The isotopic signature of UV during bacterial reduction
Affiliations | Corresponding Author | Cite as | Funding information- Share this article
- Article views:162
Cumulative count of HTML views and PDF downloads.
- Download Citation
- Rights & Permissions
Abstract
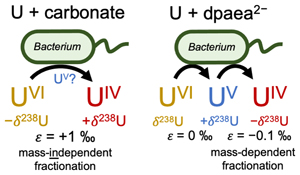
Figures
![]() Figure 1 (a) Uranium mass distribution in sacrificial reactors containing S. oneidensis incubated with UVIO2-dpaea. (b) Normalised U M4-edge HR-XANES spectrum of aqueous uranium after 144 hr of incubation with S. oneidensis, along with UVIO2-dpaea, UVO2-dpaea– and UIV-(dpaea)2 standards. | ![]() Figure 2 (a) Aqueous uranium concentrations throughout the first 24 hr of incubation of UVIO2-dpaea and S. oneidensis. Symbols and error bars depict one standard deviation of the mean of duplicate reactors. (b) Corresponding δ238U values of the aqueous U in duplicate systems (A and B), reported as a fraction of the maximum aqueous U concentration. Symbols and error bars depict two standard deviations of the mean of triplicate measurements. The δ238U value of the initial UVIO2-dpaea is plotted as a yellow dotted line. (c) Aqueous uranium concentrations throughout the whole reaction between UVIO2-dpaea and S. oneidensis. Symbols and error bars depict one standard deviation of the mean of duplicate reactors. (d) δ238U values of the aqueous U after 24 hr when the aqueous U concentration began to decrease. Values are reported as a fraction of the maximum aqueous U concentration. Symbols and error bars depict two standard deviations of the mean of triplicate measurements. The Rayleigh model (blue dashed line) corresponds to the linear best fit of the logarithmic data, R2 = 0.89, from which the isotope enrichment factor, ɛ, is derived. | ![]() Figure 3 (a) Aqueous uranium concentrations during equilibrium isotope exchange experiments between UVO2-dpaea– with an initial isotopic composition of ∼5 ‰, and UIV present as the product of the bioreduction experiments of natural U, with an initial isotopic composition of 0 ‰. Symbols and error bars depict one standard deviation of the mean of duplicate reactors. (b) δ238U values of the aqueous U. Symbols and error bars depict one standard deviation of the mean of duplicate reactors. | ![]() Figure 4 Cartoon of the proposed mechanism of U isotope reduction and fractionation for both UVI-carbonate (left) and UV-dpaea– (right). Electrons are transferred from the cell to outer membrane U reducing proteins (blue areas) containing multiple redox active heme iron centres (red circles). Depending on the flux of electrons, the heme iron centres are either in their reduced state (solid fill) or oxidised state (open fill). |
Figure 1 | Figure 2 | Figure 3 | Figure 4 |
Introduction
Hexavalent uranium (UVI) is the predominant oxidation state of U under ambient oxic conditions at Earth’s surface and forms soluble uranyl complexes. Under anoxic conditions, reduction of UVI to tetravalent U (UIV) can be mediated by an array of microorganisms or abiotically via Fe(II)- or sulfide-bearing compounds (Basu et al., 2014Basu, A., Sanford, R.A., Johnson, T.M., Lundstrom, C.C., Löffler, F.E. (2014) Uranium isotopic fractionation factors during U(VI) reduction by bacterial isolates. Geochimica et Cosmochimica Acta 136, 100–113. https://doi.org/10.1016/j.gca.2014.02.041
; Brown et al., 2018Brown, S.T., Basu, A., Ding, X., Christensen, J.N., DePaolo, D.J. (2018) Uranium isotope fractionation by abiotic reductive precipitation. Proceedings of the National Academy of Sciences of the United States of America 115, 8688–8693. https://doi.org/10.1073/pnas.1805234115
), resulting in the precipitation of sparingly soluble UIV species. This behaviour has been harnessed for the (bio)remediation of U contaminated groundwater.Such U redox transformations are often accompanied by changes in the 238U/235U ratio, reported as δ238U (Andersen et al., 2017Andersen, M.B., Stirling, C.H., Weyer, S. (2017) Uranium Isotope Fractionation. Reviews in Mineralogy and Geochemistry 82, 799–850. https://doi.org/10.2138/rmg.2017.82.19
). Both ab initio calculations and isotope exchange experiments indicate that, at equilibrium, heavy 238U is enriched in the UIV oxidation state (Schauble, 2007Schauble, E.A. (2007) Role of nuclear volume in driving equilibrium stable isotope fractionation of mercury, thallium, and other very heavy elements. Geochimica et Cosmochimica Acta 71, 2170–2189. https://doi.org/10.1016/j.gca.2007.02.004
; Abe et al., 2008Abe, M., Suzuki, T., Fujii, Y., Hada, M., Hirao, K. (2008) An ab initio molecular orbital study of the nuclear volume effects in uranium isotope fractionations. The Journal of Chemical Physics 129, 164309. https://doi.org/10.1063/1.2992616
; Wang et al., 2015Wang, X., Johnson, T.M., Lundstrom, C.C. (2015) Low temperature equilibrium isotope fractionation and isotope exchange kinetics between U(IV) and U(VI). Geochimica et Cosmochimica Acta 158, 262–275. https://doi.org/10.1016/j.gca.2015.03.006
). This mass-independent fractionation arises from the nuclear field shift effect (NFSE), due to differences in the size and shape of the nuclei of heavy element isotopologues (Bigeleisen, 1996Bigeleisen, J. (1996) Nuclear size and shape effects in chemical reactions. Isotope chemistry of the heavy elements. Journal of the American Chemical Society 118, 3676–3680. https://doi.org/10.1021/ja954076k
; Schauble, 2007Schauble, E.A. (2007) Role of nuclear volume in driving equilibrium stable isotope fractionation of mercury, thallium, and other very heavy elements. Geochimica et Cosmochimica Acta 71, 2170–2189. https://doi.org/10.1016/j.gca.2007.02.004
). At equilibrium, the NFSE is larger than, and operates in the opposite direction to, the conventional mass-dependent isotope effect, whereby the vibrational zero point energy of the lighter isotope leads to its enrichment in UIV as mass-dependent fractionation (MDF) (Bigeleisen, 1996Bigeleisen, J. (1996) Nuclear size and shape effects in chemical reactions. Isotope chemistry of the heavy elements. Journal of the American Chemical Society 118, 3676–3680. https://doi.org/10.1021/ja954076k
; Schauble, 2007Schauble, E.A. (2007) Role of nuclear volume in driving equilibrium stable isotope fractionation of mercury, thallium, and other very heavy elements. Geochimica et Cosmochimica Acta 71, 2170–2189. https://doi.org/10.1016/j.gca.2007.02.004
; Fujii et al., 2009Fujii, T., Moynier, F., Albarède, F. (2009) The nuclear field shift effect in chemical exchange reactions. Chemical Geology 267, 139–156. https://doi.org/10.1016/j.chemgeo.2009.06.015
). Thus, enrichment of 238U in UIV following UVI reduction has also been attributed to a dominant NFSE (Weyer et al., 2008Weyer, S., Anbar, A.D., Gerdes, A., Gordon, G.W., Algeo, T.J., Boyle, E.A. (2008) Natural fractionation of 238U/235U. Geochimica et Cosmochimica Acta 72, 345–359. https://doi.org/10.1016/j.gca.2007.11.012
; Basu et al., 2014Basu, A., Sanford, R.A., Johnson, T.M., Lundstrom, C.C., Löffler, F.E. (2014) Uranium isotopic fractionation factors during U(VI) reduction by bacterial isolates. Geochimica et Cosmochimica Acta 136, 100–113. https://doi.org/10.1016/j.gca.2014.02.041
, 2020Basu, A., Wanner, C., Johnson, T.M., Lundstrom, C., Sanford, R.A., Sonnenthal, E., Boyanov, M.I., Kemner, K.M. (2020) Microbial U isotope fractionation depends on U(VI) reduction rate. Environmental Science and Technology 54, 2295–2303. https://doi.org/10.1021/acs.est.9b05935
; Stirling et al., 2015Stirling, C.H., Andersen, M.B., Warthmann, R., Halliday, A.N. (2015) Isotope fractionation of 238U and 235U during biologically-mediated uranium reduction. Geochimica et Cosmochimica Acta 163, 200–218. https://doi.org/10.1016/j.gca.2015.03.017
; Stylo et al., 2015Stylo, M., Neubert, N., Wang, Y., Monga, N., Romaniello, S.J., Weyer, S., Bernier-Latmani, R. (2015) Uranium isotopes fingerprint biotic reduction. Proceedings of the National Academy of Sciences of the United States of America 112, 5619–24. https://doi.org/10.1073/pnas.1421841112
