’s building blocks as revealed by calcium isotopes. Earth and Planetary Science Letters 394, 135–145. https://doi.org/10.1016/j.epsl.2014.02.052
; Dauphas and Pourmand, 2015Dauphas, N., Pourmand, A. (2015) Thulium anomalies and rare earth element patterns in meteorites and Earth: Nebular fractionation and the nugget effect. Geochimica et Cosmochimica Acta 163, 234–261. https://doi.org/10.1016/j.gca.2015.03.037
). Therefore, stable Ca isotopes could be useful for investigating the origin of Ca excess in Ryugu samples compared to CIs.Here we have analysed the stable Ca isotopic compositions of Ryugu samples collected from the first and second touchdown sites, using the collision cell equipped multicollection inductively-coupled plasma mass spectrometer (CC-MC-ICP-MS), Nu Sapphire.
top
Samples and Methods
The samples returned by the Hayabusa2 spacecraft consist of ∼5 g of materials from the Ryugu asteroid recovered during two touchdowns (Tachibana et al., 2022
Tachibana, S., Sawada, H., Okazaki, R., Takano, Y., Sakamoto, K. et al. (2022) Pebbles and sand on asteroid (162173) Ryugu: In situ observation and particles returned to Earth. Science 375, 1011–1016. https://doi.org/10.1126/science.abj8624
; Yada et al., 2022Yada, T., Abe, M., Okada, T., Nakato, A., Yogata, K. et al. (2022) Preliminary analysis of the Hayabusa2 samples returned from C-type asteroid Ryugu. Nature Astronomy 6, 214–220. https://doi.org/10.1038/s41550-021-01550-6
). Approximately 3 g of samples representing the surface materials of Ryugu were collected during the first touchdown and stored in Chamber A. Approximately 2 g of samples likely representing a mixture of materials from the surface and subsurface were collected into Chamber C at a site that was close to the crater formed by the Small Carry-on Impactor, a kinetic impact experiment of the Hayabusa2 mission (Saiki et al., 2017Saiki, S., Imamura, H., Arakawa, M., Wada, K., Takagi, Y., Hayakawa, M., Shirai, K., Yano, H., Okamoto, C. (2017) The Small Carry-on Impactor (SCI) and the Hayabusa2 Impact Experiment. Space Science Reviews 208, 165–186. https://doi.org/10.1007/s11214-016-0297-5
; Arakawa et al., 2020Arakawa, M., Saiki, T., Wada, K., Ogawa, K., Kadono, T. et al. (2020) An artificial impact on the asteroid (162173) Ryugu formed a crater in the gravity-dominated regime. Science 368, 67–71. https://doi.org/10.1126/science.aaz1701
). Two Ryugu samples, A0106-A0107 (Chamber A) and C0108 (Chamber C), were analysed in this study (for information on the mineralogy see https://jaxa.repo.nii.ac.jp/?action=repository_uri&item_id=48255&file_id=31&file_no=1).Sample A0106-A0107 was prepared from a mixed aggregate of A0106 (1.6 mg) and A0107 (27.3 mg). In addition to the Ryugu samples, fusion-crust free bulk samples of six CC, Orgueil (CI1), Alais (CI1), Tarda (C2-ungrouped), Tagish Lake (C2-ungrouped), Murchison (CM2), and Allende (CV3), were analysed in the same way for comparison. See Table S-1 for the weights and providers of the meteorite samples. All samples were dissolved in PFA vials with a mixture of concentrated HF and HNO3 at the Tokyo Institute of Technology (Yokoyama et al., 2022
Yokoyama, T., Nagashima, K., Nakai, I., Young, E.D., Abe, Y. et al. (2022) Samples returned from the asteroid Ryugu are similar to Ivuna-type carbonaceous meteorites. Science https://doi.org/10.1126/science.abn7850
).After dissolution, aliquots of ∼0.15 % of the solutions containing ∼5 μg of Ca were transferred and dedicated for our study. All the sample aliquots were dried and redissolved in 0.4 ml of 4 mol/L HNO3 in preparation for Ca chemical purification and isotopic measurements at the Institut de Physique du Globe, following Dai et al.
Dai, W., Moynier, F., Paquet, M., Moureau, J., Debret, B., Siebert, J., Gerard, Y., Zhao, Y. (2021) Calcium isotope measurements using a collision cell (CC)-MC-ICP-MS. Chemical Geology 590, 120688. https://doi.org/10.1016/j.chemgeo.2021.120688
(2022Dai, W., Moynier, F., Paquet, M., Moureau, J., Debret, B., Siebert, J., Gerard, Y., Zhao, Y. (2021) Calcium isotope measurements using a collision cell (CC)-MC-ICP-MS. Chemical Geology 590, 120688. https://doi.org/10.1016/j.chemgeo.2021.120688
) (see Supplementary Information).We report both the mass dependent deviation and the radiogenic ingrowth on 40Ca from the decay of 40K. For mass dependent deviation, the data are reported as δx/yCa:

with x and y = 40, 42, 43 or 44. Since most of the published Ca isotope data are measured against the SRM 915a standard, the δ44/40Ca values reported here are re-normalised to SRM915a to facilitate comparison.
The radiogenic ingrowth on 40Ca is reported using the epsilon notation,

