Extreme 18O-enrichment in majorite constrains a crustal origin of transition zone diamonds
Affiliations | Corresponding Author | Cite asKeywords: diamond, majorite, oxygen isotopes, carbon isotopes, transition zone
- Share this article
-
Article views:10,987Cumulative count of HTML views and PDF downloads.
- Download Citation
- Rights & Permissions
Abstract

Figures and Tables
![]() Figure 1 The chemical composition of the Jagersfontein majoritic garnet inclusions based on a formula unit containing twelve oxygens, and their relationship to minimum entrapment depth. The elongate ellipses are the chemical compositions of the garnets and the size and shape of the ellipse represents the 95 % confidence limit based on electron microprobe uncertainties. The small circles are experimental results (from the compilation of Collerson et al., 2010, with the addition of data from Irifune et al., 1986), with colour shading corresponding to the apparent depth that their pressure of equilibration represents. For clarity, uncertainties are not shown on the experimental results; however they are approximately the same size as the uncertainties for the natural garnets. Depth contours are derived from a linear regression of the experimental data relating Si to equilibration pressure. | ![]() Table 1 Oxygen and carbon isotope compositions of majoritic inclusions and their diamond hosts, respectively. The carbon isotope compositions are from Tappert et al. (2005b). Uncertainties on δ18O values include repeatability of the reference material (RM), the calibration uncertainty, and the uncertainty in the bulk values of the RM. Note that diamonds JF-01 and JF-37 have two inclusions each. | ![]() Figure 2 The δ18O compositions of the majoritic garnets and the δ13C values of their host diamonds. The majoritic garnets and their diamond hosts plot well away from the mantle field. The histogram on the Y-axis is of garnets from eclogite xenoliths (compilation of Ickert et al., 2013), the histogram on the X-axis is of cratonic diamonds (Stachel et al., 2009). |
Figure 1 | Table 1 | Figure 2 |
Supplementary Figures and Tables
![]() Figure S-1 Time series of the majorite analyses. All data are plotted as raw isotope ratios relative to 0.0020052, corrected only for amplifier gains and backgrounds. There are no corrections needed for drift or to eliminate outliers. Tie lines connect analyses of the same grain. | ![]() Figure S-2 Magnitude of the matrix correction. Each pair of points represents a single analysis, with the grey point representing the value prior to correction for the matrix effect, and the black point representing the value after the matrix correction. For reference, secondary reference material UAJM is plotted, which, after making a much larger correction for matrix effects than needed for the majorities, agrees within uncertainty with the nominal reference value. | ![]() Figure S-3 Plot of each pair of analyses from the same grain. The analyses all agree within uncertainty, suggesting that the uncertainties have been estimated correctly. | ![]() Table S-1 Full analytical data for oxygen isotope measurements of majorites. |
Figure S-1 | Figure S-2 | Figure S-3 | Table S-1 |
top
Introduction
The volatile abundance, bulk composition, and nature of deep Earth are unresolved geochemical questions (e.g., Hirschmann and Dasgupta, 2009
Hirschmann, M.M., Dasgupta, R. (2009) The H/C ratios of Earth’s near-surface and deep reservoirs, and consequences for deep Earth volatile cycles. Chemical Geology 262, 4–16.
). Diamonds and their mineral inclusions are the only materials available from sublithospheric depths and provide the best geochemical information from these otherwise inaccessible regions (Stachel et al., 2005Stachel, T., Brey, G.P., Harris, J.W. (2005) Inclusions in sublithospheric diamonds: Glimpses of deep Earth. Elements 1, 73–78.
). Such ultra-deep diamonds have been interpreted as having formed in subducted oceanic crust based on trace element patterns of their inclusions showing negative Eu anomalies and 13C depletion of their diamond hosts (Tappert et al., 2005bTappert, R., Stachel, T., Harris, J.W., Muehlenbachs, K., Ludwig, T., Brey, G.P. (2005b) Subducting oceanic crust: The source of deep diamonds. Geology 33, 565–568.
). However, this interpretation is non-unique and controversial (Cartigny, 2005Cartigny, P. (2005) Stable isotopes and the origin of diamond. Elements 1, 79–84.