), despite not necessarily representing isotopic equilibrium conditions.As U isotope fractionation is predominantly associated with redox transformations, U isotope signatures have been utilised as a (1) monitoring tool tuned specifically to the reductive rather than adsorptive removal of UVI during remediation (Bopp et al., 2010Bopp, C.J., Lundstrom, C.C., Johnson, T.M., Sandford, R.A., Long, P.E., Williams, K.H. (2010) Uranium 238U/235U isotope ratios as indicators of reduction: Results from an in situ biostimulation experiment at Rifle, Colorado, U.S.A. Environmental Science and Technology 44, 5927–5933. https://doi.org/10.1021/es100643v
), and (2) palaeo-redox proxy, whereby the preferential reduction of 238U during marine anoxia is recorded in sedimentary rocks and can be used to reconstruct the pervasiveness of anoxia in past global oceans (Montoya-Pino et al., 2010Montoya-Pino, C., Anbar, A.D., van de Schootbrugge, B., Oschmann, W., Pross, J., Arz, H.W., Weyer, S. (2010) Global enhancement of ocean anoxia during Oceanic Anoxic Event 2: A quantitative approach using U isotopes. Geology 38, 315–318. https://doi.org/10.1130/G30652.1
; Brennecka et al., 2011Brennecka, G.A., Herrmann, A.D., Algeo, T.J., Anbar, A.D. (2011) Rapid expansion of oceanic anoxia immediately before the end-Permian mass extinction. Proceedings of the National Academy of Sciences of the United States of America 108, 17631–17634. https://doi.org/10.1073/pnas.1106039108
; Andersen et al., 2017Andersen, M.B., Stirling, C.H., Weyer, S. (2017) Uranium Isotope Fractionation. Reviews in Mineralogy and Geochemistry 82, 799–850. https://doi.org/10.2138/rmg.2017.82.19
). Hence, it is crucial to constrain the mechanistic underpinnings of U isotope fractionation to improve the reliability of U isotope based redox reconstructions.One important aspect of the U reduction mechanism is the role of the pentavalent U (UV) intermediate. Previous studies have focused on the complete reduction of UVI to UIV. However, there is increasing evidence of the stabilisation and persistence of UV intermediates within abiotic and biological systems (Roberts et al., 2017Roberts, H.E., Morris, K., Law, G.T.W., Mosselmans, J.F.W., Bots, P., Kvashnina, K., Shaw, S. (2017) Uranium(V) incorporation mechanisms and stability in Fe(II)/Fe(III) (oxyhydr)oxides. Environmental Science and Technology Letters 4, 421–426. https://doi.org/10.1021/acs.estlett.7b00348
; Pan et al., 2020Pan, Z., Bártová, B., Lagrange, T., Butorin, S.M., Hyatt, N.C., Stennett, M.C., Kvashnina, K.O., Bernier-latmani, R. (2020) Nanoscale mechanism of UO2 formation through uranium reduction by magnetite. Nature Communications 11, 1–12. https://doi.org/10.1038/s41467-020-17795-0
).During microbiological UVI reduction, two distinct mechanisms for the complete reduction to UIV can occur: either via disproportionation of two uranylV atoms (generating UVI and UIV) (Vettese et al., 2020Vettese, G.F., Morris, K., Natrajan, L.S., Shaw, S., Vitova, T., Galanzew, J., Jones, D.L. and Lloyd, J.R. (2020) Multiple lines of evidence identify U(V) as a key intermediate during U(VI) reduction by Shewanella oneidensis MR1. Environmental Science and Technology 54, 2268–2276. https://doi.org/10.1021/acs.est.9b05285
), or via a second biologically mediated electron transfer to UV (Molinas et al., 2021Molinas, M., Faizova, R., Brown, A., Galanzew, J., Schacherl, B., Bartova, B., Meibom, K.L., Vitova, T., Mazzanti, M. and Bernier-Latmani, R. (2021) Biological reduction of a U(V)-organic ligand complex. Environmental Science and Technology 55, 4753–4761. https://doi.org/10.1021/acs.est.0c06633
, 2023Molinas, M., Meibom, K.L., Faizova, R., Mazzanti, M., Bernier-Latmani, R. (2023) Mechanism of reduction of aqueous U(V)-dpaea and solid-phase U(VI)-dpaea complexes: The role of multiheme c-type cytochromes. Environmental Science and Technology 57, 7537–7546. https://doi.org/10.1021/acs.est.3c00666
). However, due to the challenges associated with the chemical stabilisation and separation of UV, there is a lack of experimental evidence for its isotopic fractionation, and thus its role in the fractionation mechanism remains unresolved.Ab initio calculations of the equilibrium isotope fractionation factor combined with a multi-step model of biological UVI-carbonate reduction suggests that fractionation factors of up to 1.6 ‰ for the UVI to UV step and ∼0.8 ‰ for the UV to UIV step (a total of ∼2.4 ‰) may be expected (Sato et al., 2021Sato, A., Bernier-Latmani, R., Hada, M., Abe, M. (2021) Ab initio and steady-state models for uranium isotope fractionation in multi-step biotic and abiotic reduction. Geochimica et Cosmochimica Acta 307, 212–227. https://doi.org/10.1016/j.gca.2021.05.044
). However, these values are significantly larger than those observed in nature or experimentally for UVI to UIV reduction, and it is not clear whether and how redox transformations to and from the UV intermediate are involved in this discrepancy.The aminocarboxylate ligand dpaea2− (dpaeaH2 = bis(pyridyl-6-methyl-2-carboxylate)-ethylamine) can be used to precipitate both UVI and UIV whilst maintaining UV as an aqueous complex at circumneutral pH (Faizova et al., 2018Faizova, R., Scopelliti, R., Chauvin, A.-S., Mazzanti, M. (2018) Synthesis and characterization of a water stable uranyl(V) complex. Journal of the American Chemical Society 140, 13554–13557. https://doi.org/10.1021/jacs.8b07885
). These properties have allowed the reduction of UVI by Shewanella oneidensis to be followed, revealing the potential for the biological reduction of the UV intermediate, rather than its disproportionation (Molinas et al., 2021Molinas, M., Faizova, R., Brown, A., Galanzew, J., Schacherl, B., Bartova, B., Meibom, K.L., Vitova, T., Mazzanti, M. and Bernier-Latmani, R. (2021) Biological reduction of a U(V)-organic ligand complex. Environmental Science and Technology 55, 4753–4761. https://doi.org/10.1021/acs.est.0c06633
, 2023Molinas, M., Meibom, K.L., Faizova, R., Mazzanti, M., Bernier-Latmani, R. (2023) Mechanism of reduction of aqueous U(V)-dpaea and solid-phase U(VI)-dpaea complexes: The role of multiheme c-type cytochromes. Environmental Science and Technology 57, 7537–7546. https://doi.org/10.1021/acs.est.3c00666
).Here, we leveraged the characteristics of dpaea2− to trap aqueous UV and provide direct experimental evidence of the UV isotope signature during biological reduction by S. oneidensis. The observed isotopic fractionation factors were then compared to those predicted for equilibrium both computationally, using ab initio calculations, and experimentally, using isotope exchange approaches (see Supplementary Information for details). topResults and Discussion
The overall experimental flow entails the biological reduction of UVI-dpaea to first UV-dpaea and then of UV-dpaea to UIV-dpaea. The temporal separation of the two steps, made possible by the vastly different reduction rates, allows the investigation of the isotopic fractionation of one step and then the other. Additionally, the equilibrium isotope fractionation factor was calculated via ab initio calculations. Finally, to investigate the equilibrium isotopic fractionation of UV-dpaea and UIV-dpaea, a heavy UV-dpaea was incubated with a light UIV-dpaea and the isotopic exchange probed over time.First, UVI-dpaea was produced and reduced biologically. We incubated S. oneidensis with solid phase UVIO2-dpaea and observed a rapid decrease in UVI over 24 hr. This was concomitant with an increase in aqueous U (Fig. 1a) comprising predominantly UV (Fig. 1b) that was not observed in abiotic controls (Fig. S-1). Acidification of the aqueous U in 4.5 N HCl, in preparation for ion exchange chromatography, led to the detection of approximately equal quantities of UVI and UIV after separation (Fig. S-2), indicative of UV disproportionation in the acidified preparation. Collectively, these data suggest that the first electron transfer was achieved rapidly, leading to the accumulation of UV in solution, in agreement with previous studies (Molinas et al., 2021Molinas, M., Faizova, R., Brown, A., Galanzew, J., Schacherl, B., Bartova, B., Meibom, K.L., Vitova, T., Mazzanti, M. and Bernier-Latmani, R. (2021) Biological reduction of a U(V)-organic ligand complex. Environmental Science and Technology 55, 4753–4761. https://doi.org/10.1021/acs.est.0c06633
, 2023Molinas, M., Meibom, K.L., Faizova, R., Mazzanti, M., Bernier-Latmani, R. (2023) Mechanism of reduction of aqueous U(V)-dpaea and solid-phase U(VI)-dpaea complexes: The role of multiheme c-type cytochromes. Environmental Science and Technology 57, 7537–7546. https://doi.org/10.1021/acs.est.3c00666
).