with (40Ca/44Ca)n representing the 40Ca/44Ca ratio corrected from the mass dependent isotopic fractionation after being normalised to the 42Ca/44Ca ratio using the exponential law and 42Ca/44Ca = 0.31221 (Russell et al., 1978
Russell, W.A., Papanastassiou, D.A., Tombrello, T.A. (1978) Ca isotope fractionation on the Earth and other solar system materials. Geochimica et Cosmochimica Acta 42, 1075–1090. https://doi.org/10.1016/0016-7037(78)90105-9
).The effect of concentration mismatch on the Sapphire is more significant than on traditional MC-ICP-MS (Moynier et al., 2021
Moynier, F., Hu, Y., Wang, K., Zhao, Y., Gérard, Y., Deng, Z., Moureau, J., Li, W., Simon, J.I., Teng, F.-Z. (2021) Potassium isotopic composition of various samples using a dual-path collision-cell-capable multiple-collector inductively coupled plasma mass spectrometer. Chemical Geology 571, 120144. https://doi.org/10.1016/j.chemgeo.2021.120144
), and all the samples were analysed with Ca concentrations within 1 % of the standard.top
Results and Discussion
The Ca isotopic compositions of the two Ryugu samples and the six CC are reported in Table 1, along with literature values for the chondrites where available. The radiogenic ingrowth on 40Ca from 40K decay was corrected using the K and Ca abundances of the samples (from Yokoyama et al., 2022
Yokoyama, T., Nagashima, K., Nakai, I., Young, E.D., Abe, Y. et al. (2022) Samples returned from the asteroid Ryugu are similar to Ivuna-type carbonaceous meteorites. Science https://doi.org/10.1126/science.abn7850
) and δ44/40Ca (age corrected) ratios are also presented in Table 1. The following discussion focuses on these corrected mass dependent isotopic variations. The δ44/40Ca difference between SRM915b and SRM915a is 0.72 ‰ (Heuser and Eisenhauer, 2008Heuser, A., Eisenhauer, A. (2008) The calcium isotope composition (delta Ca-44/40) of NIST SRM 915b and NIST SRM 1486. Geostandards and Geoanalytical Research 32, 311–315. https://doi.org/10.1111/j.1751-908X.2008.00877.x
). Neither the Ryugu samples nor the meteorites analysed here show any 40Ca anomalies (after age corrections), which is consistent with the literature (e.g., Simon and DePaolo, 2010Simon, J.I., DePaolo, D.J. (2010) Stable calcium isotopic composition of meteorites and rocky planets. Earth and Planetary Science Letters 289, 457–466. https://doi.org/10.1016/j.epsl.2009.11.035
; Huang and Jacobsen, 2017Huang, S., Jacobsen, S.B. (2017) Calcium isotopic compositions of chondrites. Geochimica et Cosmochimica Acta 201, 364–376. https://doi.org/10.1016/j.gca.2016.09.039
).Table 1 Calcium isotopic data from this study and literature (Simon and DePaolo, 2010
Simon, J.I., DePaolo, D.J. (2010) Stable calcium isotopic composition of meteorites and rocky planets. Earth and Planetary Science Letters 289, 457–466. https://doi.org/10.1016/j.epsl.2009.11.035
; Valdes et al., 2014Valdes, M., Moreira, M., Foriel, J., Moynier, F. (2014) The nature of Earth’s building blocks as revealed by calcium isotopes. Earth and Planetary Science Letters 394, 135–145. https://doi.org/10.1016/j.epsl.2014.02.052
; Amsellem et al., 2017Amsellem, E., Moynier, F., Pringle, E., Bouvier, A., Chen, H., Day, J.M.D. (2017) Testing the chondrule-rich accretion model for planetary embryos using calcium isotopes. Earth and Planetary Science Letters 469, 75–83. https://doi.org/10.1016/j.epsl.2017.04.022
; Huang and Jacobsen, 2017Huang, S., Jacobsen, S.B. (2017) Calcium isotopic compositions of chondrites. Geochimica et Cosmochimica Acta 201, 364–376. https://doi.org/10.1016/j.gca.2016.09.039
). 2 s.d. = 2 x standard deviation and 2 s.e. = 2 x standard error (2sd/√n). n is number of measurements.Sample names | δ40/44CaSRM915b | 2 s.d. | δ42/44CaSRM915b | 2 s.d. | δ43/44CaSRM915b | 2 s.d. | ϵ40Ca | 2 s.e | n | δ44/40CaSRM915a | δ44/40CaSRM915a (age corrected) |
Ryugu C0108 | 0.22 | 0.03 | 0.12 | 0.03 | 0.04 | 0.07 | −0.17 | 0.25 | 5 | 0.50 | 0.58 |
Ryugu A0106-A0107 | 0.23 | 0.08 | 0.11 | 0.05 | 0.06 | 0.07 | 0.09 | 0.22 | 6 | 0.49 | 0.55 |
Murchison | 0.12 | 0.07 | 0.02 | 0.07 | 0.01 | 0.10 | 0.85 | 0.46 | 5 | 0.60 | 0.67 |
Murchison (Valdes+) | 0.84 | ||||||||||
Murchison (Huang+.) | 0.72 | ||||||||||
Allende | 0.36 | 0.05 | 0.10 | 0.08 | 0.06 | 0.13 | 1.47 | 0.79 | 4 | 0.36 | 0.39 |
Allende (Simon+) | 0.52 | 0.58 | 2 | 0.49 | 0.54 | ||||||
Allende (Valdes+) | 0.55 | ||||||||||
Allende (Amsellem+) | 0.26 | ||||||||||
Allende (Amsellem+) | 0.10 | ||||||||||
Allende (Amsellem+) | 0.44 | ||||||||||
Allende (Huang+) | 0.28 | ||||||||||
Alais | 0.41 | 0.06 | 0.17 | 0.06 | 0.10 | 0.08 | 0.56 | 0.34 | 6 | 0.31 | 0.42 |
Tarda | 0.34 | 0.06 | 0.15 | 0.07 | 0.05 | 0.07 | 0.25 | 0.37 | 6 | 0.38 | 0.45 |
Tagish Lake | 0.30 | 0.03 | 0.13 | 0.03 | 0.06 | 0.07 | 0.33 | 0.26 | 6 | 0.42 | 0.48 |
Orgueil | 0.30 | 0.04 | 0.13 | 0.02 | 0.06 | 0.08 | 0.44 | 0.40 | 4 | 0.42 | 0.48 |
Orgueil (Amsellem+) | 0.45 | ||||||||||
Orgueil (Valdes+) | 0.65 | ||||||||||
Orgueil (Huang+) | 0.75 |
In a plot of δ44/42Ca vs. δ44/40Ca (age corrected), the data fall along a mass dependent line, regardless of whether the slope for equilibrium fractionation (1/2.1 as shown in Fig. 1) or kinetic fractionation is used. Likewise, variations between δ44/42Ca and δ44/43Ca are mass dependent within error (Fig. S-2). Therefore, the Ca isotopic variations observed among the samples analysed primarily reflect mass dependent isotopic fractionation.