; Corgne et al., 2012Corgne, A., Armstrong, L.S., Keshav, S., Fei, Y., McDonough, W.F., Minarik, W.G., Moreno, K. (2012) Trace element partitioning between majoritic garnet and silicate melt at 10–17 GPa: Implications for deep mantle processes. Lithos 148, 128–141.
). To understand Earth’s deep carbon cycle it is, however, of key importance to constrain definitively or refute whether diamond acts as a carrier of crustal derived carbon in deeply subducting slabs. To resolve this question, we present the first analysis of oxygen isotope compositions of ultradeep inclusions – majoritic garnets in diamond from Jagersfontein (South Africa).The carbon isotopic systematics of the mantle – which may contain more carbon than the crust-biosphere system (Hirschmann and Dasgupta, 2009
Hirschmann, M.M., Dasgupta, R. (2009) The H/C ratios of Earth’s near-surface and deep reservoirs, and consequences for deep Earth volatile cycles. Chemical Geology 262, 4–16.
) – are not well understood. Much of the chemical information that we have on deep carbon comes from the study of diamonds and their inclusions (Deines, 1980Deines, P. (1980) The carbon isotopic composition of diamonds: Relationship to diamond shape, color, occurrence and vapor composition. Geochimica et Cosmochimica Acta 44, 943–961.
). From studies of the minerals included in diamonds, it is clear that the vast majority are sourced from the lithospheric mantle. However, there is a small fraction of inclusion assemblages that imply trapping in the asthenosphere, transition zone, or even the lower mantle (Stachel et al., 2005Stachel, T., Brey, G.P., Harris, J.W. (2005) Inclusions in sublithospheric diamonds: Glimpses of deep Earth. Elements 1, 73–78.
). Much of the difficulty in understanding the nature of the deep carbon cycle revolves around this diamond material. The carbon isotope ratios (δ13C values) in diamond have a wide range – from -41 ‰ to +3 ‰ (Deines, 1980Deines, P. (1980) The carbon isotopic composition of diamonds: Relationship to diamond shape, color, occurrence and vapor composition. Geochimica et Cosmochimica Acta 44, 943–961.
; Cartigny, 2005Cartigny, P. (2005) Stable isotopes and the origin of diamond. Elements 1, 79–84.
; De Stefano et al., 2009De Stefano, A., Kopylova, M.G., Cartigny, P., Afanasiev, V. (2009) Diamonds and eclogites of the Jericho kimberlite (Northern Canada). Contributions to Mineralogy and Petrology 158, 295–315.
) – however, there is controversy over whether the observed variability represents sampling of distinct, unhomogenised, primitive carbon domains, fractionation at mantle conditions, or subduction of carbon that was fractionated by near-surface processes (Cartigny, 2005Cartigny, P. (2005) Stable isotopes and the origin of diamond. Elements 1, 79–84.
). Though mainly formulated in the context of cratonic diamonds, these considerations apply equally to the origin of ultradeep diamonds. δ13C values for sub-lithospheric diamond have a wide range (from 0 ‰ to -25 ‰; Stachel et al., 2002Stachel, T., Harris J.W., Aulbach, S., Deines, P. (2002) Kankan diamonds (Guinea) III: δ13C and nitrogen characteristics of deep diamonds. Contributions to Mineralogy and Petrology 142, 465–475.
; Tappert et al., 2005aTappert, R., Stachel, T., Harris, J.W., Muehlenbachs, K., Ludwig, T., Brey, G.P. (2005a) Diamonds from Jagersfontein (South Africa): Messengers from the sublithospheric mantle. Contributions to Mineralogy and Petrology 150, 505–522.
; Walter et al., 2011Walter, M.J., Kohn, S.C., Araujo, D., Bulanova, G.P., Smith, C.B., Gaillou, E., Wang, J., Steele, A., Shirey, S.B. (2011) Deep mantle cycling of oceanic crust: Evidence from diamonds and their mineral inclusions. Science 334, 54–57.