Molinas, M., Faizova, R., Brown, A., Galanzew, J., Schacherl, B., Bartova, B., Meibom, K.L., Vitova, T., Mazzanti, M. and Bernier-Latmani, R. (2021) Biological reduction of a U(V)-organic ligand complex. Environmental Science and Technology 55, 4753–4761. https://doi.org/10.1021/acs.est.0c06633
, 2023Molinas, M., Meibom, K.L., Faizova, R., Mazzanti, M., Bernier-Latmani, R. (2023) Mechanism of reduction of aqueous U(V)-dpaea and solid-phase U(VI)-dpaea complexes: The role of multiheme c-type cytochromes. Environmental Science and Technology 57, 7537–7546. https://doi.org/10.1021/acs.est.3c00666
). It is likely that reduction of UVIO2-dpaea proceeds via dissolution of the solid uranylVI followed by rapid reduction of aqueous uranylVI, i.e. dissolution is the rate limiting step for the first electron transfer (Molinas et al., 2023Molinas, M., Meibom, K.L., Faizova, R., Mazzanti, M., Bernier-Latmani, R. (2023) Mechanism of reduction of aqueous U(V)-dpaea and solid-phase U(VI)-dpaea complexes: The role of multiheme c-type cytochromes. Environmental Science and Technology 57, 7537–7546. https://doi.org/10.1021/acs.est.3c00666
).A slow second electron transfer step (UV/UIV) is consistent with abiotic reduction by sodium hydrosulfite (Faizova et al., 2020Faizova, R., Fadaei‐Tirani, F., Bernier‐Latmani, R., Mazzanti, M. (2020) Ligand‐supported facile conversion of uranyl(VI) into uranium(IV) in organic and aqueous media. Angewandte Chemie 132, 6822–6825. https://doi.org/10.1002/ange.201916334
). Cyclic voltammograms of a UVO2-dpaea complex at pH 7 did not display a UV/UIV reduction event, suggesting slow electron transfer kinetics that may be related to required structural re-arrangements for the formation of a tri-nuclear UIV product (Faizova et al., 2018Faizova, R., Scopelliti, R., Chauvin, A.-S., Mazzanti, M. (2018) Synthesis and characterization of a water stable uranyl(V) complex. Journal of the American Chemical Society 140, 13554–13557. https://doi.org/10.1021/jacs.8b07885
, 2020Faizova, R., Fadaei‐Tirani, F., Bernier‐Latmani, R., Mazzanti, M. (2020) Ligand‐supported facile conversion of uranyl(VI) into uranium(IV) in organic and aqueous media. Angewandte Chemie 132, 6822–6825. https://doi.org/10.1002/ange.201916334
).Uranium isotopic fractionation during the first electron transfer from UVI to UV was investigated with a dedicated incubation of UVIO2-dpaea (Fig. 2a). Here, the increasing aqueous U showed negligible changes in δ238U, indicating that the UVI/UV reduction displayed little fractionation (Fig. 2b). Reduction of UVI by a range of bacterial species typically display enrichment of the heavier 238U in the reduced product, consistent with the predictions of NFS theory during equilibrium isotope fractionation (Basu et al., 2014Basu, A., Sanford, R.A., Johnson, T.M., Lundstrom, C.C., Löffler, F.E. (2014) Uranium isotopic fractionation factors during U(VI) reduction by bacterial isolates. Geochimica et Cosmochimica Acta 136, 100–113. https://doi.org/10.1016/j.gca.2014.02.041
). Indeed, ab initio calculation of the expected isotope fractionation factor between the UVIO2-dpaea and UVO2-dpaea- at equilibrium gave a value of 0.82–1.60 ‰ (Table S-1), wherein the positive value signals preferential reduction of 238U. Rather, the isotope signatures of the UVO2-dpaea- observed in the experiment appear consistent with dissolution being the rate limiting step for the first electron transfer, such that U isotope reduction is rapid and quantitative. As dissolution does not involve a redox reaction, the mass-independent isotope fractionation predicted by the NFSE would not be expected.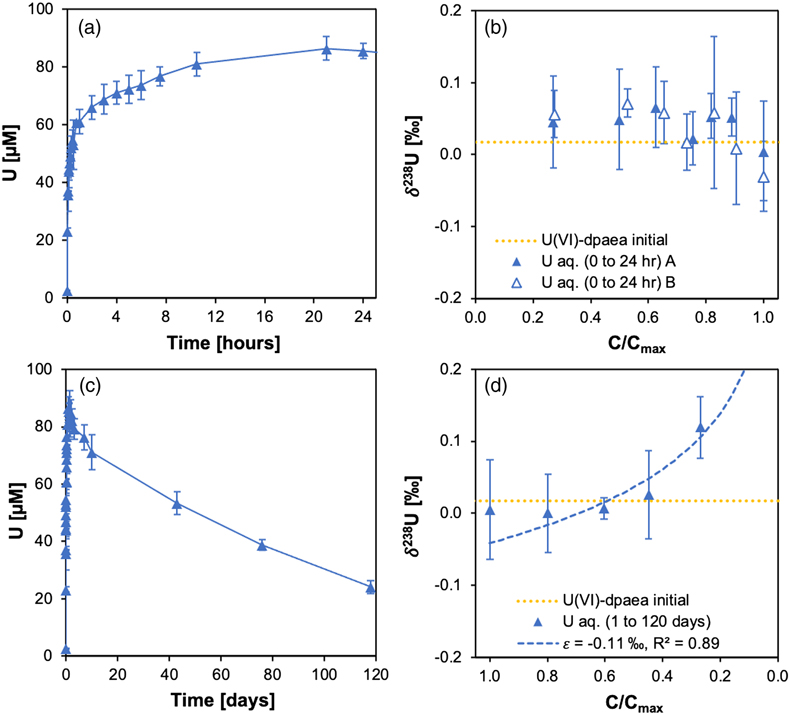
Basu, A., Sanford, R.A., Johnson, T.M., Lundstrom, C.C., Löffler, F.E. (2014) Uranium isotopic fractionation factors during U(VI) reduction by bacterial isolates. Geochimica et Cosmochimica Acta 136, 100–113. https://doi.org/10.1016/j.gca.2014.02.041
; Stirling et al., 2015Stirling, C.H., Andersen, M.B., Warthmann, R., Halliday, A.N. (2015) Isotope fractionation of 238U and 235U during biologically-mediated uranium reduction. Geochimica et Cosmochimica Acta 163, 200–218. https://doi.org/10.1016/j.gca.2015.03.017
; Stylo et al., 2015Stylo, M., Neubert, N., Wang, Y., Monga, N., Romaniello, S.J., Weyer, S., Bernier-Latmani, R. (2015) Uranium isotopes fingerprint biotic reduction. Proceedings of the National Academy of Sciences of the United States of America 112, 5619–24. https://doi.org/10.1073/pnas.1421841112
). To ascertain whether this direction of fractionation reflected equilibrium in the peculiar case of a strong aminocarboxylate ligand, we performed ab initio calculations of the fractionation factor at equilibrium between UVO2-dpaea− and either UIV-(dpaea)2 or a non-uraninite UIV species, the two likely products of this biological reaction (Molinas et al., 2021Molinas, M., Faizova, R., Brown, A., Galanzew, J., Schacherl, B., Bartova, B., Meibom, K.L., Vitova, T., Mazzanti, M. and Bernier-Latmani, R. (2021) Biological reduction of a U(V)-organic ligand complex. Environmental Science and Technology 55, 4753–4761. https://doi.org/10.1021/acs.est.0c06633
). We modelled the non-uraninite UIV as a cluster of ningyoite (CaU(PO4)2), a close analogue of the non-crystalline biotic reduction products (Bernier-Latmani et al., 2010Bernier-Latmani, R., Veeramani, H., Vecchia, E.D., Junier, P., Lezama-Pacheco, J.S., Suvorova, E.I., Sharp, J.O., Wigginton, N.S., Bargar, J.R. (2010) Non-uraninite products of microbial U (VI) reduction. Environmental Science and Technology 44, 9456–9462. https://doi.org/10.1021/es101675a
; Alessi et al., 2014Alessi, D.S., Lezama-Pacheco, J.S., Stubbs, J.E., Janousch, M., Bargar, J.R., Persson, P., Bernier-Latmani, R. (2014) The product of microbial uranium reduction includes multiple species with U(IV)-phosphate coordination. Geochimica et Cosmochimica Acta 131, 115–127. https://doi.org/10.1016/j.gca.2014.01.005
). The fractionation factors of 0.27–0.33 ‰ for the UIV-(dpaea)2 product and 0.13–0.46 ‰ for ningyoite both reveal that 238U would be enriched in the UIV product at equilibrium (Table S-1), contrary to that observed during biological reduction. These calculations indicate that the bioreduction system was far from equilibrium and suggest that the reaction mechanism precluded the full expression of NFSE that would have enriched 238U in the product. Furthermore, recent work has proposed that slow microbial reduction should impart significant mass-independent fractionation of up to +1 ‰ (Brown et al., 2018Brown, S.T., Basu, A., Ding, X., Christensen, J.N., DePaolo, D.J. (2018) Uranium isotope fractionation by abiotic reductive precipitation. Proceedings of the National Academy of Sciences of the United States of America 115, 8688–8693. https://doi.org/10.1073/pnas.1805234115
; Basu et al., 2020Basu, A., Wanner, C., Johnson, T.M., Lundstrom, C., Sanford, R.A., Sonnenthal, E., Boyanov, M.I., Kemner, K.M. (2020) Microbial U isotope fractionation depends on U(VI) reduction rate. Environmental Science and Technology 54, 2295–2303. https://doi.org/10.1021/acs.est.9b05935