Figure 1 The δ44/42Ca values plotted against δ44/40Ca values for the various samples analysed in this study, including the Ryugu samples. All the samples fall on a mass dependent line within error. Error bars represent 2 sigma standard deviation.
The meteorite data reported here are consistent with literature values (Fig. 2 and Table 1), but it should be noted that literature Ca isotopic values are variable, especially for Orgueil and Allende. The variability may reflect interlaboratory bias, but more likely it reflects isotopic heterogeneity at the sample scale analysed. This is particularly the case for Allende, which contains abundant calcium-aluminum-rich inclusions (CAIs). Since our Allende sample was obtained from the Smithsonian Museum’s large batch of homogenised powder, and our measured Ca isotopic composition falls in the middle of the range previously reported, it is likely representative of the bulk (Fig. 2). For Orgueil, part of the interlaboratory variability may be controlled by the variable distribution of secondary phases produced by aqueous alteration since Ca may be isotopically fractionated during alteration and carbonate precipitation (Blättler and Higgins, 2017
Blättler, C., Higgins, J. (2017) Testing Urey’s carbonate–silicate cycle using the calcium isotopic composition of sedimentary carbonates. Earth and Planetary Science Letters 479, 241–251. https://doi.org/10.1016/j.epsl.2017.09.033
). However, none of the studies that report Ca isotopic data include the Ca contents of their Orgueil analyses. We report here the first δ44/40Ca values for Tarda and Tagish Lake, which are within error of one another and overlap with Orgueil.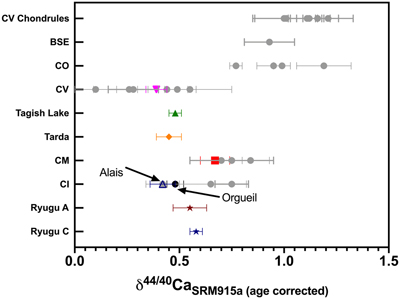
Figure 2 A comparison of age corrected δ44/40Ca values for the samples analysed here (in colour, see Fig. 1) and from the literature (grey). Ryugu samples from Chambers A and C are similar within error and fall within the range defined by the CI chondrites. The literature data are from Table 1, BSE estimate from Kang et al. (2017)
Kang, J.T., Ionov, D.A., Liu, F., Zhang, C.L., Golovin, A.V., Qin, L.-P., Zhang, Z.-F., Huang, F. (2017) Calcium isotopic fractionation in mantle peridotites by melting and metasomatism and Ca isotope composition of the Bulk Silicate Earth. Earth and Planetary Science Letters 474, 128–137. https://doi.org/10.1016/j.epsl.2017.05.035
and chondrules data from Amsellem et al. (2017)Amsellem, E., Moynier, F., Pringle, E., Bouvier, A., Chen, H., Day, J.M.D. (2017) Testing the chondrule-rich accretion model for planetary embryos using calcium isotopes. Earth and Planetary Science Letters 469, 75–83. https://doi.org/10.1016/j.epsl.2017.04.022
. Error bars are 2 x standard deviation.The two Ryugu samples have Ca isotopic compositions within error of one another (δ44/40Ca = 0.58 ± 0.03 ‰ for C0108 and 0.55 ± 0.08 ‰ for A0106-A0107; uncertainties represent 2 s.e. for n = 5 and 6, respectively). They are also within the range of published δ44/40Ca values for CIs (Fig. 2). Notably, Ryugu sample A0106-A0107 (Ca/Al ∼ 1.9) has almost twice the amount of Ca compared to the average CI (Ca/Al ∼ 1.06) (Fig. 3), and ∼20 % more than Ryugu sample C0108 (Ca/Al ∼ 1.55). If the excess Ca in A0106-A0107 is mainly stored in secondary carbonates (Yokoyama et al., 2022
Yokoyama, T., Nagashima, K., Nakai, I., Young, E.D., Abe, Y. et al. (2022) Samples returned from the asteroid Ryugu are similar to Ivuna-type carbonaceous meteorites. Science https://doi.org/10.1126/science.abn7850
and our discussion below), then these carbonates must contribute significantly to the bulk Ca isotopic composition. Therefore, our results imply that the Ca isotopic composition of the Ryugu samples and CIs are not significantly modified by the dissolution of primary Ca-bearing phases and precipitation as carbonates during aqueous alteration. One reason for the similar Ca isotopic compositions in Ryugu samples and CIs is that their Ca isotopic compositions are mostly dominated by the high abundance and the composition of the carbonates.
Figure 3 δ44/40Ca plotted against the Ca/Al ratio of the samples. The Ca/Al ratios of bulk Chamber A and Chamber C samples from Yokoyama et al.
Yokoyama, T., Nagashima, K., Nakai, I., Young, E.D., Abe, Y. et al. (2022) Samples returned from the asteroid Ryugu are similar to Ivuna-type carbonaceous meteorites. Science https://doi.org/10.1126/science.abn7850
(2022Yokoyama, T., Nagashima, K., Nakai, I., Young, E.D., Abe, Y. et al. (2022) Samples returned from the asteroid Ryugu are similar to Ivuna-type carbonaceous meteorites. Science https://doi.org/10.1126/science.abn7850
) are used for Ryugu samples in this work and are taken from the average value in Barrat et al.Barrat, J.A., Zanda, B., Moynier, F., Bollinger, C., Liorzou, C. et al. (2012) Geochemistry of CI chondrites: Major and trace elements, and Cu and Zn Isotopes. Geochimica et Cosmochimica Acta 83, 79–92. https://doi.org/10.1016/j.gca.2011.12.011
(2012Barrat, J.A., Zanda, B., Moynier, F., Bollinger, C., Liorzou, C. et al. (2012) Geochemistry of CI chondrites: Major and trace elements, and Cu and Zn Isotopes. Geochimica et Cosmochimica Acta 83, 79–92. https://doi.org/10.1016/j.gca.2011.12.011
) for Orgueil, as it was not available for specific samples used here.Several studies have quantified the modal abundances of minerals in CIs, but there is no systematic study and consensus on the Ca carriers. Calcium sulfates are usually not detected in CIs but can be present at up to ∼1 vol. % (e.g., Endress and Bischoff, 1996
Endress, M., Bishoff, A. (1996) Carbonates in CI chondrites: Clues to parent body evolution. Geochimica et Cosmochimica Acta 60, 489–507. https://doi.org/10.1016/0016-7037(95)00399-1
; Morlok, et al., 2006Morlok, A., Bischoff, A., Stephan, T., Floss, C., Zinner, E., Jessberger, E.K. (2006) Brecciation and chemical heterogeneities of CI chondrites. Geochimica et Cosmochimica Acta 70, 5371–5394. https://doi.org/10.1016/j.gca.2006.08.007
). Even when present, they were suggested to have formed during terrestrial alteration (Gounelle and Zolensky, 2001Gounelle, M., Zolensky, M. (2001) A terrestrial origin for sulfate veins in CI1 chondrites. Meteoritics & Planetary Science. 36, 1321–1329. https://doi.org/10.1111/j.1945-5100.2001.tb01827.x
). Ca-rich phosphates exist in CI chondrites (Morlok et al., 2006Morlok, A., Bischoff, A., Stephan, T., Floss, C., Zinner, E., Jessberger, E.K. (2006) Brecciation and chemical heterogeneities of CI chondrites. Geochimica et Cosmochimica Acta 70, 5371–5394. https://doi.org/10.1016/j.gca.2006.08.007
), but they appear to be quite rare (0–0.05 vol. %; King et al., 2015King, A., Schofield, P.F., Howard, K., Russell, S.S. (2015) Modal mineralogy of CI and CI-like chondrites by X-ray diffraction. Geochimica et Cosmochimica Acta 165, 148–160. https://doi.org/10.1016/j.gca.2015.05.038
; Alfing et al., 2019Alfing, J., Patsek, M., Bischoff, A. (2019) Geochemistry Modal abundances of coarse-grained (>5μm) components within CI-chondrites and their individual clasts - Mixing of various lithologies on the CI parent body(ies). Geochemistry 39, 3–16. https://doi.org/10.1016/j.chemer.2019.08.004
) and therefore are unlikely to have a strong control on the total Ca budget. Thus, carbonates are the most likely major carriers of Ca in CIs (Endress and Bischoff, 1996Endress, M., Bishoff, A. (1996) Carbonates in CI chondrites: Clues to parent body evolution. Geochimica et Cosmochimica Acta 60, 489–507. https://doi.org/10.1016/0016-7037(95)00399-1
; Morlok et al., 2006Morlok, A., Bischoff, A., Stephan, T., Floss, C., Zinner, E., Jessberger, E.K. (2006) Brecciation and chemical heterogeneities of CI chondrites. Geochimica et Cosmochimica Acta 70, 5371–5394. https://doi.org/10.1016/j.gca.2006.08.007
, Alfing et al., 2019Alfing, J., Patsek, M., Bischoff, A. (2019) Geochemistry Modal abundances of coarse-grained (>5μm) components within CI-chondrites and their individual clasts - Mixing of various lithologies on the CI parent body(ies). Geochemistry 39, 3–16. https://doi.org/10.1016/j.chemer.2019.08.004
). Scanning electron microscopic (SEM) analyses of 18 sections of CIs (including Orgueil) point to an average carbonate abundance of ∼5 vol. % and the carbonates are dominated by dolomite (Endress and Bischoff, 1996Endress, M., Bishoff, A. (1996) Carbonates in CI chondrites: Clues to parent body evolution. Geochimica et Cosmochimica Acta 60, 489–507. https://doi.org/10.1016/0016-7037(95)00399-1
). However, analyses of CO2 released by phosphoric acid dissolution of ∼100 mg of Orgueil only returned ∼0.1 wt. % of carbonate C (Alexander et al., 2015Alexander, C.M.O’D., Bowden, R., Fogel, M.L., Howard, K. (2015) Carbonate abundances and isotopic compositions in chondrites. Meteoritics & Planetary Science 50, 810–833. https://doi.org/10.1111/maps.12410