) and have often been interpreted as reflecting incorporation of subducted carbon, thereby documenting deep subduction and the subsequent return of originally crustal carbon. Nevertheless, this interpretation is not unique. For example, Mikhail et al. (2014)Mikhail, S., Guillermier, C., Franchi, I.A., Beard, A.D., Crispin, K., Verchovsky, A.B., Jones, A.P., Milledge, H.J. (2014) Empirical evidence for the fractionation of carbon isotopes between diamond and iron carbide from the Earth’s mantle. Geochemistry, Geophysics, Geosystems 15, 855–866.
suggested that iron carbide inclusions in diamonds from Jagersfontein document isotopic fractionation (Δ13Ccarbide-diamond >7 ‰) sufficiently strong to cause the observed 13C depleted signature. Similarly, evidence based on the rare earth element signatures (observation of negative Eu anomalies) of majoritic garnet inclusions from Jagersfontein, interpreted to reflect feldspar fractionation in crustal protoliths (Tappert et al., 2005bTappert, R., Stachel, T., Harris, J.W., Muehlenbachs, K., Ludwig, T., Brey, G.P. (2005b) Subducting oceanic crust: The source of deep diamonds. Geology 33, 565–568.
), was disputed by Griffin and O’Reilly (2007)Griffin, W.L., O’Reilly, S.Y. (2007) Cratonic lithospheric mantle: Is anything subducted? Episodes 30, 43–53.
and Corgne et al. (2012)Corgne, A., Armstrong, L.S., Keshav, S., Fei, Y., McDonough, W.F., Minarik, W.G., Moreno, K. (2012) Trace element partitioning between majoritic garnet and silicate melt at 10–17 GPa: Implications for deep mantle processes. Lithos 148, 128–141.
who considered Eu anomalies to reflect redox-related metasomatic signatures, or crystallisation from a melt at high pressures.In contrast, oxygen isotope compositions (δ18OVSMOW values) in mantle peridotites, primitive basalts, and the moon have a narrow range, deviating only slightly from +5.5 ‰ (Eiler, 2001
Eiler, J.M. (2001) Oxygen isotope variations of basaltic lavas and upper mantle rocks. In: Valley, J.W., Cole, D.R., Rosso, J.J. (Eds.) Reviews in Mineralogy and Geochemistry 43, 415–468.
). This gross homogeneity is a consequence of an initially well-mixed Earth (Pahlevan and Stevenson, 2007Pahlevan, K., Stevenson, D.J. (2007) Equilibration in the aftermath of the lunar-forming giant impact. Earth and Planetary Science Letters 262, 438–449.
), and small values of mass dependent stable isotope fractionation at high temperatures which prevent mantle silicates from substantially fractionating oxygen isotopes among each other (Urey, 1947Urey, H.C. (1947) The thermodynamic properties of isotopic substances. Journal of the Chemical Society (Resumed), 562–562.
; Criss, 1991Criss, R.E. (1991) Temperature dependence of isotopic fractionation factors. In: Taylor, H.P., O’Neil, J.R., Kaplan, I.R. (Eds.) Stable Isotope Geochemistry. The Geochemical Society Special Publication 3, 11–16.
). Crustal rocks typically have higher δ18O values mainly within the range +6 to +12 ‰ (Simon and Lécuyer, 2005Simon, L., Lécuyer, C. (2005) Continental recycling: The oxygen isotope point of view. Geochemistry, Geophysics, Geosystems 6, 1–10.
) due to low-temperature reactions involving water. In rare cases, crustal rocks can become relatively depleted in 18O, with values below +5.5 ‰ (Taylor, 1968Taylor, H.P. (1968) The oxygen isotope geochemistry of igneous rocks. Contributions to Mineralogy and Petrology 19, 1–71.
). Thus, variation makes oxygen isotope compositions a uniquely robust tracer of crustal material in the mantle (Eiler et al., 2000Eiler, J.M., Schiano, P., Kitchen, N., Stolper, E.M. (2000) Oxygen-isotope evidence for recycled crust in the sources of mid-ocean-ridge basalts. Nature 403, 530–534.
; Schulze et al., 2003Schulze, D.J., Harte, B., Valley, J.W., Brenan, J.M., Channer, D.M.D.R. (2003) Extreme crustal oxygen isotope signatures preserved in coesite in diamond. Nature 423, 68–70.