), whereas negative fractionation factors are typically only observed for rapid abiotic reductions, on the order of hours (Stylo et al., 2015Stylo, M., Neubert, N., Wang, Y., Monga, N., Romaniello, S.J., Weyer, S., Bernier-Latmani, R. (2015) Uranium isotopes fingerprint biotic reduction. Proceedings of the National Academy of Sciences of the United States of America 112, 5619–24. https://doi.org/10.1073/pnas.1421841112
). The slow reduction of the UVO2-dpaea- observed in our experiments (on the order of months), suggests that the proposed reduction rate-fractionation relationship does not hold for all circumstances.To investigate whether equilibrium isotope exchange and the associated expression of the NFSE could overprint the reduction-derived MDF signature, we performed isotope exchange experiments between the solid UIV product of the bioreduction experiment, with an initial (light) δ238U of 0 ‰, and aqueous UVO2-dpaea-, with an initial (heavy) δ238U of ∼5 ‰. Over 200 days, aqueous U became isotopically lighter by 0.6 ‰, indicating the preferential accumulation of 238U in the UIV solid (Fig. 3). Whilst this direction of fractionation is in agreement with that calculated for equilibrium, isotope mass balance calculations indicate that the UIV solid did not become heavier than the aqueous UV, contrary to the computed equilibrium. These data show that progress to full equilibrium is significantly limited over the course of the experiment, presumably due to slow ligand exchange kinetics.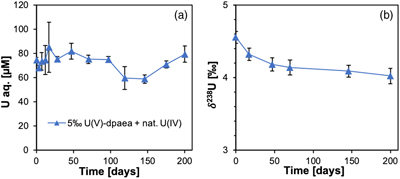
Faizova, R., Scopelliti, R., Chauvin, A.-S., Mazzanti, M. (2018) Synthesis and characterization of a water stable uranyl(V) complex. Journal of the American Chemical Society 140, 13554–13557. https://doi.org/10.1021/jacs.8b07885
). Furthermore, any preferential re-oxidation of 235UIV to UV would require the de novo formation of the two uranyl dioxo bonds and re-coordination with dpaea. This is likely kinetically limited due to steric hinderance by the UIV coordinating ligands. Therefore, we propose that isotope signatures indicating mass-dependent fractionation (faster reaction of 235U) are preserved during the biological reduction of UVO2-dpaea- because subsequent equilibrium isotope exchange, fractionating in the opposite direction, is limited.Regardless of the abiotic equilibrium isotope exchange between reactants and products (independent of the bioreduction reaction), a recent model has demonstrated the importance of back reaction within the UVI bioreduction pathway in controlling the overall isotope fractionation (Sato et al., 2021Sato, A., Bernier-Latmani, R., Hada, M., Abe, M. (2021) Ab initio and steady-state models for uranium isotope fractionation in multi-step biotic and abiotic reduction. Geochimica et Cosmochimica Acta 307, 212–227. https://doi.org/10.1016/j.gca.2021.05.044
). The model stipulates that the overall isotope fractionation at each reaction step arises from the balance between the forward and backward reaction rates, and the attendant isotope fractionation for the forward and backward reactions. As such, reactions with equal forward and backward reaction rates will display the full fractionation factor predicted for equilibrium (typically positive for U reduction, indicating preferential accumulation of 238U in the product). On the other hand, irreversible reactions will result in no observed fractionation. The theory of this model has been demonstrated experimentally during UVI reduction by S. oneidensis, in which back reaction (reverse electron transfer) was limited by large electron fluxes from oxidation of the electron donor (Brown et al., 2023aBrown, A.R., Molinas, M., Roebbert, Y., Sato, A., Abe, M., Weyer, S., Bernier-Latmani, R. (2023a) Electron flux is a key determinant of uranium isotope fractionation during bacterial reduction. Communications Earth and Environment 4, 329. https://doi.org/10.1038/s43247-023-00989-x
). These systems result in significantly less isotope fractionation than those with small electron fluxes, which permit more back reaction. The theoretical model and associated experimental evidence, coupled to our observations of the isotope exchange experiment, would suggest that back reaction during biological reduction of UVO2-dpaea- is limited and point toward the role of the U coordinating ligand in controlling the magnitude of isotope fractionation.Furthermore, during microbiological reduction of UVI-carbonate, the conventional isotopic mass effect was fully expressed, while the NFSE was not (Brown et al., 2023bBrown, A.R., Roebbert, Y., Sato, A., Hada, M., Abe, M., Weyer, S., Bernier-Latmani, R. (2023b) Contribution of the nuclear field shift to kinetic uranium isotope fractionation. Geochemical Perspectives Letters 27, 43–47. https://doi.org/10.7185/geochemlet.2333
). This implies that the mass-dependent vibrational effect and the mass-independent NFSE are two competing effects operating in opposing directions and is consistent with the proposal that the NFSE requires reaction reversibility in order to overprint the mass-dependent effect.Collectively, these studies indicate that the inhibition of back reaction in the dpaea system is so severe that the mass-dependent isotope fractionation factor is preserved. More specifically, we propose the following mechanism: first, the flux of electrons from the cell to the outer membrane U-reducing proteins is significantly greater than the UV reduction rates (limited by either low redox potential and/or steric hinderance) (Fig. 4). This allows the redox-active Fe-bearing heme groups of these proteins to become fully reduced prior to electron transfer to UV. Eventually, electron transfer from the heme FeII to UV occurs with isotopic fractionation according to the conventional mass effect – faster reaction of 235U. Concurrently, a rapid continuous flux of electrons from metabolism re-reduces the FeIII of the heme group (empty circles in Fig. 4) and prevents reverse electron transfer from the newly reduced UIV. Consequently, isotopic equilibration that is dominated by the mass-independent NFSE cannot over-print the initial MDF, unlike in U-carbonate containing systems.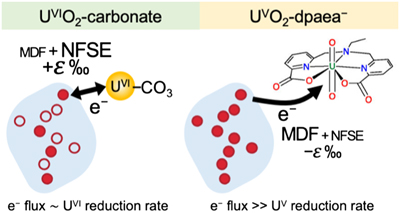
Conclusions
We employed the UV stabilising ligand, dpaea, to trap aqueous UV and observed, for the first time, the isotopic signature of UV throughout the bioreduction of UVI to UIV. Whilst the observation of a mass-dependent isotope fractionation factor appears to conflict with previous studies of microbial U reduction, this is likely not an artefact of the unique properties of dpaea (i.e. its ability to solubilise and trap UV). Rather, these adventitious properties have elucidated the control U coordinating ligands exert over the balance between reactant U supply, electron transfer rate, and UIV product sequestration. Thus, we infer that other ligands (that cannot stabilise UV) will behave similarly when such conditions are met. This has significant implications for the interpretation of U isotope signatures in environments where the availability of high affinity ligands may impact U lability. For example, in reducing environments with considerable amounts of organic carbon (providing both a source of electrons for microbial UVI reduction and a supply of organic complexants), the contribution of the NFSE to observed isotopic signatures may be diminished. This may lead to false interpretations of U isotope signatures, e.g., in applications using organic-rich anoxic sediments as a palaeo-redox archive. In such studies, the observation of lower δ238U (arising from NFSE-dominated mass-independent fractionation) is usually thought to indicate either a local shift in depositional conditions or water column stratification (Andersen et al., 2017Andersen, M.B., Stirling, C.H., Weyer, S. (2017) Uranium Isotope Fractionation. Reviews in Mineralogy and Geochemistry 82, 799–850. https://doi.org/10.2138/rmg.2017.82.19
