An isotopically light nitrogen reservoir in the ocean: evidence from ferromanganese crusts
Affiliations | Corresponding Author | Cite as | Funding information- Share this article
-
Article views:282Cumulative count of HTML views and PDF downloads.
- Download Citation
- Rights & Permissions
top
Abstract

Figures
![]() Figure 1 (a) Total nitrogen (TN) versus δ15Nbulk. (b) TN versus total inorganic carbon (TIC). (c) TN versus total organic carbon (TOC). The hydrogenetic crusts are relatively depleted in δ15Nbulk and TN but enriched in TIC. | ![]() Figure 2 (a) δ13Corg versus δ15Nbulk. (b) δ13Ccarb versus δ15Nbulk. The hydrogenetic crusts tend to be depleted in δ15Nbulk relative to average seawater (blue line), possibly reflecting intake of isotopically light NO2− generated within sediments from NH4+ oxidation. Slight enrichment in δ15Nbulk in diagenetic oxides may reflect retention of isotopically heavy NH4+ after partial oxidation. | ![]() Figure 3 Proposed mechanism of partial NH4+ oxidation to NO3− via NO2− and associated Rayleigh fractionation. (a) Fractionation (ɛ = δ15Nreactant – δ15Nproduct) of 25 ‰, maximum net fractionation for NH4+ → NO3−. (b) Fractionation of 15 ‰, smallest possible fractionation to explain the data. Reactions on the right show that partial oxidation could also generate light NO2−. Light grey shading = range of δ15N values seen in hydrogenetic crusts; dark grey shading = range of δ15N values seen in diagenetic and hydrogenetic nodules. | ![]() Figure 4 Environmental sketch. Dead biomass (dotted brown lines) releases ammonium during degradation, which is partially oxidised to nitrite. Some of this nitrite is trapped in FeMn oxides by adsorption and possibly by assimilation into fresh biomass (dotted pale green lines). (a) In hydrogenetic crusts, the residual 15N-enriched ammonium is lost to the water column by diffusion. (b) In diagenetic and hydrogenetic nodules, some of the ammonium is retained in clay minerals, leading to higher bulk δ15N values and TN abundances. |
Figure 1 | Figure 2 | Figure 3 | Figure 4 |
top
Introduction
Ferromanganese (FeMn) oxide deposits in the deep ocean have received increasing attention over the past two decades as they have become recognised as reservoirs of critical metals with potentially economic value (Hein et al., 2000
Hein, J.R., Koschinsky, A., Bau, M., Manheim, T., Kang, J.-K., Roberts, L. (2000) Cobalt rich ferromanganese crusts in the Pacific. In: Cronan, D.S. (Ed.) Handbook of Marine Mineral Deposits. CRC Press, Boca Raon, Florida, 239–279. https://doi.org/10.1201/9780203752760-9
; Lusty et al., 2018Lusty, P.A., Hein, J.R., Josso, P. (2018) Formation and occurrence of ferromanganese crusts: earth’s storehouse for critical metals. Elements 14, 313–318. https://doi.org/10.2138/gselements.14.5.313
). There are three “end member types” of marine FeMn oxide deposits: hydrogenetic crusts and nodules that form very slowly (at a rate of only a few mm/Myr) on exposed rock surfaces at the sediment-water interface; diagenetic nodules that form from pore waters within marine sediments around a nucleus such as a rock fragment; and hydrothermal precipitates that may form as plume fallout or within the sediment (e.g., Koschinsky and Hein, 2017Koschinsky, A., Hein, J.R. (2017) Marine ferromanganese encrustations: archives of changing oceans. Elements 13, 177–182. https://doi.org/10.2113/gselements.13.3.177
, and references therein). Rare earths and yttrium (REY) concentrations and REY patterns can be used to discriminate between these three genetic pathways, which further attests to their distinct fluid sources, growth rates, and formation mechanisms (Bau et al., 2014Bau, M., Schmidt, K., Koschinsky, A., Hein, J., Kuhn, T., Usui, A. (2014) Discriminating between different genetic types of marine ferro-manganese crusts and nodules based on rare earth elements and yttrium. Chemical Geology 381, 1–9. https://doi.org/10.1016/j.chemgeo.2014.05.004
). One potentially important factor in the formation of these deposits may be microbial activity, but its role is still unclear. Manganese oxidisers have been detected with genomic techniques (Shiraishi et al., 2016Shiraishi, F., Mitsunobu, S., Suzuki, K., Hoshino, T., Morono, Y., Inagaki, F. (2016) Dense microbial community on a ferromanganese nodule from the ultra-oligotrophic South Pacific Gyre: Implications for biogeochemical cycles. Earth and Planetary Science Letters 447, 10–20. https://doi.org/10.1016/j.epsl.2016.04.021
). The presence of biomass within the FeMn oxides therefore opens the possibility of investigating these deposits as novel archives of nitrogen in the sedimentary record. So far, most studies of nitrogen isotopes and abundances in marine sediments have focused on mudrocks (Ader et al., 2016Ader, M., Thomazo, C., Sansjofre, P., Busigny, V., Papineau, D., Laffont, R., Cartigny, P., Halverson, G.P. (2016) Interpretation of the nitrogen isotopic composition of Precambrian sedimentary rocks: Assumptions and perspectives. Chemical Geology 429, 93–110. https://doi.org/10.1016/j.chemgeo.2016.02.010
). To our knowledge, FeMn oxides have not previously been studied as geological N repositories. To fill this gap, we selected a suite of hydrogenetic and diagenetic FeMn crusts and nodules from the northern, eastern and southern Central Pacific and three hydrogenetic and mixed type hydrogenetic-diagenetic FeMn nodule certified reference materials from the Atlantic and Pacific (see Supplementary Information for a detailed description of the methods employed). Classification as hydrogenetic versus diagenetic is based on the REY concentration and distribution, following the approach outlined in detail by Bau et al. (2014)Bau, M., Schmidt, K., Koschinsky, A., Hein, J., Kuhn, T., Usui, A. (2014) Discriminating between different genetic types of marine ferro-manganese crusts and nodules based on rare earth elements and yttrium. Chemical Geology 381, 1–9. https://doi.org/10.1016/j.chemgeo.2014.05.004
. For further discussion of some of these samples see also Bau et al. (1996)Bau, M., Koschinsky, A., Dulski, P., Hein, J.R. (1996) Comparison of the partitioning behaviours of yttrium, rare earth elements, and titanium between hydrogenetic marine ferromanganese crusts and seawater. Geochimica et Cosmochimica Acta 60, 1709–1725. https://doi.org/10.1016/0016-7037(96)00063-4
, Schier et al. (2021)Schier, K., Ernst, D.M., de Sousa, I.M.C., Garbe-Schönberg, D., Kuhn, T., Hein, J.R., Bau, M. (2021) Gallium-aluminum systematics of marine hydrogenetic ferromanganese crusts: Inter-oceanic differences and fractionation during scavenging. Geochimica et Cosmochimica Acta 310, 187–204. https://doi.org/10.1016/j.gca.2021.05.019
and Ernst et al. (2022)Ernst, D.M., Schier, K., Garbe-Schönberg, D., Bau, M. (2022) Fractionation of germanium and silicon during scavenging from seawater by marine Fe (oxy) hydroxides: Evidence from hydrogenetic ferromanganese crusts and nodules. Chemical Geology 595, https://doi.org/10.1016/j.chemgeo.2022.120791
.top
Results
We find that FeMn oxide nodules, both diagenetic and hydrogenetic, tend to be enriched in total nitrogen (TN) content by a factor of 2.3 on average and display heavier bulk δ15N values (+3 ‰ to +12 ‰) compared to the hydrogenetic crusts (−12 ‰ to +3 ‰) (Table S-1, Fig. 1a). In contrast, the hydrogenetic crusts are enriched in total inorganic carbon (TIC) by a mean factor of 2.6 (Fig. 1b). Total organic carbon (TOC) does not vary systematically between crusts and nodules (Fig. 1c), but the hydrogenetic crusts express relatively lower organic carbon isotope values (δ13Corg = −27.8 ± 0.6 ‰ versus −22.6 ± 2.0 ‰) (Fig. 2a). Carbonate carbon isotopes (δ13Ccarb) do not vary systematically, but all samples fall below −8 ‰ and are thus depleted relative to open marine carbonate which falls near 0 ‰ (Fig. 2b). Our results for the certified reference materials NOD-P1, NOD-A1 and JMn-1 fall within the respective range observed for the other samples (Fig. 1a).
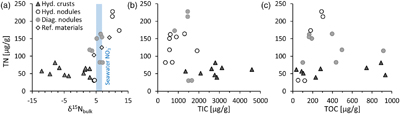
Figure 1 (a) Total nitrogen (TN) versus δ15Nbulk. (b) TN versus total inorganic carbon (TIC). (c) TN versus total organic carbon (TOC). The hydrogenetic crusts are relatively depleted in δ15Nbulk and TN but enriched in TIC.