), which is equivalent to ∼0.8 wt. % carbonate (although carbonate abundance in Ivuna estimated by a similar method is three times higher). X-ray diffraction (detection limit ∼1 vol. %) did not reveal carbonates in three Orgueil samples (from 50 to 200 mg), but 2 vol. % in Alais (200 mg) and 3 vol. % in Ivuna (50 mg) (King et al., 2015King, A., Schofield, P.F., Howard, K., Russell, S.S. (2015) Modal mineralogy of CI and CI-like chondrites by X-ray diffraction. Geochimica et Cosmochimica Acta 165, 148–160. https://doi.org/10.1016/j.gca.2015.05.038
), while Bland et al.Bland, P., Cressey, G., Menzies, O. (2010) Modal mineralogy of carbonaceous chondrites by X-ray diffraction and Mossbauer spectroscopy. Meteoritics and Planetary Science 39, 3–16. https://doi.org/10.1111/j.1945-5100.2004.tb00046.x
(2004Bland, P., Cressey, G., Menzies, O. (2010) Modal mineralogy of carbonaceous chondrites by X-ray diffraction and Mossbauer spectroscopy. Meteoritics and Planetary Science 39, 3–16. https://doi.org/10.1111/j.1945-5100.2004.tb00046.x
) detected no carbonates in Orgueil (200–300 mg samples). Given the variability in the modal mineralogy in the literature, Alfing et al.Alfing, J., Patsek, M., Bischoff, A. (2019) Geochemistry Modal abundances of coarse-grained (>5μm) components within CI-chondrites and their individual clasts - Mixing of various lithologies on the CI parent body(ies). Geochemistry 39, 3–16. https://doi.org/10.1016/j.chemer.2019.08.004
(2009Alfing, J., Patsek, M., Bischoff, A. (2019) Geochemistry Modal abundances of coarse-grained (>5μm) components within CI-chondrites and their individual clasts - Mixing of various lithologies on the CI parent body(ies). Geochemistry 39, 3–16. https://doi.org/10.1016/j.chemer.2019.08.004
) focused on phases >5 μm (which only represent ∼6 vol. % of CIs) and found ∼0.5 wt. % of carbonates in CIs. A variability in the abundance of carbonates in CIs is consistent with variable Ca concentrations (from 0.77 to 0.96 wt. %) measured even in large (0.5–1 g) Orgueil bulk samples (Barrat et al., 2012Barrat, J.A., Zanda, B., Moynier, F., Bollinger, C., Liorzou, C. et al. (2012) Geochemistry of CI chondrites: Major and trace elements, and Cu and Zn Isotopes. Geochimica et Cosmochimica Acta 83, 79–92. https://doi.org/10.1016/j.gca.2011.12.011
). Considering that the most abundant carbonates in Orgueil are dolomites with ∼20 wt. % Ca (Endress and Bishoff, 1996Endress, M., Bishoff, A. (1996) Carbonates in CI chondrites: Clues to parent body evolution. Geochimica et Cosmochimica Acta 60, 489–507. https://doi.org/10.1016/0016-7037(95)00399-1
), the presence of ∼4 wt. % carbonates in CIs would be sufficient to dominate their Ca budget, less if calcites or aragonites are involved. Despite the variability in carbonate abundances of CIs reported in the literature, we suggest that the major fraction of Ca in CIs is stored in carbonates.Nakamura, T. et al.
Nakamura, T., Matsumoto, M., Amano, K., Enokido, Y., Zolensky, M. et al. (2022) Formation and evolution of carbonaceous asteroid Ryugu: Direct evidence from returned samples. Science. https://doi.org/10.1126/science.abn8671
(2022Nakamura, T., Matsumoto, M., Amano, K., Enokido, Y., Zolensky, M. et al. (2022) Formation and evolution of carbonaceous asteroid Ryugu: Direct evidence from returned samples. Science. https://doi.org/10.1126/science.abn8671
) estimated the mineral abundances and compositions of the main phases of the Ryugu samples by SEM observations of two ∼10 mm2 sections from a sample from the second touchdown site (sample C0002) (see their Tables S6 and S7). No Ca sulfates were found in these sections, and a simple mass balance using their data shows that carbonates account for 75–80 % of the Ca budget, with apatite and phyllosilicates accommodating the remaining Ca more or less equally. This calculation may underestimate the Ca fraction in carbonates because the samples also contain small Ca carbonate grains (e.g., Table S7 of Nakamura T. et al., 2022Nakamura, T., Matsumoto, M., Amano, K., Enokido, Y., Zolensky, M. et al. (2022) Formation and evolution of carbonaceous asteroid Ryugu: Direct evidence from returned samples. Science. https://doi.org/10.1126/science.abn8671
), the abundances of which could not be quantified here. C0002 is the third largest sample among all returned grains containing the major lithology (Nakamura T. et al., 2022Nakamura, T., Matsumoto, M., Amano, K., Enokido, Y., Zolensky, M. et al. (2022) Formation and evolution of carbonaceous asteroid Ryugu: Direct evidence from returned samples. Science. https://doi.org/10.1126/science.abn8671
), suggesting that the Ca budget in the Ryugu samples is dominated by carbonates. It should be noted that another study also found several vol. % of carbonate minerals in samples from both touchdown sites, with a large variability in Ca content between ∼1 mg grains (Nakamura E. et al., 2022Nakamura, E., Kobayashi, K., Tanaka, R., Kunihiro, T., Kitagawa, H. et al. (2022) On the origin and evolution of the asteroid Ryugu: A comprehensive geochemical perspective. Proceedings of the Japan Academy, Series B. 6, 227–282. https://doi.org/10.2183/pjab.98.015
) and a correlation between Ca content and the dolomite abundances of the grains (Fig. S-1). The two heaviest Ca isotopic compositions for Orgueil samples from Valdes et al.Valdes, M., Moreira, M., Foriel, J., Moynier, F. (2014) The nature of Earth’s building blocks as revealed by calcium isotopes. Earth and Planetary Science Letters 394, 135–145. https://doi.org/10.1016/j.epsl.2014.02.052
(2014Valdes, M., Moreira, M., Foriel, J., Moynier, F. (2014) The nature of Earth’s building blocks as revealed by calcium isotopes. Earth and Planetary Science Letters 394, 135–145. https://doi.org/10.1016/j.epsl.2014.02.052
) (0.65 ± 0.17 ‰, 2 s.d.) and Huang and JacobsenHuang, S., Jacobsen, S.B. (2017) Calcium isotopic compositions of chondrites. Geochimica et Cosmochimica Acta 201, 364–376. https://doi.org/10.1016/j.gca.2016.09.039
(2017Huang, S., Jacobsen, S.B. (2017) Calcium isotopic compositions of chondrites. Geochimica et Cosmochimica Acta 201, 364–376. https://doi.org/10.1016/j.gca.2016.09.039
) (0.75 ± 0.11 ‰, 2 s.d.) may reflect different proportions of carbonates. Unfortunately, these two studies did not report the Ca contents of their Orgueil fractions, so it is not possible to test this hypothesis.The similar Ca isotopic compositions between the two Ryugu samples and CIs are most simply explained if the Ca excesses observed in the bulk Ryugu samples are due to the heterogeneous distribution of carbonates, and if these carbonates have similar Ca isotopic compositions to the bulk samples. This explanation is consistent with an episode of fluid circulation and carbonate precipitation in the Ryugu samples that occurred 2.5 to 5 Myr after CAIs formation, as dated using 53Mn-53Cr chronometry in carbonate phases (Nakamura E. et al., 2022
Nakamura, E., Kobayashi, K., Tanaka, R., Kunihiro, T., Kitagawa, H. et al. (2022) On the origin and evolution of the asteroid Ryugu: A comprehensive geochemical perspective. Proceedings of the Japan Academy, Series B. 6, 227–282. https://doi.org/10.2183/pjab.98.015
; Yokoyama et al., 2022Yokoyama, T., Nagashima, K., Nakai, I., Young, E.D., Abe, Y. et al. (2022) Samples returned from the asteroid Ryugu are similar to Ivuna-type carbonaceous meteorites. Science https://doi.org/10.1126/science.abn7850
). Hence, at present the average of the two Ryugu samples (0.57 ± 0.04 ‰, 2 s.d.) represents the best estimate of Ryugu’s and Solar System Ca isotopic composition. Future work should test whether this value is representative of the whole body by analysing other Ryugu fragments containing fewer carbonate phases and less total Ca, such as the Ryugu material in section 5 from C0002 (Nakamura T. et al., 2022Nakamura, T., Matsumoto, M., Amano, K., Enokido, Y., Zolensky, M. et al. (2022) Formation and evolution of carbonaceous asteroid Ryugu: Direct evidence from returned samples. Science. https://doi.org/10.1126/science.abn8671
), which only contains ∼75 % of its Ca in carbonates.top
Acknowledgements
This work was partly supported by the IPGP analytical platform PARI, Ile-de-France SESAME Grants 12015908, DIM ACAV+, the ERC grant 101001282 (METAL), Labex UnivEarth (FM), JSPS Kaken-hi grants (ST, HY, TY) and the CNES. We thank Dominik Hezel and one anonymous reviewer for constructive comments that helped improve the manuscript.
Editor: Anat Shahar
top
References
Alfing, J., Patsek, M., Bischoff, A. (2019) Geochemistry Modal abundances of coarse-grained (>5μm) components within CI-chondrites and their individual clasts - Mixing of various lithologies on the CI parent body(ies). Geochemistry 39, 3–16. https://doi.org/10.1016/j.chemer.2019.08.004