; Brüske et al., 2020Brüske, A., Weyer, S., Zhao, M.Y., Planavsky, N.J., Wegwerth, A., Neubert, N., Dellwig, O., Lau, K.V., Lyons, T.W. (2020) Correlated molybdenum and uranium isotope signatures in modern anoxic sediments: Implications for their use as paleo-redox proxy. Geochimica et Cosmochimica Acta 270, 449–474. https://doi.org/10.1016/j.gca.2019.11.031
; Lau et al., 2022Lau, K.V, Hancock, L.G., Severmann, S., Kuzminov, A., Cole, D.B., Behl, R.J., Planavsky, N.J., Lyons, T.W. (2022) Variable local basin hydrography and productivity control the uranium isotope paleoredox proxy in anoxic black shales. Geochimica et Cosmochimica Acta. 317, 433–456. https://doi.org/10.1016/j.gca.2021.10.011
), or a shift in the U isotope mass balance, resulting from enhanced oceanic anoxic environments at regional or global scales (Montoya-Pino et al., 2010Montoya-Pino, C., Anbar, A.D., van de Schootbrugge, B., Oschmann, W., Pross, J., Arz, H.W., Weyer, S. (2010) Global enhancement of ocean anoxia during Oceanic Anoxic Event 2: A quantitative approach using U isotopes. Geology 38, 315–318. https://doi.org/10.1130/G30652.1
; Andersen et al., 2017Andersen, M.B., Stirling, C.H., Weyer, S. (2017) Uranium Isotope Fractionation. Reviews in Mineralogy and Geochemistry 82, 799–850. https://doi.org/10.2138/rmg.2017.82.19
). However, our results show that the extent and direction of U isotope fractionation during U reduction may depend on the stabilisation of UV and, more generally, the lability of U complexes.Furthermore, this study suggests that full expression of isotopic equilibrium in nature may be precluded by U speciation, in addition to the previous roles reported for electron flux and U supply dynamics (Basu et al., 2020Basu, A., Wanner, C., Johnson, T.M., Lundstrom, C., Sanford, R.A., Sonnenthal, E., Boyanov, M.I., Kemner, K.M. (2020) Microbial U isotope fractionation depends on U(VI) reduction rate. Environmental Science and Technology 54, 2295–2303. https://doi.org/10.1021/acs.est.9b05935
; Brown et al., 2023aBrown, A.R., Molinas, M., Roebbert, Y., Sato, A., Abe, M., Weyer, S., Bernier-Latmani, R. (2023a) Electron flux is a key determinant of uranium isotope fractionation during bacterial reduction. Communications Earth and Environment 4, 329. https://doi.org/10.1038/s43247-023-00989-x
). Future work should focus on delineating these controls with an aim to incorporate U speciation as a parameter within models of U isotope fractionation in the environment. topAcknowledgements
Funding for this work was provided by an ERC consolidator grant awarded to RB-L (725675: UNEARTH: “Uranium isotope fractionation: a novel biosignature to identify microbial metabolism on early Earth”). This work was also supported by JSPS KAKENHI Grant Numbers JP19K22171, JP21H01864 and JP22J12551. A part of the calculations was performed at the Research Center for Computational Science, Okazaki, Japan (Project: 21-IMS-C049 and 22-IMS-C049). Pierre Rossi provided invaluable support in complying with radiological safety regulations in the lab. We also thank two reviewers and the editor, Claudine Stirling, for their constructive input.Editor: Claudine Stirling topReferences

Both ab initio calculations and isotope exchange experiments indicate that, at equilibrium, heavy 238U is enriched in the UIV oxidation state (Schauble, 2007; Abe et al., 2008; Wang et al., 2015). View in article
Alessi, D.S., Lezama-Pacheco, J.S., Stubbs, J.E., Janousch, M., Bargar, J.R., Persson, P., Bernier-Latmani, R. (2014) The product of microbial uranium reduction includes multiple species with U(IV)-phosphate coordination. Geochimica et Cosmochimica Acta 131, 115–127. https://doi.org/10.1016/j.gca.2014.01.005
We modelled the non-uraninite UIV as a cluster of ningyoite (CaU(PO4)2), a close analogue of the non-crystalline biotic reduction products (Bernier-Latmani et al., 2010; Alessi et al., 2014). View in article
Andersen, M.B., Stirling, C.H., Weyer, S. (2017) Uranium Isotope Fractionation. Reviews in Mineralogy and Geochemistry 82, 799–850. https://doi.org/10.2138/rmg.2017.82.19
Such U redox transformations are often accompanied by changes in the 238U/235U ratio, reported as δ238U (Andersen et al., 2017). View in articleAs U isotope fractionation is predominantly associated with redox transformations, U isotope signatures have been utilised as a (1) monitoring tool tuned specifically to the reductive rather than adsorptive removal of UVI during remediation (Bopp et al., 2010), and (2) palaeo-redox proxy, whereby the preferential reduction of 238U during marine anoxia is recorded in sedimentary rocks and can be used to reconstruct the pervasiveness of anoxia in past global oceans (Montoya-Pino et al., 2010; Brennecka et al., 2011; Andersen et al., 2017). View in articleIn such studies, the observation of lower δ238U (arising from NFSE-dominated mass-independent fractionation) is usually thought to indicate either a local shift in depositional conditions or water column stratification (Andersen et al., 2017; Brüske et al., 2020; Lau et al., 2022), or a shift in the U isotope mass balance, resulting from enhanced oceanic anoxic environments at regional or global scales (Montoya-Pino et al., 2010; Andersen et al., 2017). View in article
Basu, A., Sanford, R.A., Johnson, T.M., Lundstrom, C.C., Löffler, F.E. (2014) Uranium isotopic fractionation factors during U(VI) reduction by bacterial isolates. Geochimica et Cosmochimica Acta 136, 100–113. https://doi.org/10.1016/j.gca.2014.02.041
Under anoxic conditions, reduction of UVI to tetravalent U (UIV) can be mediated by an array of microorganisms or abiotically via Fe(II)- or sulfide-bearing compounds (Basu et al., 2014; Brown et al., 2018), resulting in the precipitation of sparingly soluble UIV species. View in articleThus, enrichment of 238U in UIV following UVI reduction has also been attributed to a dominant NFSE (Weyer et al., 2008; Basu et al., 2014, 2020; Stirling et al., 2015; Stylo et al., 2015), despite not necessarily representing isotopic equilibrium conditions. View in articleReduction of UVI by a range of bacterial species typically display enrichment of the heavier 238U in the reduced product, consistent with the predictions of NFS theory during equilibrium isotope fractionation (Basu et al., 2014). View in articleThese negative values indicate the preferential accumulation of lighter 235U in the reduced product, contrary to previous observations for microbial UVI reduction and at odds with NFS theory (Basu et al., 2014; Stirling et al., 2015; Stylo et al., 2015). View in article