Figure 2 (a) δ13Corg versus δ15Nbulk. (b) δ13Ccarb versus δ15Nbulk. The hydrogenetic crusts tend to be depleted in δ15Nbulk relative to average seawater (blue line), possibly reflecting intake of isotopically light NO2− generated within sediments from NH4+ oxidation. Slight enrichment in δ15Nbulk in diagenetic oxides may reflect retention of isotopically heavy NH4+ after partial oxidation.
top
Discussion
The nitrogen isotopic composition of some of the hydrogenetic crusts is unusually depleted in 15N. For comparison, average marine mud from the modern ocean clusters around a mean of +5 ‰ to +6 ‰ (Tesdal et al., 2013
Tesdal, J.E., Galbraith, E.D., Kienast, M. (2013) Nitrogen isotopes in bulk marine sediment: linking seafloor observations with subseafloor records. Biogeosciences 10, 101–118. https://doi.org/10.5194/bg-10-101-2013
), which reflects the isotopic composition of seawater nitrate – the major nitrogen source for algae that is captured in sediments via biomass burial. However, values in the negative range are rare throughout the siliciclastic rock record (Ader et al., 2016Ader, M., Thomazo, C., Sansjofre, P., Busigny, V., Papineau, D., Laffont, R., Cartigny, P., Halverson, G.P. (2016) Interpretation of the nitrogen isotopic composition of Precambrian sedimentary rocks: Assumptions and perspectives. Chemical Geology 429, 93–110. https://doi.org/10.1016/j.chemgeo.2016.02.010
). There are four possible mechanisms that could explain such low δ15N values:(1) Biological nitrogen fixation by alternative nitrogenases. When dissolved N becomes biologically limiting, some microbes are able to convert N2 into ammonium, a process known as nitrogen fixation that is catalysed by the enzyme nitrogenase. Most nitrogenases contain Mo at a catalytic centre, and those impart an isotopic fractionation (ɛ = δ15Nreactant – δ15Nproduct) of 1–2 ‰ on the resulting biomass. Under Mo-depleted conditions, V or Fe can be used instead, and these so called alternative nitrogenases impart larger fractionations of up to 8 ‰ (Zhang et al., 2014
Zhang, X., Sigman, D.M., Morel, F.M., Kraepiel, A.M. (2014) Nitrogen isotope fractionation by alternative nitrogenases and past ocean anoxia. Proceedings of the National Academy of Sciences 111, 4782–4787. https://doi.org/10.1073/pnas.1402976111
). However, it is unlikely that this metabolism caused the low δ15N values in our samples, because the deep ocean is enriched in both nitrate and Mo, meaning that nitrogen fixation is not required, and, if necessary, the more efficient Mo-based nitrogenase would likely be preferred.(2) Dissimilatory nitrate reduction to ammonium (DNRA). Given the high abundance of nitrate in the deep ocean with an isotopic composition of around +5 ‰ to +6 ‰, it is conceivable that the low δ15N values reflect ammonium generated by DNRA, which imparts a fractionation of up to 30 ‰ (McCready et al., 1983
McCready, R.G.L., Gould, W.D., Barendregt, R.W. (1983) Nitrogen isotope fractionation during the reduction of NO3− to NH4+ by Desulfovibrio sp. Canadian Journal of Microbiology 29, 231–234. https://doi.org/10.1139/m83-038
). However, this metabolism is unlikely to take place within an environment where Mn(IV) oxides are thermodynamically stable, because Mn has a high redox potential that is not compatible with nitrate reduction (Brookins, 1988Brookins, D.G. (1988) Eh-pH Diagrams for Geochemistry, Springer-Verlag, New York. https://doi.org/10.1007/978-3-642-73093-1
).(3) Partial assimilation of either ammonium or nitrate into biomass. It has been suggested that FeMn crusts are populated by microorganisms (e.g., Kato et al., 2019
Kato, S., Hirai, M., Ohkuma, M., Suzuki, K. (2019) Microbial metabolisms in an abyssal ferromanganese crust from the Takuyo-Daigo Seamount as revealed by metagenomics. PLoS ONE 14, p.e0224888. https://doi.org/10.1371/journal.pone.0224888
), and these could be fractionating 15N/14N ratios as they uptake nitrate (ɛ = 5–10‰) or ammonium (ɛ = 14–27‰) into their biomass (Casciotti, 2009Casciotti, K.L. (2009) Inverse kinetic isotope fractionation during bacterial nitrite oxidation. Geochimica et Cosmochimica Acta 73, 2061–2076. https://doi.org/10.1016/j.gca.2008.12.022
). Nitrate is readily available in seawater (30 μM on average; e.g., Webb, 2021Webb, P. (2021) Introduction to Oceanography, Pressbooks. Rebus Community: Montreal, QC, Canada, 393pp.
), and ammonium could potentially be supplied by decaying biomass underneath or within the FeMn crust. Both would likely have a starting composition of +5 ‰ to +6 ‰, as observed for marine nitrate and average marine sediments (Tesdal et al., 2013Tesdal, J.E., Galbraith, E.D., Kienast, M. (2013) Nitrogen isotopes in bulk marine sediment: linking seafloor observations with subseafloor records. Biogeosciences 10, 101–118. https://doi.org/10.5194/bg-10-101-2013
). Hence this mechanism offers a plausible explanation for the δ15N data; however, it does not account for the light δ13Ccarb values in the same samples.(4) Partial oxidation of ammonium (nitrification) to nitrite and nitrate. In the oxidising environment of the FeMn crusts, ammonium would likely undergo oxidation. This microbially catalysed reaction produces isotopically light nitrite and later nitrate in a second step, while the residual ammonium becomes enriched in 15N (Fig. 3). If the oxidation process does not go to completion, perhaps due to diffusion limited O2 migration into the crusts as described in sediments elsewhere (Morales et al., 2014