). This unique feature has previously been leveraged to test the origin of carbon isotope anomalies in lithospheric diamonds. Measuring the oxygen isotope composition of coesite (Schulze et al., 2003Schulze, D.J., Harte, B., Valley, J.W., Brenan, J.M., Channer, D.M.D.R. (2003) Extreme crustal oxygen isotope signatures preserved in coesite in diamond. Nature 423, 68–70.
) and eclogitic garnet inclusions (Schulze et al., 2013Schulze, D.J., Harte, B., EIMF staff, Page, F.Z., Valley, J.W., Channer, D.M.D.R., Jaques, A.L. (2013) Anticorrelation between low δ13C of eclogitic diamonds and high δ18O of their coesite and garnet inclusions requires a subduction origin. Geology 41, 455-458.
; Ickert et al., 2013Ickert, R.B., Stachel, T., Stern, R.A., Harris, J.W. (2013) Diamond from recycled crustal carbon documented by coupled δ18O–δ13C measurements of diamonds and their inclusions. Earth and Planetary Science Letters 364, 85–97.
) in diamond, a coupled relationship of high δ18O values (inclusions) and low δ13C values (host diamonds) emerged, constraining a crustal origin of both the diamond carbon and the diamond substrate. For sublithospheric diamonds, similar evidence involving oxygen isotopic analyses of inclusions has so far been lacking.Rare diamonds from Jagersfontein (South Africa) contain inclusions of majoritic garnet (Deines et al., 1991
Deines, P., Harris, J.W., Gurney, J.J. (1991) The carbon isotopic composition and nitrogen content of lithospheric and asthenospheric diamonds from the Jagersfontein and Koffiefontein kimberlite, South Africa. Geochimica et Cosmochimica Acta 55, 2615–2625.
; Tappert et al., 2005bTappert, R., Stachel, T., Harris, J.W., Muehlenbachs, K., Ludwig, T., Brey, G.P. (2005b) Subducting oceanic crust: The source of deep diamonds. Geology 33, 565–568.
), an ultra-high pressure mineral reflecting the increasing solubility of pyroxene in the garnet structure at sub-lithospheric depth (Ringwood and Major, 1971Ringwood, A.E., Major, A. (1971) Synthesis of majorite and other high pressure garnets and perovskites. Earth and Planetary Science Letters 12, 411–418.
). Experimental work (e.g., Akaogi and Akimoto, 1977Akaogi, M., Akimoto, S. (1977) Pyroxene-garnet solid-solution equilibria in the systems Mg4Si4O12 -Mg3Al2Si3O12 and Fe4Si4O12-Fe3Al2Si3O12 at high pressures and temperatures. Physics of the Earth and Planetary Interiors 15, 90–106.
; Irifune et al., 1986Irifune, T., Sekine, T., Ringwood, A.E., Hibberson, W.O. (1986) The eclogite-garnetite transformation at high pressure and some geophysical implications. Earth and Planetary Science Letters 77, 245–256.
) demonstrated a linear relationship between the solubility of pyroxene (which can be parameterised by Si-excess over the available tetrahedral sites) and pressure, thereby providing crude minimum depth estimates. The studied inclusions from Jagersfontein have Si contents of up to 3.5 atoms per formula unit, indicating that some of the inclusions came from depths of at least 500 km (Tappert et al., 2005bTappert, R., Stachel, T., Harris, J.W., Muehlenbachs, K., Ludwig, T., Brey, G.P. (2005b) Subducting oceanic crust: The source of deep diamonds. Geology 33, 565–568.
), well within the transition zone (Fig. 1). Based on low Cr contents (<0.4 wt. %) the majoritic garnets are classified as "eclogitic", or based on their low Na concentrations, metapyroxenitic (Gurney, 1984Gurney, J.J. (1984) A correlation between garnets and diamonds in kimberlites. In: Glover, J.E., Harris, P.G. (Eds.) Kimberlite Occurrence and Origin: A Basis for Conceptual Models in Exploration. University of Western Australia Special Publication, 143–166.
; Schulze, 2003Schulze, D.J. (2003) A classification scheme for mantle-derived garnets in kimberlite: A tool for investigating the mantle and exploring for diamonds. Lithos 71, 195–213.
; Kiseeva et al., 2012Kiseeva, E.S., Yaxley, G.M., Hermann, J., Litasov, K.D., Rosenthal, A., Kamenetsky, V.S. (2012) An experimental study of carbonated eclogite at 3.5-5.5 GPa — implications for silicate and carbonate metasomatism in the cratonic mantle. Journal of Petrology 53, 727–759.