Basu, A., Wanner, C., Johnson, T.M., Lundstrom, C., Sanford, R.A., Sonnenthal, E., Boyanov, M.I., Kemner, K.M. (2020) Microbial U isotope fractionation depends on U(VI) reduction rate. Environmental Science and Technology 54, 2295–2303. https://doi.org/10.1021/acs.est.9b05935
Thus, enrichment of 238U in UIV following UVI reduction has also been attributed to a dominant NFSE (Weyer et al., 2008; Basu et al., 2014, 2020; Stirling et al., 2015; Stylo et al., 2015), despite not necessarily representing isotopic equilibrium conditions. View in articleFurthermore, recent work has proposed that slow microbial reduction should impart significant mass-independent fractionation of up to +1 ‰ (Brown et al., 2018; Basu et al., 2020), whereas negative fractionation factors are typically only observed for rapid abiotic reductions, on the order of hours (Stylo et al., 2015). View in articleFurthermore, this study suggests that full expression of isotopic equilibrium in nature may be precluded by U speciation, in addition to the previous roles reported for electron flux and U supply dynamics (Basu et al., 2020; Brown et al., 2023a). View in article
Bernier-Latmani, R., Veeramani, H., Vecchia, E.D., Junier, P., Lezama-Pacheco, J.S., Suvorova, E.I., Sharp, J.O., Wigginton, N.S., Bargar, J.R. (2010) Non-uraninite products of microbial U (VI) reduction. Environmental Science and Technology 44, 9456–9462. https://doi.org/10.1021/es101675a
We modelled the non-uraninite UIV as a cluster of ningyoite (CaU(PO4)2), a close analogue of the non-crystalline biotic reduction products (Bernier-Latmani et al., 2010; Alessi et al., 2014). View in article
Bigeleisen, J. (1996) Nuclear size and shape effects in chemical reactions. Isotope chemistry of the heavy elements. Journal of the American Chemical Society 118, 3676–3680. https://doi.org/10.1021/ja954076k
This mass-independent fractionation arises from the nuclear field shift effect (NFSE), due to differences in the size and shape of the nuclei of heavy element isotopologues (Bigeleisen, 1996; Schauble, 2007). View in articleAt equilibrium, the NFSE is larger than, and operates in the opposite direction to, the conventional mass-dependent isotope effect, whereby the vibrational zero point energy of the lighter isotope leads to its enrichment in UIV as mass-dependent fractionation (MDF) (Bigeleisen, 1996; Schauble, 2007; Fujii et al., 2009). View in article
Bopp, C.J., Lundstrom, C.C., Johnson, T.M., Sandford, R.A., Long, P.E., Williams, K.H. (2010) Uranium 238U/235U isotope ratios as indicators of reduction: Results from an in situ biostimulation experiment at Rifle, Colorado, U.S.A. Environmental Science and Technology 44, 5927–5933. https://doi.org/10.1021/es100643v
As U isotope fractionation is predominantly associated with redox transformations, U isotope signatures have been utilised as a (1) monitoring tool tuned specifically to the reductive rather than adsorptive removal of UVI during remediation (Bopp et al., 2010), and (2) palaeo-redox proxy, whereby the preferential reduction of 238U during marine anoxia is recorded in sedimentary rocks and can be used to reconstruct the pervasiveness of anoxia in past global oceans (Montoya-Pino et al., 2010; Brennecka et al., 2011; Andersen et al., 2017). View in article
Brennecka, G.A., Herrmann, A.D., Algeo, T.J., Anbar, A.D. (2011) Rapid expansion of oceanic anoxia immediately before the end-Permian mass extinction. Proceedings of the National Academy of Sciences of the United States of America 108, 17631–17634. https://doi.org/10.1073/pnas.1106039108
As U isotope fractionation is predominantly associated with redox transformations, U isotope signatures have been utilised as a (1) monitoring tool tuned specifically to the reductive rather than adsorptive removal of UVI during remediation (Bopp et al., 2010), and (2) palaeo-redox proxy, whereby the preferential reduction of 238U during marine anoxia is recorded in sedimentary rocks and can be used to reconstruct the pervasiveness of anoxia in past global oceans (Montoya-Pino et al., 2010; Brennecka et al., 2011; Andersen et al., 2017). View in article
Brown, S.T., Basu, A., Ding, X., Christensen, J.N., DePaolo, D.J. (2018) Uranium isotope fractionation by abiotic reductive precipitation. Proceedings of the National Academy of Sciences of the United States of America 115, 8688–8693. https://doi.org/10.1073/pnas.1805234115
Under anoxic conditions, reduction of UVI to tetravalent U (UIV) can be mediated by an array of microorganisms or abiotically via Fe(II)- or sulfide-bearing compounds (Basu et al., 2014; Brown et al., 2018), resulting in the precipitation of sparingly soluble UIV species. View in articleFurthermore, recent work has proposed that slow microbial reduction should impart significant mass-independent fractionation of up to +1 ‰ (Brown et al., 2018; Basu et al., 2020), whereas negative fractionation factors are typically only observed for rapid abiotic reductions, on the order of hours (Stylo et al., 2015). View in article
Brown, A.R., Molinas, M., Roebbert, Y., Sato, A., Abe, M., Weyer, S., Bernier-Latmani, R. (2023a) Electron flux is a key determinant of uranium isotope fractionation during bacterial reduction. Communications Earth and Environment 4, 329. https://doi.org/10.1038/s43247-023-00989-x
The theory of this model has been demonstrated experimentally during UVI reduction by S. oneidensis, in which back reaction (reverse electron transfer) was limited by large electron fluxes from oxidation of the electron donor (Brown et al., 2023a). View in articleFurthermore, this study suggests that full expression of isotopic equilibrium in nature may be precluded by U speciation, in addition to the previous roles reported for electron flux and U supply dynamics (Basu et al., 2020; Brown et al., 2023a). View in article
Brown, A.R., Roebbert, Y., Sato, A., Hada, M., Abe, M., Weyer, S., Bernier-Latmani, R. (2023b) Contribution of the nuclear field shift to kinetic uranium isotope fractionation. Geochemical Perspectives Letters 27, 43–47. https://doi.org/10.7185/geochemlet.2333
Furthermore, during microbiological reduction of UVI-carbonate, the conventional isotopic mass effect was fully expressed, while the NFSE was not (Brown et al., 2023b). View in article
Brüske, A., Weyer, S., Zhao, M.Y., Planavsky, N.J., Wegwerth, A., Neubert, N., Dellwig, O., Lau, K.V., Lyons, T.W. (2020) Correlated molybdenum and uranium isotope signatures in modern anoxic sediments: Implications for their use as paleo-redox proxy. Geochimica et Cosmochimica Acta 270, 449–474. https://doi.org/10.1016/j.gca.2019.11.031
In such studies, the observation of lower δ238U (arising from NFSE-dominated mass-independent fractionation) is usually thought to indicate either a local shift in depositional conditions or water column stratification (Andersen et al., 2017; Brüske et al., 2020; Lau et al., 2022), or a shift in the U isotope mass balance, resulting from enhanced oceanic anoxic environments at regional or global scales (Montoya-Pino et al., 2010; Andersen et al., 2017). View in article