Morales, L.V., Granger, J., Chang, B.X., Prokopenko, M.G., Plessen, B., Gradinger, R., Sigman, D.M. (2014) Elevated 15N/14N in particulate organic matter, zooplankton, and diatom frustule-bound nitrogen in the ice-covered water column of the Bering Sea eastern shelf. Deep Sea Research Part II: Topical Studies in Oceanography 109, 100–111. https://doi.org/10.1016/j.dsr2.2014.05.008
), isotopically light nitrite/nitrate may accumulate and become incorporated into the crust, either by uptake into fresh biomass or by adsorption to the mineral surface (as documented for nitrate by Takematsu et al., 1990Takematsu, N., Sato, Y., Okabe, S., Usui, A. (1990) Uptake of selenium and other oxyanionic elements in marine ferromanganese concretions of different origins. Marine Chemistry 31, 271–283. https://doi.org/10.1016/0304-4203(90)90042-B
). This could plausibly explain the δ15N values of the hydrogenetic crusts. Indeed, nitrifying organisms of the phylum Thaumarchaea have been documented by molecular techniques from several deep marine FeMn oxides (e.g., Kato et al., 2019Kato, S., Hirai, M., Ohkuma, M., Suzuki, K. (2019) Microbial metabolisms in an abyssal ferromanganese crust from the Takuyo-Daigo Seamount as revealed by metagenomics. PLoS ONE 14, p.e0224888. https://doi.org/10.1371/journal.pone.0224888
; Bergo et al., 2021Bergo, N.M., Bendia, A.G., Ferreira, J.C.N., Murton, B.J., Brandini, F.P., Pellizari, V.H. (2021) Microbial diversity of deep-sea ferromanganese crust field in the Rio Grande Rise, Southwestern Atlantic Ocean. Microbial Ecology 82, 344–355. https://doi.org/10.1007/s00248-020-01670-y
).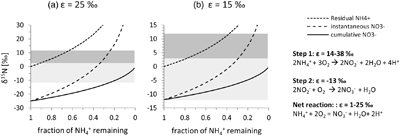
Figure 3 Proposed mechanism of partial NH4+ oxidation to NO3− via NO2− and associated Rayleigh fractionation. (a) Fractionation (ɛ = δ15Nreactant – δ15Nproduct) of 25 ‰, maximum net fractionation for NH4+ → NO3−. (b) Fractionation of 15 ‰, smallest possible fractionation to explain the data. Reactions on the right show that partial oxidation could also generate light NO2−. Light grey shading = range of δ15N values seen in hydrogenetic crusts; dark grey shading = range of δ15N values seen in diagenetic and hydrogenetic nodules.
It has been suggested that these nitrifying organisms are feeding on trace levels of ammonium dissolved in the deep ocean (Wuchter et al., 2006
Wuchter, C., Abbas, B., Coolen, M.J., Herfort, L., van Bleijswijk, J., Timmers, P., Strous, M., Teira, E., Herndl, G.J., Middelburg, J.J., Schouten, S. (2006) Archaeal nitrification in the ocean. Proceedings of the National Academy of Sciences 103, 12317–12322. https://doi.org/10.1073/pnas.0600756103
; Kato et al., 2019Kato, S., Hirai, M., Ohkuma, M., Suzuki, K. (2019) Microbial metabolisms in an abyssal ferromanganese crust from the Takuyo-Daigo Seamount as revealed by metagenomics. PLoS ONE 14, p.e0224888. https://doi.org/10.1371/journal.pone.0224888
). While this appears possible, it would, however, leave the light δ13Ccarb values unexplained. Such light δ13Ccarb values most likely reflect in situ oxidation of organic matter to dissolved inorganic carbon (DIC) within pore waters. This diagenetic DIC pool would inherit the isotopic composition of local biomass (<−20 ‰, Fig. 2a), such that variable mixing with seawater (δ13C ≈ 0 ‰) can explain the observed δ13Ccarb values between −28 ‰ and −8 ‰ (Fig. 2b). The carbon isotope data are thus evidence for degradation of older biomass from within the FeMn oxides. This biomass is the most likely source of ammonium for nitrification, because the Redfield ratio of average marine organisms dictates that 1 mole of N is released for every 7–10 mole of organic C (Godfrey and Glass, 2011Godfrey, L.V., Glass, J.B. (2011) The geochemical record of the ancient nitrogen cycle, nitrogen isotopes, and metal cofactors. Methods in Enzymology 486, 483–506. https://doi.org/10.1016/B978-0-12-381294-0.00022-5
). We note that δ15Nbulk and δ13Ccarb are not directly correlated, but as the isotopically light N and C are likely associated with different minerals and undergo variable mixing with isotopically heavier phases, a correlation cannot necessarily be expected.Overall, the most plausible scenario for the N-C isotope systematics observed in the hydrogenetic FeMn crusts is, therefore, the presence of a benthic biosphere, where older biomass underwent oxidation and nitrification, catalysed by active organisms. Some of the products (including isotopically light nitrite/nitrate) were re-assimilated into fresh biomass and/or adsorbed to mineral surfaces, while the oxidised organic carbon was partly redeposited as carbonate. This interpretation is consistent with biomolecular evidence of nitrifiers from FeMn crusts worldwide (see above). However, it leaves open the question as to what happened to the residual isotopically heavy ammonium. We speculate that in the case of the isotopically lightest hydrogenetic FeMn crusts this ammonium was diffusively lost to the water column. This may indicate that the preferential retention of nitrite/nitrate within the FeMn crust was driven by adsorption rather than biological uptake, because the latter would typically prefer ammonium. Nitrite adsorption on FeMn oxides has to our knowledge not been studied systematically, but we note that empirical data from one study suggest concentrations of around 50 μg·g-1 of nitrate at equilibrium with seawater (Takematsu et al., 1990