Ca-rich phosphates exist in CI chondrites (Morlok et al., 2006), but they appear to be quite rare (0–0.05 vol. %; King et al., 2015; Alfing et al., 2019) and therefore are unlikely to have a strong control on the total Ca budget.
View in article
Thus, carbonates are the most likely major carriers of Ca in CIs (Endress and Bischoff, 1996; Morlok et al., 2006, Alfing et al., 2019).
View in article
Alexander, C.M.O’D., Bowden, R., Fogel, M.L., Howard, K. (2015) Carbonate abundances and isotopic compositions in chondrites. Meteoritics & Planetary Science 50, 810–833. https://doi.org/10.1111/maps.12410

However, analyses of CO2 released by phosphoric acid dissolution of ∼100 mg of Orgueil only returned ∼0.1 wt. % of carbonate C (Alexander et al., 2015), which is equivalent to ∼0.8 wt. % carbonate (although carbonate abundance in Ivuna estimated by a similar method is three times higher).
View in article
Amsellem, E., Moynier, F., Pringle, E., Bouvier, A., Chen, H., Day, J.M.D. (2017) Testing the chondrule-rich accretion model for planetary embryos using calcium isotopes. Earth and Planetary Science Letters 469, 75–83. https://doi.org/10.1016/j.epsl.2017.04.022

Calcium isotopic data from this study and literature (Simon and DePaolo, 2010; Valdes et al., 2014; Amsellem et al., 2017; Huang and Jacobsen, 2017).
View in article
The literature data are from Table 1, BSE estimate from Kang et al. (2017) and chondrules data from Amsellem et al. (2017). Error bars are 2 x standard deviation.
View in article
Arakawa, M., Saiki, T., Wada, K., Ogawa, K., Kadono, T. et al. (2020) An artificial impact on the asteroid (162173) Ryugu formed a crater in the gravity-dominated regime. Science 368, 67–71. https://doi.org/10.1126/science.aaz1701

Approximately 2 g of samples likely representing a mixture of materials from the surface and subsurface were collected into Chamber C at a site that was close to the crater formed by the Small Carry-on Impactor, a kinetic impact experiment of the Hayabusa2 mission (Saiki et al., 2017; Arakawa et al., 2020).
View in article
Barrat, J.A., Zanda, B., Moynier, F., Bollinger, C., Liorzou, C. et al. (2012) Geochemistry of CI chondrites: Major and trace elements, and Cu and Zn Isotopes. Geochimica et Cosmochimica Acta 83, 79–92. https://doi.org/10.1016/j.gca.2011.12.011

The Ca/Al ratios of bulk Chamber A and Chamber C samples from Yokoyama et al. (2022) are used for Ryugu samples in this work and are taken from the average value in Barrat et al. (2012) for Orgueil, as it was not available for specific samples used here.
View in article
A variability in the abundance of carbonates in CIs is consistent with variable Ca concentrations (from 0.77 to 0.96 wt. %) measured even in large (0.5–1 g) Orgueil bulk samples (Barrat et al., 2012).
View in article
Bland, P., Cressey, G., Menzies, O. (2010) Modal mineralogy of carbonaceous chondrites by X-ray diffraction and Mossbauer spectroscopy. Meteoritics and Planetary Science 39, 3–16. https://doi.org/10.1111/j.1945-5100.2004.tb00046.x