Faizova, R., Scopelliti, R., Chauvin, A.-S., Mazzanti, M. (2018) Synthesis and characterization of a water stable uranyl(V) complex. Journal of the American Chemical Society 140, 13554–13557. https://doi.org/10.1021/jacs.8b07885
The aminocarboxylate ligand dpaea2− (dpaeaH2 = bis(pyridyl-6-methyl-2-carboxylate)-ethylamine) can be used to precipitate both UVI and UIV whilst maintaining UV as an aqueous complex at circumneutral pH (Faizova et al., 2018). View in articleCyclic voltammograms of a UVO2-dpaea complex at pH 7 did not display a UV/UIV reduction event, suggesting slow electron transfer kinetics that may be related to required structural re-arrangements for the formation of a tri-nuclear UIV product (Faizova et al., 2018, 2020). View in articleThis hypothesis is consistent with the strong pentadentate coordination of UV by dpaea, which provides protection from ligand dissociation and cation-cation interactions typical of UV disproportionation (Faizova et al., 2018). View in article
Faizova, R., Fadaei‐Tirani, F., Bernier‐Latmani, R., Mazzanti, M. (2020) Ligand‐supported facile conversion of uranyl(VI) into uranium(IV) in organic and aqueous media. Angewandte Chemie 132, 6822–6825. https://doi.org/10.1002/ange.201916334
A slow second electron transfer step (UV/UIV) is consistent with abiotic reduction by sodium hydrosulfite (Faizova et al., 2020). View in articleCyclic voltammograms of a UVO2-dpaea complex at pH 7 did not display a UV/UIV reduction event, suggesting slow electron transfer kinetics that may be related to required structural re-arrangements for the formation of a tri-nuclear UIV product (Faizova et al., 2018, 2020). View in article
Fujii, T., Moynier, F., Albarède, F. (2009) The nuclear field shift effect in chemical exchange reactions. Chemical Geology 267, 139–156. https://doi.org/10.1016/j.chemgeo.2009.06.015
At equilibrium, the NFSE is larger than, and operates in the opposite direction to, the conventional mass-dependent isotope effect, whereby the vibrational zero point energy of the lighter isotope leads to its enrichment in UIV as mass-dependent fractionation (MDF) (Bigeleisen, 1996; Schauble, 2007; Fujii et al., 2009). View in article
Lau, K.V, Hancock, L.G., Severmann, S., Kuzminov, A., Cole, D.B., Behl, R.J., Planavsky, N.J., Lyons, T.W. (2022) Variable local basin hydrography and productivity control the uranium isotope paleoredox proxy in anoxic black shales. Geochimica et Cosmochimica Acta. 317, 433–456. https://doi.org/10.1016/j.gca.2021.10.011
In such studies, the observation of lower δ238U (arising from NFSE-dominated mass-independent fractionation) is usually thought to indicate either a local shift in depositional conditions or water column stratification (Andersen et al., 2017; Brüske et al., 2020; Lau et al., 2022), or a shift in the U isotope mass balance, resulting from enhanced oceanic anoxic environments at regional or global scales (Montoya-Pino et al., 2010; Andersen et al., 2017). View in article
Molinas, M., Faizova, R., Brown, A., Galanzew, J., Schacherl, B., Bartova, B., Meibom, K.L., Vitova, T., Mazzanti, M. and Bernier-Latmani, R. (2021) Biological reduction of a U(V)-organic ligand complex. Environmental Science and Technology 55, 4753–4761. https://doi.org/10.1021/acs.est.0c06633
During microbiological UVI reduction, two distinct mechanisms for the complete reduction to UIV can occur: either via disproportionation of two uranylV atoms (generating UVI and UIV) (Vettese et al., 2020), or via a second biologically mediated electron transfer to UV (Molinas et al., 2021, 2023). View in articleThese properties have allowed the reduction of UVI by Shewanella oneidensis to be followed, revealing the potential for the biological reduction of the UV intermediate, rather than its disproportionation (Molinas et al., 2021, 2023). View in articleCollectively, these data suggest that the first electron transfer was achieved rapidly, leading to the accumulation of UV in solution, in agreement with previous studies (Molinas et al., 2021, 2023). View in articlePrevious work confirms that reduction from UV to UIV is indeed mediated by electron transfer from S. oneidensis, as opposed to UV disproportionation (Molinas et al., 2021, 2023). View in articleTo ascertain whether this direction of fractionation reflected equilibrium in the peculiar case of a strong aminocarboxylate ligand, we performed ab initio calculations of the fractionation factor at equilibrium between UVO2-dpaea− and either UIV-(dpaea)2 or a non-uraninite UIV species, the two likely products of this biological reaction (Molinas et al., 2021). View in article
Molinas, M., Meibom, K.L., Faizova, R., Mazzanti, M., Bernier-Latmani, R. (2023) Mechanism of reduction of aqueous U(V)-dpaea and solid-phase U(VI)-dpaea complexes: The role of multiheme c-type cytochromes. Environmental Science and Technology 57, 7537–7546. https://doi.org/10.1021/acs.est.3c00666
During microbiological UVI reduction, two distinct mechanisms for the complete reduction to UIV can occur: either via disproportionation of two uranylV atoms (generating UVI and UIV) (Vettese et al., 2020), or via a second biologically mediated electron transfer to UV (Molinas et al., 2021, 2023). View in articleThese properties have allowed the reduction of UVI by Shewanella oneidensis to be followed, revealing the potential for the biological reduction of the UV intermediate, rather than its disproportionation (Molinas et al., 2021, 2023). View in articleCollectively, these data suggest that the first electron transfer was achieved rapidly, leading to the accumulation of UV in solution, in agreement with previous studies (Molinas et al., 2021, 2023). View in articlePrevious work confirms that reduction from UV to UIV is indeed mediated by electron transfer from S. oneidensis, as opposed to UV disproportionation (Molinas et al., 2021, 2023). View in articleIt is likely that reduction of UVIO2-dpaea proceeds via dissolution of the solid uranylVI followed by rapid reduction of aqueous uranylVI, i.e. dissolution is the rate limiting step for the first electron transfer (Molinas et al., 2023). View in article
Montoya-Pino, C., Anbar, A.D., van de Schootbrugge, B., Oschmann, W., Pross, J., Arz, H.W., Weyer, S. (2010) Global enhancement of ocean anoxia during Oceanic Anoxic Event 2: A quantitative approach using U isotopes. Geology 38, 315–318. https://doi.org/10.1130/G30652.1