). The relatively high molar Mg/(Mg+Fe) (0.68-0.83) of these inclusions preclude derivation from typical pelitic sediments.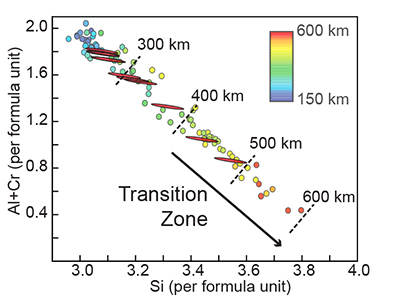
Figure 1 The chemical composition of the Jagersfontein majoritic garnet inclusions based on a formula unit containing twelve oxygens, and their relationship to minimum entrapment depth. The elongate ellipses are the chemical compositions of the garnets and the size and shape of the ellipse represents the 95 % confidence limit based on electron microprobe uncertainties. The small circles are experimental results (from the compilation of Collerson et al., 2010
Collerson, K.D., Williams, Q., Kamber, B.S., Omori, S., Arai, H., Ohtani, E. (2010) Majoritic garnet: A new approach to pressure estimation of shock events in meteorites and the encapsulation of sub-lithospheric inclusions in diamond. Geochimica et Cosmochimica Acta 74, 5939–5957.
, with the addition of data from Irifune et al., 1986Irifune, T., Sekine, T., Ringwood, A.E., Hibberson, W.O. (1986) The eclogite-garnetite transformation at high pressure and some geophysical implications. Earth and Planetary Science Letters 77, 245–256.
), with colour shading corresponding to the apparent depth that their pressure of equilibration represents. For clarity, uncertainties are not shown on the experimental results; however they are approximately the same size as the uncertainties for the natural garnets. Depth contours are derived from a linear regression of the experimental data relating Si to equilibration pressure.Methods
Mineral inclusions in diamonds have generally presented a unique analytical challenge because of their small size. Non-destructive or microbeam techniques are preferred because they consume little or no sample. Although Lowry et al. (1999)
Lowry, D., Mattey, D.P., Harris, J.W. (1999) Oxygen isotope composition of syngenetic inclusions in diamond from the Finsch Mine, RSA. Geochimica et Cosmochimica Acta 63, 1825–1836.
employed a novel, bulk laser fluorination technique to measure oxygen isotope compositions of mineral inclusions in diamond, most subsequent workers have employed secondary ion mass spectrometry (SIMS) for δ18O measurements (Schulze et al., 2003Schulze, D.J., Harte, B., Valley, J.W., Brenan, J.M., Channer, D.M.D.R. (2003) Extreme crustal oxygen isotope signatures preserved in coesite in diamond. Nature 423, 68–70.
; Smart et al., 2012Smart, K.A., Chacko, T., Stachel, T., Tappe, S., Stern, R.A., Ickert, R.B., EIMF staff (2012) Eclogite formation beneath the northern Slave craton constrained by diamond inclusions: Oceanic lithosphere origin without a crustal signature. Earth and Planetary Science Letters 319-320, 165–177.
; Ickert et al., 2013Ickert, R.B., Stachel, T., Stern, R.A., Harris, J.W. (2013) Diamond from recycled crustal carbon documented by coupled δ18O–δ13C measurements of diamonds and their inclusions. Earth and Planetary Science Letters 364, 85–97.
; Schulze et al., 2013Schulze, D.J., Harte, B., EIMF staff, Page, F.Z., Valley, J.W., Channer, D.M.D.R., Jaques, A.L. (2013) Anticorrelation between low δ13C of eclogitic diamonds and high δ18O of their coesite and garnet inclusions requires a subduction origin. Geology 41, 455-458.
).We made high-precision SIMS δ18O measurements on the majorite inclusions in diamond originally studied by Tappert et al. (2005b)
Tappert, R., Stachel, T., Harris, J.W., Muehlenbachs, K., Ludwig, T., Brey, G.P. (2005b) Subducting oceanic crust: The source of deep diamonds. Geology 33, 565–568.