X-ray diffraction (detection limit ∼1 vol. %) did not reveal carbonates in three Orgueil samples (from 50 to 200 mg), but 2 vol. % in Alais (200 mg) and 3 vol. % in Ivuna (50 mg) (King et al., 2015), while Bland et al. (2004) detected no carbonates in Orgueil (200–300 mg samples).
View in article
Blättler, C., Higgins, J. (2017) Testing Urey’s carbonate–silicate cycle using the calcium isotopic composition of sedimentary carbonates. Earth and Planetary Science Letters 479, 241–251. https://doi.org/10.1016/j.epsl.2017.09.033

Calcium is a major constituent of carbonates and can be isotopically fractionated during aqueous alteration and carbonate precipitation, leading to more than 1 ‰ variations in the 44Ca/40Ca ratio in terrestrial carbonates (e.g., Fantle and Tipper, 2014; Blättler and Higgins, 2017).
View in article
For Orgueil, part of the interlaboratory variability may be controlled by the variable distribution of secondary phases produced by aqueous alteration since Ca may be isotopically fractionated during alteration and carbonate precipitation (Blättler and Higgins, 2017).
View in article
Dai, W., Moynier, F., Paquet, M., Moureau, J., Debret, B., Siebert, J., Gerard, Y., Zhao, Y. (2021) Calcium isotope measurements using a collision cell (CC)-MC-ICP-MS. Chemical Geology 590, 120688. https://doi.org/10.1016/j.chemgeo.2021.120688

All the sample aliquots were dried and redissolved in 0.4 ml of 4 mol/L HNO3 in preparation for Ca chemical purification and isotopic measurements at the Institut de Physique du Globe, following Dai et al. (2022) (see Supplementary Information).
View in article
Dauphas, N., Pourmand, A. (2015) Thulium anomalies and rare earth element patterns in meteorites and Earth: Nebular fractionation and the nugget effect. Geochimica et Cosmochimica Acta 163, 234–261. https://doi.org/10.1016/j.gca.2015.03.037

This range is likely related to a combination of the variable modal abundances of refractory inclusions among CC (Hezel et al., 2008) that can be enriched in the lighter Ca isotopes by several per mille (Niederer and Papanastassiou, 1984; Huang et al., 2012) and the heterogeneous distribution of carbonates (Simon and DePaolo, 2010; Valdes et al., 2014; Dauphas and Pourmand, 2015).
View in article
Endress, M., Bishoff, A. (1996) Carbonates in CI chondrites: Clues to parent body evolution. Geochimica et Cosmochimica Acta 60, 489–507. https://doi.org/10.1016/0016-7037(95)00399-1

Calcium sulfates are usually not detected in CIs but can be present at up to ∼1 vol. % (e.g., Endress and Bischoff, 1996; Morlok, et al., 2006).
View in article
Thus, carbonates are the most likely major carriers of Ca in CIs (Endress and Bischoff, 1996; Morlok et al., 2006, Alfing et al., 2019).
View in article
Scanning electron microscopic (SEM) analyses of 18 sections of CIs (including Orgueil) point to an average carbonate abundance of ∼5 vol. % and the carbonates are dominated by dolomite (Endress and Bischoff, 1996).
View in article
Considering that the most abundant carbonates in Orgueil are dolomites with ∼20 wt. % Ca (Endress and Bishoff, 1996), the presence of ∼4 wt. % carbonates in CIs would be sufficient to dominate their Ca budget, less if calcites or aragonites are involved.
View in article
Fantle, M.S., Tipper, E.T. (2014) Calcium isotopes in the global biogeochemical Ca cycle: Implications for development of a Ca isotope proxy. Earth-Science Reviews 129, 148–177. https://doi.org/10.1016/j.earscirev.2013.10.004

Calcium is a major constituent of carbonates and can be isotopically fractionated during aqueous alteration and carbonate precipitation, leading to more than 1 ‰ variations in the 44Ca/40Ca ratio in terrestrial carbonates (e.g., Fantle and Tipper, 2014; Blättler and Higgins, 2017).
View in article
Gounelle, M., Zolensky, M. (2001) A terrestrial origin for sulfate veins in CI1 chondrites. Meteoritics & Planetary Science. 36, 1321–1329. https://doi.org/10.1111/j.1945-5100.2001.tb01827.x

Even when present, they were suggested to have formed during terrestrial alteration (Gounelle and Zolensky, 2001).
View in article
Heuser, A., Eisenhauer, A. (2008) The calcium isotope composition (delta Ca-44/40) of NIST SRM 915b and NIST SRM 1486. Geostandards and Geoanalytical Research 32, 311–315. https://doi.org/10.1111/j.1751-908X.2008.00877.x

The following discussion focuses on these corrected mass dependent isotopic variations. The δ44/40Ca difference between SRM915b and SRM915a is 0.72 ‰ (Heuser and Eisenhauer, 2008).
View in article
Hezel, DC., Russell, S.S., Ross, A.J., Kearsley, A.T. (2008) Modal Abundances of CAIs: Implications for bulk chondrite element abundances and fractionations. Meteoritics & Planetary Science 43, 1879–1894. https://doi.org/10.1111/j.1945-5100.2008.tb00649.x

This range is likely related to a combination of the variable modal abundances of refractory inclusions among CC (Hezel et al., 2008) that can be enriched in the lighter Ca isotopes by several per mille (Niederer and Papanastassiou, 1984; Huang et al., 2012) and the heterogeneous distribution of carbonates (Simon and DePaolo, 2010; Valdes et al., 2014; Dauphas and Pourmand, 2015).
View in article
Huang, S., Jacobsen, S.B. (2017) Calcium isotopic compositions of chondrites. Geochimica et Cosmochimica Acta 201, 364–376. https://doi.org/10.1016/j.gca.2016.09.039

Neither the Ryugu samples nor the meteorites analysed here show any 40Ca anomalies (after age corrections), which is consistent with the literature (e.g., Simon and DePaolo, 2010; Huang and Jacobsen, 2017).
View in article
Calcium isotopic data from this study and literature (Simon and DePaolo, 2010; Valdes et al., 2014; Amsellem et al., 2017; Huang and Jacobsen, 2017).
View in article
The two heaviest Ca isotopic compositions for Orgueil samples from Valdes et al. (2014) (0.65 ± 0.17 ‰, 2 s.d.) and Huang and Jacobsen (2017) (0.75 ± 0.11 ‰, 2 s.d.) may reflect different proportions of carbonates.
View in article
Huang, S., Farkas, J., Yu, G., Petaev, M.I., Jacobsen, S.B. (2012) Calcium isotopic ratios and rare earth elements abundances from refractory inclusions from the Allende CV3 chondrite. Geochimica et Cosmochimica Acta 77, 252–265. https://doi.org/10.1016/j.gca.2011.11.002