Takematsu, N., Sato, Y., Okabe, S., Usui, A. (1990) Uptake of selenium and other oxyanionic elements in marine ferromanganese concretions of different origins. Marine Chemistry 31, 271–283. https://doi.org/10.1016/0304-4203(90)90042-B
), which is consistent with our data.In the case of the diagenetic and hydrogenetic nodules, we also see low δ13Ccarb values indicative of biomass oxidation; however, δ15N shows no evidence of light nitrite/nitrate retention. Instead, δ15N values of up to 12 ‰ and comparatively high TN abundances may indicate that isotopically heavy ammonium (i.e. the residuum after partial nitrification) was preferentially retained (Fig. 3), possibly due to a higher clay or active biomass content within these samples. Ammonium (NH4+) has a similar ionic radius to K+, which allows it to substitute into potassic phyllosilicates during diagenesis (Müller, 1977
Müller, P.J. (1977) CN ratios in Pacific deep-sea sediments: Effect of inorganic ammonium and organic nitrogen compounds sorbed by clays. Geochimica et Cosmochimica Acta 41, 765–776. https://doi.org/10.1016/0016-7037(77)90047-3
). Retention of isotopically heavy ammonium in the clay matrix would probably have overwhelmed the proportion of adsorbed isotopically light nitrite/nitrate, such that the bulk average δ15N value of the nodular samples is positive. Further work is needed to test this hypothesis. We also note the systematically higher δ13Corg values within nodules compared to crusts (Fig. 3a), which likely point towards an ecosystem with different carbon fixation pathways. This interpretation is broadly consistent with a distinct microenvironment with differing nutrient inventories.top
Conclusions
In summary, our data reveal novel insights into biological processes that take place within FeMn oxide deposits in the deep sea, including biological oxidation of biomass in situ, which led to the formation of isotopically light DIC and nitrite/nitrate (Figs. 3, 4). The latter may be trapped by adsorption to oxide minerals. Our results thus uncover a mechanism for generating a previously unknown repository of isotopically light nitrogen in marine sediments. However, it is unlikely that this repository contributes significantly to the global mass balance of N burial from the ocean. For example, assuming a global average mass accumulation rate of 24.8 × 109 g for FeMn oxides in the deep ocean (e.g., Ernst et al., 2022
Ernst, D.M., Schier, K., Garbe-Schönberg, D., Bau, M. (2022) Fractionation of germanium and silicon during scavenging from seawater by marine Fe (oxy) hydroxides: Evidence from hydrogenetic ferromanganese crusts and nodules. Chemical Geology 595, https://doi.org/10.1016/j.chemgeo.2022.120791
) with an average concentration of TN of 57 ± 12 μg·g-1 (Table S-1) would lead to a N burial flux of 1.4·106 g·yr-1. For comparison, the amount of N buried in siliciclastic marine sediments globally with a mean concentration of 560 ± 230 μg·g-1 (Johnson and Goldblatt, 2015Johnson, B., Goldblatt, C. (2015) The Nitrogen budget of Earth. Earth-Science Reviews 148, 150–173. https://doi.org/10.1016/j.earscirev.2015.05.006
) at a sedimentation rate of 1016 g·yr-1 (Gregor, 1985Gregor, C.B. (1985) The mass-age distribution of Phanerozoic sediments. In: Snelling, N.J. (Ed.) The chronology of the geologic record. The Geological Society of London, London, UK, 284–289. https://doi.org/10.1144/GSL.MEM.1985.010.01.22
) yields an N burial flux of 5.6·1012 g·yr-1 and thus dominates as the major sink. However, our study uncovers a novel archive of microbial activity that may be preserved in the rock record and contribute reconstructions of biological evolution over Earth history, or serve as a biosignature in Martian settings.
Figure 4 Environmental sketch. Dead biomass (dotted brown lines) releases ammonium during degradation, which is partially oxidised to nitrite. Some of this nitrite is trapped in FeMn oxides by adsorption and possibly by assimilation into fresh biomass (dotted pale green lines). (a) In hydrogenetic crusts, the residual 15N-enriched ammonium is lost to the water column by diffusion. (b) In diagenetic and hydrogenetic nodules, some of the ammonium is retained in clay minerals, leading to higher bulk δ15N values and TN abundances.
top
Acknowledgements
EES acknowledges funding from a NERC Frontiers grant (NE/V010824/1). MB acknowledges funding from Deutsche Forschungsgemeinschaft (DFG SPP1833 grant BA-2289/8-1). We thank Claudine Stirling for editorial handling and two anonymous reviewers for constructive feedback that improved the manuscript.
Editor: Claudine Stirling
top
References
Ader, M., Thomazo, C., Sansjofre, P., Busigny, V., Papineau, D., Laffont, R., Cartigny, P., Halverson, G.P. (2016) Interpretation of the nitrogen isotopic composition of Precambrian sedimentary rocks: Assumptions and perspectives. Chemical Geology 429, 93–110. https://doi.org/10.1016/j.chemgeo.2016.02.010