, who published trace element measurements of the majorities and carbon isotope compositions of their host diamonds. Analytical procedures follow Ickert and Stern (2013)Ickert, R.B., Stern, R.A. (2013) Matrix corrections and error analysis in high-precision SIMS 18O/16O measurements of Ca–Mg–Fe garnet. Geostandards and Geoanalytical Research 37, 429–448.
, with modifications described in the Supplementary Information. As in previous studies (e.g., Schulze et al., 2013Schulze, D.J., Harte, B., EIMF staff, Page, F.Z., Valley, J.W., Channer, D.M.D.R., Jaques, A.L. (2013) Anticorrelation between low δ13C of eclogitic diamonds and high δ18O of their coesite and garnet inclusions requires a subduction origin. Geology 41, 455-458.
), the inclusions, which are <200 μm in their largest dimension, were released by crushing the host diamond, and mounted in epoxy grain mounts along with reference materials. The data are summarised in Table 1, and the full dataset is available in the Supplementary Information Table S-1. Each majorite sample was analysed twice, and in each case the two spots were identical within uncertainty. A major obstacle to accurate SIMS analysis is the dependence of the instrumental mass fractionation on the chemical composition and structure of the target material, the so called “matrix effect” (Russell et al., 1980Russell, W.A., Papanastassiou, D.A., Tombrello, T.A. (1980) The fractionation of Ca isotopes by sputtering. Radiation Effects and Defects in Solids 52, 41–52.
; Eiler et al., 1997Eiler, J.M., Graham, C., Valley, J.W. (1997) SIMS analysis of oxygen isotopes: Matrix effects in complex minerals and glasses. Chemical Geology 138, 221–244.
; Vielzeuf et al., 2005Vielzeuf, D., Champenois, M., Valley, J.W., Brunet, F., Devidal, J.L. (2005) SIMS analyses of oxygen isotopes: Matrix effects in Fe-Mg-Ca garnets. Chemical Geology 223, 208–226.
; Page et al., 2010Page, F.Z., Kita, N.T., Valley, J.W. (2010) Ion microprobe analysis of oxygen isotopes in garnets of complex chemistry. Chemical Geology 270, 9–19.
). We employ the technique described by Page et al. (2010)Page, F.Z., Kita, N.T., Valley, J.W. (2010) Ion microprobe analysis of oxygen isotopes in garnets of complex chemistry. Chemical Geology 270, 9–19.
to correct for the bias between the primary reference material and the majorite samples based on Ca-content. However, we have no reference material for which we can correct for the majorite component. Since we observe a uniformly heavy oxygen isotope composition at a wide range of abundances of majorite component (including nearly no majorite), we infer that the matrix effect associated with the majorite substitution is small and is not responsible for the heavy oxygen compositions. The range in majorite compositions mainly reflects the variable trapping depth, and therefore the solubility of pyroxene in the garnet. The total uncertainty for each spot analysis is approximately ±0.4 ‰ (2σ), which is mainly due to overdispersion of the primary reference material (±0.3 ‰) and the matrix correction (±0.2 ‰), but includes the uncertainty in the primary reference material and the uncertainty in the session-level mass fractionation correction (see Ickert and Stern, 2013Ickert, R.B., Stern, R.A. (2013) Matrix corrections and error analysis in high-precision SIMS 18O/16O measurements of Ca–Mg–Fe garnet. Geostandards and Geoanalytical Research 37, 429–448.
for details).Table 1 Oxygen and carbon isotope compositions of majoritic inclusions and their diamond hosts, respectively. The carbon isotope compositions are from Tappert et al. (2005b)
Tappert, R., Stachel, T., Harris, J.W., Muehlenbachs, K., Ludwig, T., Brey, G.P. (2005b) Subducting oceanic crust: The source of deep diamonds. Geology 33, 565–568.