This range is likely related to a combination of the variable modal abundances of refractory inclusions among CC (Hezel et al., 2008) that can be enriched in the lighter Ca isotopes by several per mille (Niederer and Papanastassiou, 1984; Huang et al., 2012) and the heterogeneous distribution of carbonates (Simon and DePaolo, 2010; Valdes et al., 2014; Dauphas and Pourmand, 2015).
View in article
Kang, J.T., Ionov, D.A., Liu, F., Zhang, C.L., Golovin, A.V., Qin, L.-P., Zhang, Z.-F., Huang, F. (2017) Calcium isotopic fractionation in mantle peridotites by melting and metasomatism and Ca isotope composition of the Bulk Silicate Earth. Earth and Planetary Science Letters 474, 128–137. https://doi.org/10.1016/j.epsl.2017.05.035

The literature data are from Table 1, BSE estimate from Kang et al. (2017) and chondrules data from Amsellem et al. (2017). Error bars are 2 x standard deviation.
View in article
King, A., Schofield, P.F., Howard, K., Russell, S.S. (2015) Modal mineralogy of CI and CI-like chondrites by X-ray diffraction. Geochimica et Cosmochimica Acta 165, 148–160. https://doi.org/10.1016/j.gca.2015.05.038

Ca-rich phosphates exist in CI chondrites (Morlok et al., 2006), but they appear to be quite rare (0–0.05 vol. %; King et al., 2015; Alfing et al., 2019) and therefore are unlikely to have a strong control on the total Ca budget.
View in article
X-ray diffraction (detection limit ∼1 vol. %) did not reveal carbonates in three Orgueil samples (from 50 to 200 mg), but 2 vol. % in Alais (200 mg) and 3 vol. % in Ivuna (50 mg) (King et al., 2015), while Bland et al. (2004) detected no carbonates in Orgueil (200–300 mg samples).
View in article
Nakamura, T. et al. (2022) estimated the mineral abundances and compositions of the main phases of the Ryugu samples by SEM observations of two ∼10 mm2 sections from a sample from the second touchdown site (sample C0002) (see their Tables S6 and S7).
View in article
Morlok, A., Bischoff, A., Stephan, T., Floss, C., Zinner, E., Jessberger, E.K. (2006) Brecciation and chemical heterogeneities of CI chondrites. Geochimica et Cosmochimica Acta 70, 5371–5394. https://doi.org/10.1016/j.gca.2006.08.007

Calcium sulfates are usually not detected in CIs but can be present at up to ∼1 vol. % (e.g., Endress and Bischoff, 1996; Morlok, et al., 2006).
View in article
Ca-rich phosphates exist in CI chondrites (Morlok et al., 2006), but they appear to be quite rare (0–0.05 vol. %; King et al., 2015; Alfing et al., 2019) and therefore are unlikely to have a strong control on the total Ca budget.
View in article
Thus, carbonates are the most likely major carriers of Ca in CIs (Endress and Bischoff, 1996; Morlok et al., 2006, Alfing et al., 2019).
View in article
Moynier, F., Hu, Y., Wang, K., Zhao, Y., Gérard, Y., Deng, Z., Moureau, J., Li, W., Simon, J.I., Teng, F.-Z. (2021) Potassium isotopic composition of various samples using a dual-path collision-cell-capable multiple-collector inductively coupled plasma mass spectrometer. Chemical Geology 571, 120144. https://doi.org/10.1016/j.chemgeo.2021.120144

The effect of concentration mismatch on the Sapphire is more significant than on traditional MC-ICP-MS (Moynier et al., 2021), and all the samples were analysed with Ca concentrations within 1 % of the standard.
View in article
Nakamura, E., Kobayashi, K., Tanaka, R., Kunihiro, T., Kitagawa, H. et al. (2022) On the origin and evolution of the asteroid Ryugu: A comprehensive geochemical perspective. Proceedings of the Japan Academy, Series B. 6, 227–282. https://doi.org/10.2183/pjab.98.015

In particular, their bulk Cr and Ti isotopic signatures and the chemical abundances of most elements are within the range of CI chondrites (Nakamura E. et al., 2022; Yokoyama et al., 2022).
View in article
It should be noted that another study also found several vol. % of carbonate minerals in samples from both touchdown sites, with a large variability in Ca content between ∼1 mg grains (Nakamura E. et al., 2022) and a correlation between Ca content and the dolomite abundances of the grains (Fig. S-1).
View in article
This explanation is consistent with an episode of fluid circulation and carbonate precipitation in the Ryugu samples that occurred 2.5 to 5 Myr after CAIs formation, as dated using 53Mn-53Cr chronometry in carbonate phases (Nakamura E. et al., 2022; Yokoyama et al., 2022).
View in article
Nakamura, T., Matsumoto, M., Amano, K., Enokido, Y., Zolensky, M. et al. (2022) Formation and evolution of carbonaceous asteroid Ryugu: Direct evidence from returned samples. Science. https://doi.org/10.1126/science.abn8671

Despite many aspects of similarities with the CIs, the Ryugu samples display an apparent excess of Ca by over 50 %, which may be related to a heterogeneous distribution of carbonates (dolomite and calcite) (Nakamura T. et al., 2022; Yokoyama et al., 2022) between Ryugu, Orgueil and other CIs.
View in article
This calculation may underestimate the Ca fraction in carbonates because the samples also contain small Ca carbonate grains (e.g., Table S7 of Nakamura T. et al., 2022), the abundances of which could not be quantified here.
View in article
C0002 is the third largest sample among all returned grains containing the major lithology (Nakamura T. et al., 2022), suggesting that the Ca budget in the Ryugu samples is dominated by carbonates.
View in article
Future work should test whether this value is representative of the whole body by analysing other Ryugu fragments containing fewer carbonate phases and less total Ca, such as the Ryugu material in section 5 from C0002 (Nakamura T. et al., 2022), which only contains ∼75 % of its Ca in carbonates.
View in article
Niederer, F.R., Papanastassiou, D.A. (1984) Ca isotopes in refractory inclusions. Geochimica et Cosmochimica Acta 48, 1279–1293. https://doi.org/10.1016/0016-7037(84)90062-0

This range is likely related to a combination of the variable modal abundances of refractory inclusions among CC (Hezel et al., 2008) that can be enriched in the lighter Ca isotopes by several per mille (Niederer and Papanastassiou, 1984; Huang et al., 2012) and the heterogeneous distribution of carbonates (Simon and DePaolo, 2010; Valdes et al., 2014; Dauphas and Pourmand, 2015).
View in article
Palme, H., Lodders, K., Jones, A. (2014) 2.2 - Solar System abundances of the elements. In: Holland, H.D., Turekian, K.K. (Exec. Eds.) Treatise on Geochemistry (Second Edition). Elsevier, Oxford, 15–36. https://doi.org/10.1016/B978-0-08-095975-7.00118-2

Among meteorites, CI chondrites have chemical compositions that most closely resemble the Sun; therefore, they are the most representative samples of the solar nebula composition with the exception of volatile elements (Palme et al., 2014).
View in article
Russell, W.A., Papanastassiou, D.A., Tombrello, T.A. (1978) Ca isotope fractionation on the Earth and other solar system materials. Geochimica et Cosmochimica Acta 42, 1075–1090. https://doi.org/10.1016/0016-7037(78)90105-9