So far, most studies of nitrogen isotopes and abundances in marine sediments have focused on mudrocks (Ader et al., 2016).
View in article
However, values in the negative range are rare throughout the siliciclastic rock record (Ader et al., 2016).
View in article
Bau, M., Koschinsky, A., Dulski, P., Hein, J.R. (1996) Comparison of the partitioning behaviours of yttrium, rare earth elements, and titanium between hydrogenetic marine ferromanganese crusts and seawater. Geochimica et Cosmochimica Acta 60, 1709–1725. https://doi.org/10.1016/0016-7037(96)00063-4

For further discussion of some of these samples see also Bau et al. (1996), Schier et al. (2021) and Ernst et al. (2022).
View in article
Bau, M., Schmidt, K., Koschinsky, A., Hein, J., Kuhn, T., Usui, A. (2014) Discriminating between different genetic types of marine ferro-manganese crusts and nodules based on rare earth elements and yttrium. Chemical Geology 381, 1–9. https://doi.org/10.1016/j.chemgeo.2014.05.004

Rare earths and yttrium (REY) concentrations and REY patterns can be used to discriminate between these three genetic pathways, which further attests to their distinct fluid sources, growth rates, and formation mechanisms (Bau et al., 2014).
View in article
Classification as hydrogenetic versus diagenetic is based on the REY concentration and distribution, following the approach outlined in detail by Bau et al. (2014).
View in article
Bergo, N.M., Bendia, A.G., Ferreira, J.C.N., Murton, B.J., Brandini, F.P., Pellizari, V.H. (2021) Microbial diversity of deep-sea ferromanganese crust field in the Rio Grande Rise, Southwestern Atlantic Ocean. Microbial Ecology 82, 344–355. https://doi.org/10.1007/s00248-020-01670-y

Indeed, nitrifying organisms of the phylum Thaumarchaea have been documented by molecular techniques from several deep marine FeMn oxides (e.g., Kato et al., 2019; Bergo et al., 2021).
View in article
Brookins, D.G. (1988) Eh-pH Diagrams for Geochemistry, Springer-Verlag, New York. https://doi.org/10.1007/978-3-642-73093-1

However, this metabolism is unlikely to take place within an environment where Mn(IV) oxides are thermodynamically stable, because Mn has a high redox potential that is not compatible with nitrate reduction (Brookins, 1988).
View in article
Casciotti, K.L. (2009) Inverse kinetic isotope fractionation during bacterial nitrite oxidation. Geochimica et Cosmochimica Acta 73, 2061–2076. https://doi.org/10.1016/j.gca.2008.12.022