As U isotope fractionation is predominantly associated with redox transformations, U isotope signatures have been utilised as a (1) monitoring tool tuned specifically to the reductive rather than adsorptive removal of UVI during remediation (Bopp et al., 2010), and (2) palaeo-redox proxy, whereby the preferential reduction of 238U during marine anoxia is recorded in sedimentary rocks and can be used to reconstruct the pervasiveness of anoxia in past global oceans (Montoya-Pino et al., 2010; Brennecka et al., 2011; Andersen et al., 2017). View in articleIn such studies, the observation of lower δ238U (arising from NFSE-dominated mass-independent fractionation) is usually thought to indicate either a local shift in depositional conditions or water column stratification (Andersen et al., 2017; Brüske et al., 2020; Lau et al., 2022), or a shift in the U isotope mass balance, resulting from enhanced oceanic anoxic environments at regional or global scales (Montoya-Pino et al., 2010; Andersen et al., 2017). View in article
Pan, Z., Bártová, B., Lagrange, T., Butorin, S.M., Hyatt, N.C., Stennett, M.C., Kvashnina, K.O., Bernier-latmani, R. (2020) Nanoscale mechanism of UO2 formation through uranium reduction by magnetite. Nature Communications 11, 1–12. https://doi.org/10.1038/s41467-020-17795-0
However, there is increasing evidence of the stabilisation and persistence of UV intermediates within abiotic and biological systems (Roberts et al., 2017; Pan et al., 2020). View in article
Roberts, H.E., Morris, K., Law, G.T.W., Mosselmans, J.F.W., Bots, P., Kvashnina, K., Shaw, S. (2017) Uranium(V) incorporation mechanisms and stability in Fe(II)/Fe(III) (oxyhydr)oxides. Environmental Science and Technology Letters 4, 421–426. https://doi.org/10.1021/acs.estlett.7b00348
However, there is increasing evidence of the stabilisation and persistence of UV intermediates within abiotic and biological systems (Roberts et al., 2017; Pan et al., 2020). View in article
Sato, A., Bernier-Latmani, R., Hada, M., Abe, M. (2021) Ab initio and steady-state models for uranium isotope fractionation in multi-step biotic and abiotic reduction. Geochimica et Cosmochimica Acta 307, 212–227. https://doi.org/10.1016/j.gca.2021.05.044
Ab initio calculations of the equilibrium isotope fractionation factor combined with a multi-step model of biological UVI-carbonate reduction suggests that fractionation factors of up to 1.6 ‰ for the UVI to UV step and ∼0.8 ‰ for the UV to UIV step (a total of ∼2.4 ‰) may be expected (Sato et al., 2021). View in articleRegardless of the abiotic equilibrium isotope exchange between reactants and products (independent of the bioreduction reaction), a recent model has demonstrated the importance of back reaction within the UVI bioreduction pathway in controlling the overall isotope fractionation (Sato et al., 2021). View in article
Schauble, E.A. (2007) Role of nuclear volume in driving equilibrium stable isotope fractionation of mercury, thallium, and other very heavy elements. Geochimica et Cosmochimica Acta 71, 2170–2189. https://doi.org/10.1016/j.gca.2007.02.004
Both ab initio calculations and isotope exchange experiments indicate that, at equilibrium, heavy 238U is enriched in the UIV oxidation state (Schauble, 2007; Abe et al., 2008; Wang et al., 2015). View in articleThis mass-independent fractionation arises from the nuclear field shift effect (NFSE), due to differences in the size and shape of the nuclei of heavy element isotopologues (Bigeleisen, 1996; Schauble, 2007). View in articleAt equilibrium, the NFSE is larger than, and operates in the opposite direction to, the conventional mass-dependent isotope effect, whereby the vibrational zero point energy of the lighter isotope leads to its enrichment in UIV as mass-dependent fractionation (MDF) (Bigeleisen, 1996; Schauble, 2007; Fujii et al., 2009). View in article
Stirling, C.H., Andersen, M.B., Warthmann, R., Halliday, A.N. (2015) Isotope fractionation of 238U and 235U during biologically-mediated uranium reduction. Geochimica et Cosmochimica Acta 163, 200–218. https://doi.org/10.1016/j.gca.2015.03.017
Thus, enrichment of 238U in UIV following UVI reduction has also been attributed to a dominant NFSE (Weyer et al., 2008; Basu et al., 2014, 2020; Stirling et al., 2015; Stylo et al., 2015), despite not necessarily representing isotopic equilibrium conditions. View in articleThese negative values indicate the preferential accumulation of lighter 235U in the reduced product, contrary to previous observations for microbial UVI reduction and at odds with NFS theory (Basu et al., 2014; Stirling et al., 2015; Stylo et al., 2015). View in article
Stylo, M., Neubert, N., Wang, Y., Monga, N., Romaniello, S.J., Weyer, S., Bernier-Latmani, R. (2015) Uranium isotopes fingerprint biotic reduction. Proceedings of the National Academy of Sciences of the United States of America 112, 5619–24. https://doi.org/10.1073/pnas.1421841112
Thus, enrichment of 238U in UIV following UVI reduction has also been attributed to a dominant NFSE (Weyer et al., 2008; Basu et al., 2014, 2020; Stirling et al., 2015; Stylo et al., 2015), despite not necessarily representing isotopic equilibrium conditions. View in articleThese negative values indicate the preferential accumulation of lighter 235U in the reduced product, contrary to previous observations for microbial UVI reduction and at odds with NFS theory (Basu et al., 2014; Stirling et al., 2015; Stylo et al., 2015). View in articleFurthermore, recent work has proposed that slow microbial reduction should impart significant mass-independent fractionation of up to +1 ‰ (Brown et al., 2018; Basu et al., 2020), whereas negative fractionation factors are typically only observed for rapid abiotic reductions, on the order of hours (Stylo et al., 2015). View in article
Vettese, G.F., Morris, K., Natrajan, L.S., Shaw, S., Vitova, T., Galanzew, J., Jones, D.L. and Lloyd, J.R. (2020) Multiple lines of evidence identify U(V) as a key intermediate during U(VI) reduction by Shewanella oneidensis MR1. Environmental Science and Technology 54, 2268–2276. https://doi.org/10.1021/acs.est.9b05285
During microbiological UVI reduction, two distinct mechanisms for the complete reduction to UIV can occur: either via disproportionation of two uranylV atoms (generating UVI and UIV) (Vettese et al., 2020), or via a second biologically mediated electron transfer to UV (Molinas et al., 2021, 2023). View in article
Wang, X., Johnson, T.M., Lundstrom, C.C. (2015) Low temperature equilibrium isotope fractionation and isotope exchange kinetics between U(IV) and U(VI). Geochimica et Cosmochimica Acta 158, 262–275. https://doi.org/10.1016/j.gca.2015.03.006
Both ab initio calculations and isotope exchange experiments indicate that, at equilibrium, heavy 238U is enriched in the UIV oxidation state (Schauble, 2007; Abe et al., 2008; Wang et al., 2015). View in article
Weyer, S., Anbar, A.D., Gerdes, A., Gordon, G.W., Algeo, T.J., Boyle, E.A. (2008) Natural fractionation of 238U/235U. Geochimica et Cosmochimica Acta 72, 345–359. https://doi.org/10.1016/j.gca.2007.11.012
Thus, enrichment of 238U in UIV following UVI reduction has also been attributed to a dominant NFSE (Weyer et al., 2008; Basu et al., 2014, 2020; Stirling et al., 2015; Stylo et al., 2015), despite not necessarily representing isotopic equilibrium conditions. View in article
Supplementary Information
The Supplementary Information includes:- Experimental Section
- Figures S-1 to S-3
- Table S-1
- Supplementary Information References
Figures
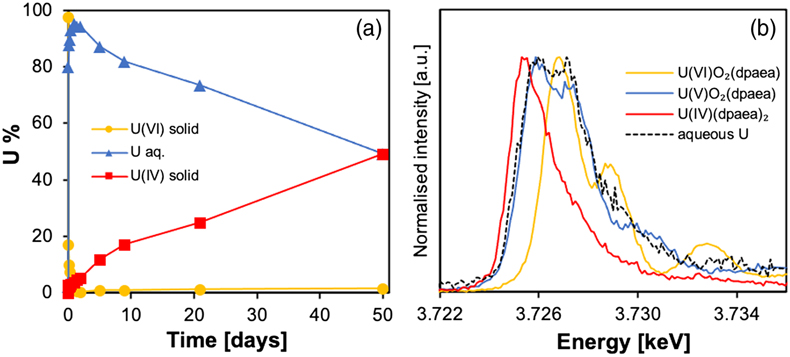