The radiogenic ingrowth on 40Ca is reported using the epsilon notation, with (40Ca/44Ca)n representing the 40Ca/44Ca ratio corrected from the mass dependent isotopic fractionation after being normalised to the 42Ca/44Ca ratio using the exponential law and 42Ca/44Ca = 0.31221 (Russell et al., 1978).
View in article
Saiki, S., Imamura, H., Arakawa, M., Wada, K., Takagi, Y., Hayakawa, M., Shirai, K., Yano, H., Okamoto, C. (2017) The Small Carry-on Impactor (SCI) and the Hayabusa2 Impact Experiment. Space Science Reviews 208, 165–186. https://doi.org/10.1007/s11214-016-0297-5

Approximately 2 g of samples likely representing a mixture of materials from the surface and subsurface were collected into Chamber C at a site that was close to the crater formed by the Small Carry-on Impactor, a kinetic impact experiment of the Hayabusa2 mission (Saiki et al., 2017; Arakawa et al., 2020).
View in article
Simon, J.I., DePaolo, D.J. (2010) Stable calcium isotopic composition of meteorites and rocky planets. Earth and Planetary Science Letters 289, 457–466. https://doi.org/10.1016/j.epsl.2009.11.035

This range is likely related to a combination of the variable modal abundances of refractory inclusions among CC (Hezel et al., 2008) that can be enriched in the lighter Ca isotopes by several per mille (Niederer and Papanastassiou, 1984; Huang et al., 2012) and the heterogeneous distribution of carbonates (Simon and DePaolo, 2010; Valdes et al., 2014; Dauphas and Pourmand, 2015).
View in article
Neither the Ryugu samples nor the meteorites analysed here show any 40Ca anomalies (after age corrections), which is consistent with the literature (e.g., Simon and DePaolo, 2010; Huang and Jacobsen, 2017).
View in article
Calcium isotopic data from this study and literature (Simon and DePaolo, 2010; Valdes et al., 2014; Amsellem et al., 2017; Huang and Jacobsen, 2017).
View in article
Simon, J.I., Jordan, M.J., Tappa, A., Schauble, E., Kohll, E., Young, E.D. (2017) Calcium and titanium isotope fractionation in refractory inclusions: Tracers of condensation and inheritance in the early solar system. Earth and Planetary Science Letters 472, 277–288. https://doi.org/10.1016/j.epsl.2017.05.002
Tachibana, S., Sawada, H., Okazaki, R., Takano, Y., Sakamoto, K. et al. (2022) Pebbles and sand on asteroid (162173) Ryugu: In situ observation and particles returned to Earth. Science 375, 1011–1016. https://doi.org/10.1126/science.abj8624

In December 2020, the JAXA Hayabusa2 spacecraft returned to Earth with the first samples collected from a Cb-type asteroid, (162173) Ryugu (Tachibana et al., 2022; Yada et al., 2022).
View in article
The samples returned by the Hayabusa2 spacecraft consist of ∼5 g of materials from the Ryugu asteroid recovered during two touchdowns (Tachibana et al., 2022; Yada et al., 2022).
View in article
Valdes, M., Moreira, M., Foriel, J., Moynier, F. (2014) The nature of Earth’s building blocks as revealed by calcium isotopes. Earth and Planetary Science Letters 394, 135–145. https://doi.org/10.1016/j.epsl.2014.02.052

This range is likely related to a combination of the variable modal abundances of refractory inclusions among CC (Hezel et al., 2008) that can be enriched in the lighter Ca isotopes by several per mille (Niederer and Papanastassiou, 1984; Huang et al., 2012) and the heterogeneous distribution of carbonates (Simon and DePaolo, 2010; Valdes et al., 2014; Dauphas and Pourmand, 2015).
View in article
Calcium isotopic data from this study and literature (Simon and DePaolo, 2010; Valdes et al., 2014; Amsellem et al., 2017; Huang and Jacobsen, 2017).
View in article
The two heaviest Ca isotopic compositions for Orgueil samples from Valdes et al. (2014) (0.65 ± 0.17 ‰, 2 s.d.) and Huang and Jacobsen (2017) (0.75 ± 0.11 ‰, 2 s.d.) may reflect different proportions of carbonates.
View in article
Yada, T., Abe, M., Okada, T., Nakato, A., Yogata, K. et al. (2022) Preliminary analysis of the Hayabusa2 samples returned from C-type asteroid Ryugu. Nature Astronomy 6, 214–220. https://doi.org/10.1038/s41550-021-01550-6

In December 2020, the JAXA Hayabusa2 spacecraft returned to Earth with the first samples collected from a Cb-type asteroid, (162173) Ryugu (Tachibana et al., 2022; Yada et al., 2022).
View in article
The samples returned by the Hayabusa2 spacecraft consist of ∼5 g of materials from the Ryugu asteroid recovered during two touchdowns (Tachibana et al., 2022; Yada et al., 2022).
View in article
Yokoyama, T., Nagashima, K., Nakai, I., Young, E.D., Abe, Y. et al. (2022) Samples returned from the asteroid Ryugu are similar to Ivuna-type carbonaceous meteorites. Science https://doi.org/10.1126/science.abn7850

In particular, their bulk Cr and Ti isotopic signatures and the chemical abundances of most elements are within the range of CI chondrites (Nakamura E. et al., 2022; Yokoyama et al., 2022).
View in article
Given that the Ryugu samples did not experience any terrestrial alteration, they are likely the chemically most pristine Solar System samples available estimating the original isotopic compositions of most elements in the Solar System (Yokoyama et al., 2022).
View in article
Despite many aspects of similarities with the CIs, the Ryugu samples display an apparent excess of Ca by over 50 %, which may be related to a heterogeneous distribution of carbonates (dolomite and calcite) (Nakamura T. et al., 2022; Yokoyama et al., 2022) between Ryugu, Orgueil and other CIs.
View in article
All samples were dissolved in PFA vials with a mixture of concentrated HF and HNO3 at the Tokyo Institute of Technology (Yokoyama et al., 2022).
View in article
The radiogenic ingrowth on 40Ca from 40K decay was corrected using the K and Ca abundances of the samples (from Yokoyama et al., 2022) and δ44/40Ca (age corrected) ratios are also presented in Table 1.
View in article
If the excess Ca in A0106-A0107 is mainly stored in secondary carbonates (Yokoyama et al., 2022 and our discussion below), then these carbonates must contribute significantly to the bulk Ca isotopic composition.
View in article
The Ca/Al ratios of bulk Chamber A and Chamber C samples from Yokoyama et al. (2022) are used for Ryugu samples in this work and are taken from the average value in Barrat et al. (2012) for Orgueil, as it was not available for specific samples used here.
View in article
This explanation is consistent with an episode of fluid circulation and carbonate precipitation in the Ryugu samples that occurred 2.5 to 5 Myr after CAIs formation, as dated using 53Mn-53Cr chronometry in carbonate phases (Nakamura E. et al., 2022; Yokoyama et al., 2022).
View in article
top
Supplementary Information
The Supplementary Information includes:
Download the Supplementary Information (PDF)