It has been suggested that FeMn crusts are populated by microorganisms (e.g., Kato et al., 2019), and these could be fractionating 15N/14N ratios as they uptake nitrate (ɛ = 5–10‰) or ammonium (ɛ = 14–27‰) into their biomass (Casciotti, 2009).
View in article
Ernst, D.M., Schier, K., Garbe-Schönberg, D., Bau, M. (2022) Fractionation of germanium and silicon during scavenging from seawater by marine Fe (oxy) hydroxides: Evidence from hydrogenetic ferromanganese crusts and nodules. Chemical Geology 595, https://doi.org/10.1016/j.chemgeo.2022.120791

For further discussion of some of these samples see also Bau et al. (1996), Schier et al. (2021) and Ernst et al. (2022).
View in article
For example, assuming a global average mass accumulation rate of 24.8 × 109 g for FeMn oxides in the deep ocean (e.g., Ernst et al., 2022) with an average concentration of TN of 57 ± 12 μg·g-1 (Table S-1) would lead to a N burial flux of 1.4·106 g·yr-1
View in article
Godfrey, L.V., Glass, J.B. (2011) The geochemical record of the ancient nitrogen cycle, nitrogen isotopes, and metal cofactors. Methods in Enzymology 486, 483–506. https://doi.org/10.1016/B978-0-12-381294-0.00022-5

This biomass is the most likely source of ammonium for nitrification, because the Redfield ratio of average marine organisms dictates that 1 mole of N is released for every 7–10 mole of organic C (Godfrey and Glass, 2011).
View in article
Gregor, C.B. (1985) The mass-age distribution of Phanerozoic sediments. In: Snelling, N.J. (Ed.) The chronology of the geologic record. The Geological Society of London, London, UK, 284–289. https://doi.org/10.1144/GSL.MEM.1985.010.01.22

For comparison, the amount of N buried in siliciclastic marine sediments globally with a mean concentration of 560 ± 230 μg·g-1 (Johnson and Goldblatt, 2015) at a sedimentation rate of 1016 g·yr-1 (Gregor, 1985) yields an N burial flux of 5.6·1012 g·yr-1 and thus dominates as the major sink.
View in article
Hein, J.R., Koschinsky, A., Bau, M., Manheim, T., Kang, J.-K., Roberts, L. (2000) Cobalt rich ferromanganese crusts in the Pacific. In: Cronan, D.S. (Ed.) Handbook of Marine Mineral Deposits. CRC Press, Boca Raon, Florida, 239–279. https://doi.org/10.1201/9780203752760-9

Ferromanganese (FeMn) oxide deposits in the deep ocean have received increasing attention over the past two decades as they have become recognised as reservoirs of critical metals with potentially economic value (Hein et al., 2000; Lusty et al., 2018).
View in article
Johnson, B., Goldblatt, C. (2015) The Nitrogen budget of Earth. Earth-Science Reviews 148, 150–173. https://doi.org/10.1016/j.earscirev.2015.05.006

For comparison, the amount of N buried in siliciclastic marine sediments globally with a mean concentration of 560 ± 230 μg·g-1 (Johnson and Goldblatt, 2015) at a sedimentation rate of 1016 g·yr-1 (Gregor, 1985) yields an N burial flux of 5.6·1012 g·yr-1 and thus dominates as the major sink.
View in article
Kato, S., Hirai, M., Ohkuma, M., Suzuki, K. (2019) Microbial metabolisms in an abyssal ferromanganese crust from the Takuyo-Daigo Seamount as revealed by metagenomics. PLoS ONE 14, p.e0224888. https://doi.org/10.1371/journal.pone.0224888

It has been suggested that FeMn crusts are populated by microorganisms (e.g., Kato et al., 2019), and these could be fractionating 15N/14N ratios as they uptake nitrate (ɛ = 5–10‰) or ammonium (ɛ = 14–27‰) into their biomass (Casciotti, 2009).
View in article
Indeed, nitrifying organisms of the phylum Thaumarchaea have been documented by molecular techniques from several deep marine FeMn oxides (e.g., Kato et al., 2019; Bergo et al., 2021).
View in article
It has been suggested that these nitrifying organisms are feeding on trace levels of ammonium dissolved in the deep ocean (Wuchter et al., 2006; Kato et al., 2019).
View in article
Koschinsky, A., Hein, J.R. (2017) Marine ferromanganese encrustations: archives of changing oceans. Elements 13, 177–182. https://doi.org/10.2113/gselements.13.3.177

There are three “end member types” of marine FeMn oxide deposits: hydrogenetic crusts and nodules that form very slowly (at a rate of only a few mm/Myr) on exposed rock surfaces at the sediment-water interface; diagenetic nodules that form from pore waters within marine sediments around a nucleus such as a rock fragment; and hydrothermal precipitates that may form as plume fallout or within the sediment (e.g., Koschinsky and Hein, 2017, and references therein).
View in article
Lusty, P.A., Hein, J.R., Josso, P. (2018) Formation and occurrence of ferromanganese crusts: earth’s storehouse for critical metals. Elements 14, 313–318. https://doi.org/10.2138/gselements.14.5.313

Ferromanganese (FeMn) oxide deposits in the deep ocean have received increasing attention over the past two decades as they have become recognised as reservoirs of critical metals with potentially economic value (Hein et al., 2000; Lusty et al., 2018).
View in article
McCready, R.G.L., Gould, W.D., Barendregt, R.W. (1983) Nitrogen isotope fractionation during the reduction of NO3− to NH4+ by Desulfovibrio sp. Canadian Journal of Microbiology 29, 231–234. https://doi.org/10.1139/m83-038

Given the high abundance of nitrate in the deep ocean with an isotopic composition of around +5 ‰ to +6 ‰, it is conceivable that the low δ15N values reflect ammonium generated by DNRA, which imparts a fractionation of up to 30 ‰ (McCready et al., 1983).
View in article
Morales, L.V., Granger, J., Chang, B.X., Prokopenko, M.G., Plessen, B., Gradinger, R., Sigman, D.M. (2014) Elevated 15N/14N in particulate organic matter, zooplankton, and diatom frustule-bound nitrogen in the ice-covered water column of the Bering Sea eastern shelf. Deep Sea Research Part II: Topical Studies in Oceanography 109, 100–111. https://doi.org/10.1016/j.dsr2.2014.05.008

If the oxidation process does not go to completion, perhaps due to diffusion limited O2 migration into the crusts as described in sediments elsewhere (Morales et al., 2014), isotopically light nitrite/nitrate may accumulate and become incorporated into the crust, either by uptake into fresh biomass or by adsorption to the mineral surface (as documented for nitrate by Takematsu et al., 1990).
View in article
Müller, P.J. (1977) CN ratios in Pacific deep-sea sediments: Effect of inorganic ammonium and organic nitrogen compounds sorbed by clays. Geochimica et Cosmochimica Acta 41, 765–776. https://doi.org/10.1016/0016-7037(77)90047-3

Instead, δ15N values of up to 12 ‰ and comparatively high TN abundances may indicate that isotopically heavy ammonium (i.e. the residuum after partial nitrification) was preferentially retained (Fig. 3), possibly due to a higher clay or active biomass content within these samples. Ammonium (NH4+) has a similar ionic radius to K+, which allows it to substitute into potassic phyllosilicates during diagenesis (Müller, 1977).
View in article
Schier, K., Ernst, D.M., de Sousa, I.M.C., Garbe-Schönberg, D., Kuhn, T., Hein, J.R., Bau, M. (2021) Gallium-aluminum systematics of marine hydrogenetic ferromanganese crusts: Inter-oceanic differences and fractionation during scavenging. Geochimica et Cosmochimica Acta 310, 187–204. https://doi.org/10.1016/j.gca.2021.05.019

For further discussion of some of these samples see also Bau et al. (1996), Schier et al. (2021) and Ernst et al. (2022).
View in article
Shiraishi, F., Mitsunobu, S., Suzuki, K., Hoshino, T., Morono, Y., Inagaki, F. (2016) Dense microbial community on a ferromanganese nodule from the ultra-oligotrophic South Pacific Gyre: Implications for biogeochemical cycles. Earth and Planetary Science Letters 447, 10–20. https://doi.org/10.1016/j.epsl.2016.04.021

Manganese oxidisers have been detected with genomic techniques (Shiraishi et al., 2016).
View in article
Takematsu, N., Sato, Y., Okabe, S., Usui, A. (1990) Uptake of selenium and other oxyanionic elements in marine ferromanganese concretions of different origins. Marine Chemistry 31, 271–283. https://doi.org/10.1016/0304-4203(90)90042-B

If the oxidation process does not go to completion, perhaps due to diffusion limited O2 migration into the crusts as described in sediments elsewhere (Morales et al., 2014), isotopically light nitrite/nitrate may accumulate and become incorporated into the crust, either by uptake into fresh biomass or by adsorption to the mineral surface (as documented for nitrate by Takematsu et al., 1990).
View in article
Nitrite adsorption on FeMn oxides has to our knowledge not been studied systematically, but we note that empirical data from one study suggest concentrations of around 50 μg·g-1 of nitrate at equilibrium with seawater (Takematsu et al., 1990), which is consistent with our data.
View in article
Tesdal, J.E., Galbraith, E.D., Kienast, M. (2013) Nitrogen isotopes in bulk marine sediment: linking seafloor observations with subseafloor records. Biogeosciences 10, 101–118. https://doi.org/10.5194/bg-10-101-2013

For comparison, average marine mud from the modern ocean clusters around a mean of +5 ‰ to +6 ‰ (Tesdal et al., 2013), which reflects the isotopic composition of seawater nitrate – the major nitrogen source for algae that is captured in sediments via biomass burial.
View in article
Both would likely have a starting composition of +5 ‰ to +6 ‰, as observed for marine nitrate and average marine sediments (Tesdal et al., 2013).
View in article
Webb, P. (2021) Introduction to Oceanography, Pressbooks. Rebus Community: Montreal, QC, Canada, 393pp.

Nitrate is readily available in seawater (30 μM on average; e.g., Webb, 2021), and ammonium could potentially be supplied by decaying biomass underneath or within the FeMn crust.
View in article
Wuchter, C., Abbas, B., Coolen, M.J., Herfort, L., van Bleijswijk, J., Timmers, P., Strous, M., Teira, E., Herndl, G.J., Middelburg, J.J., Schouten, S. (2006) Archaeal nitrification in the ocean. Proceedings of the National Academy of Sciences 103, 12317–12322. https://doi.org/10.1073/pnas.0600756103

It has been suggested that these nitrifying organisms are feeding on trace levels of ammonium dissolved in the deep ocean (Wuchter et al., 2006; Kato et al., 2019).
View in article
Zhang, X., Sigman, D.M., Morel, F.M., Kraepiel, A.M. (2014) Nitrogen isotope fractionation by alternative nitrogenases and past ocean anoxia. Proceedings of the National Academy of Sciences 111, 4782–4787. https://doi.org/10.1073/pnas.1402976111

Under Mo-depleted conditions, V or Fe can be used instead, and these so called alternative nitrogenases impart larger fractionations of up to 8 ‰ (Zhang et al., 2014).
View in article
top
Supplementary Information
The Supplementary Information includes:
Download the Supplementary Information (PDF)
Figures
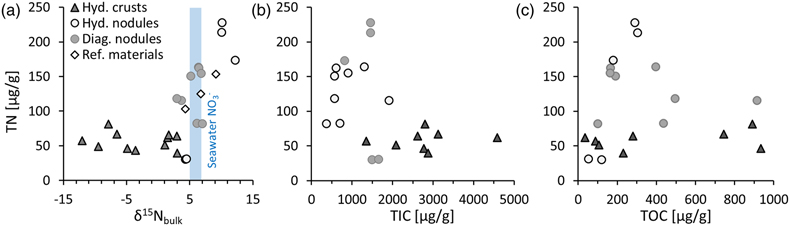
Figure 1 (a) Total nitrogen (TN) versus δ15Nbulk. (b) TN versus total inorganic carbon (TIC). (c) TN versus total organic carbon (TOC). The hydrogenetic crusts are relatively depleted in δ15Nbulk and TN but enriched in TIC.
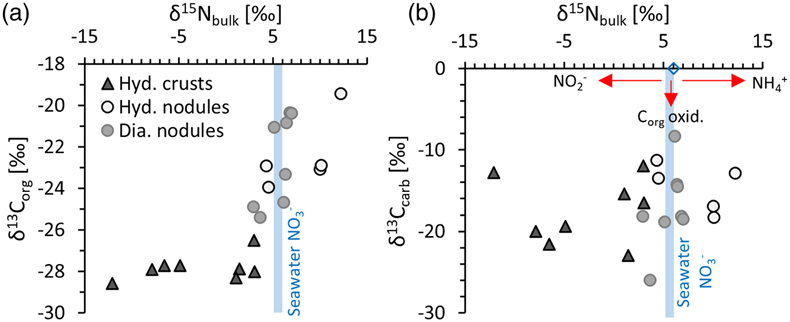
Figure 2 (a) δ13Corg versus δ15Nbulk. (b) δ13Ccarb versus δ15Nbulk. The hydrogenetic crusts tend to be depleted in δ15Nbulk relative to average seawater (blue line), possibly reflecting intake of isotopically light NO2− generated within sediments from NH4+ oxidation. Slight enrichment in δ15Nbulk in diagenetic oxides may reflect retention of isotopically heavy NH4+ after partial oxidation.
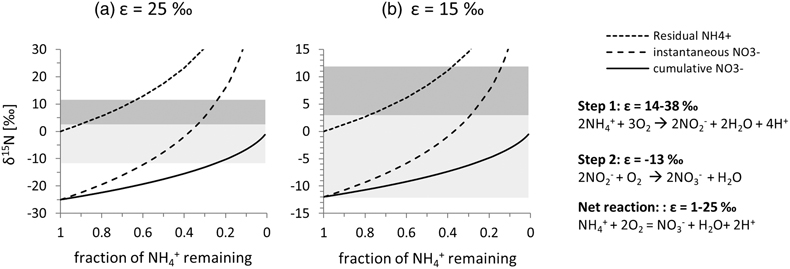
Figure 3 Proposed mechanism of partial NH4+ oxidation to NO3− via NO2− and associated Rayleigh fractionation. (a) Fractionation (ɛ = δ15Nreactant – δ15Nproduct) of 25 ‰, maximum net fractionation for NH4+ → NO3−. (b) Fractionation of 15 ‰, smallest possible fractionation to explain the data. Reactions on the right show that partial oxidation could also generate light NO2−. Light grey shading = range of δ15N values seen in hydrogenetic crusts; dark grey shading = range of δ15N values seen in diagenetic and hydrogenetic nodules.
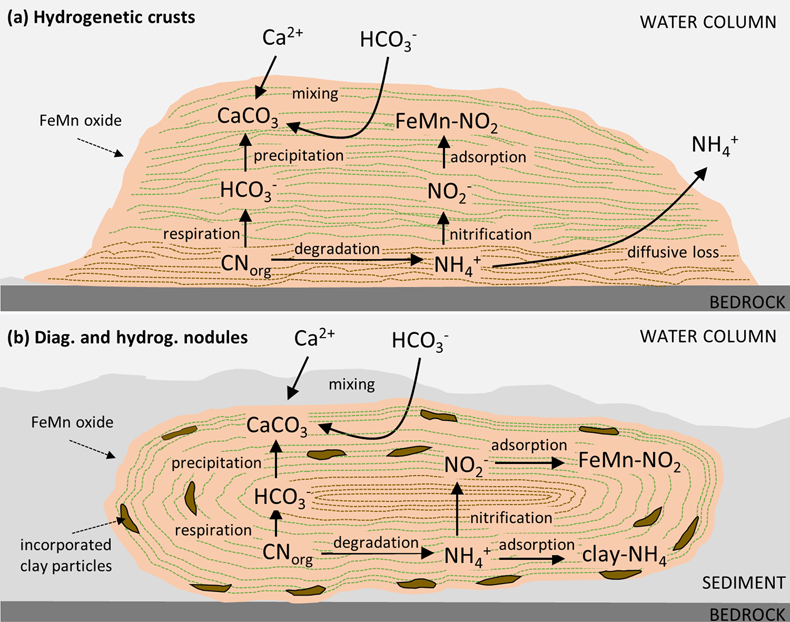
Figure 4 Environmental sketch. Dead biomass (dotted brown lines) releases ammonium during degradation, which is partially oxidised to nitrite. Some of this nitrite is trapped in FeMn oxides by adsorption and possibly by assimilation into fresh biomass (dotted pale green lines). (a) In hydrogenetic crusts, the residual 15N-enriched ammonium is lost to the water column by diffusion. (b) In diagenetic and hydrogenetic nodules, some of the ammonium is retained in clay minerals, leading to higher bulk δ15N values and TN abundances.