. Uncertainties on δ18O values include repeatability of the reference material (RM), the calibration uncertainty, and the uncertainty in the bulk values of the RM. Note that diamonds JF-01 and JF-37 have two inclusions each.Alias | Spot ID | δ18OVSMOW | Mean | ±95 % c.l. | δ13CVPDB |
JF-01A | S1318_1 | 8.78 | 8.88 | 0.37 | -21.2 |
S1318_2 | 8.97 | ||||
JF-01B | S1319_1 | 9.07 | 9.20 | 0.37 | -21.2 |
S1319_2 | 9.32 | ||||
JF-09A | S1320_1 | 9.94 | 10.00 | 0.37 | -21.8 |
S1320_2 | 10.06 | ||||
JF-22A | S1321_1 | 9.02 | 9.06 | 0.37 | -17.3 |
S1321_2 | 9.09 | ||||
JF-37A | S1322_1 | 9.28 | 9.32 | 0.37 | -21.7 |
S1322_2 | 9.36 | ||||
JF-37B | S1323_1 | 9.71 | 9.72 | 0.37 | -21.7 |
S1323_2 | 9.73 | ||||
JF-39A | S1324_1 | 9.19 | 9.09 | 0.37 | -17.9 |
S1324_2 | 8.98 | ||||
JF-42A | S1325_1 | 9.26 | 9.21 | 0.37 | -17.4 |
S1325_2 | 9.15 | ||||
JF-44B | S1326_1 | 8.62 | 8.74 | 0.38 | -17.7 |
S1326_2 | 8.85 | ||||
JF-55A | S1328_1 | 8.62 | 8.57 | 0.38 | -17.6 |
S1328_2 | 8.52 | ||||
JF-58B | S1331_1 | 9.14 | 9.21 | 0.38 | -23.0 |
S1331_2 | 9.28 |
top
Discussion and Conclusions
The measured δ18O values for the inclusions fall into a small range from +8.6 ‰ to +10.0 ‰ (Fig. 2). These values far exceed the range of upper mantle peridotite and derivative melts (+5.5 ±0.4 ‰; Mattey et al., 1994
Mattey, D., Lowry, D., Macpherson, C. (1994) Oxygen-isotope composition of mantle peridotite. Earth and Planetary Science Letters 128, 231–241.
); in the crust, values this high (in silicate rocks) are only associated with strong interactions between rocks and water at low temperature (Taylor and Sheppard, 1986Taylor, H.P., Sheppard, S.M.F. (1986) Igneous rocks: 1. Processes of isotopic fractionation and isotope systematics. In: Valley, J.W., Taylor, H.P., O’Neil, J.R. (Eds.) Stable Isotopes in High Temperature Geological Processes. Reviews in Mineralogy. Mineralogical Society of America, 227–271.
). High temperature processes, such as CO2 escape from carbon-rich melts, can fractionate both carbon and oxygen isotope ratios (Deines, 1980Deines, P. (1980) The carbon isotopic composition of diamonds: Relationship to diamond shape, color, occurrence and vapor composition. Geochimica et Cosmochimica Acta 44, 943–961.
; Ickert et al., 2013Ickert, R.B., Stachel, T., Stern, R.A., Harris, J.W. (2013) Diamond from recycled crustal carbon documented by coupled δ18O–δ13C measurements of diamonds and their inclusions. Earth and Planetary Science Letters 364, 85–97.
), however this will only occur under unusual circumstances, and the magnitudes of the fractionations are relatively small and cannot account for >4 ‰ shifts. Moreover, if the carbon isotope data are also considered, there are no processes that can simultaneously create depletion in 13C and enrichment in 18O (Ickert et al., 2013Ickert, R.B., Stachel, T., Stern, R.A., Harris, J.W. (2013) Diamond from recycled crustal carbon documented by coupled δ18O–δ13C measurements of diamonds and their inclusions. Earth and Planetary Science Letters 364, 85–97.
). Cartigny (2010)Cartigny, P. (2010) Mantle-related carbonados? Geochemical insights from diamonds from the Dachine komatiite (French Guiana). Earth and Planetary Science Letters 296, 329–339.
suggested that the depleted carbon isotope signatures of the Jagersfontein transition zone-suite of diamonds were derived from a primordial mantle reservoir and not from crustal materials. The presence of a previously undetected high-δ18O, primordial reservoir in the deep Earth is unlikely, given the homogeneity of the mantle and the near identical oxygen isotope systematics between the Earth and Moon (Wiechert et al., 2001Wiechert, U., Halliday, A.N., Lee, D.C., Snyder, G.A., Taylor, L.A., Rumble, D. (2001) Oxygen isotopes and the Moon-forming giant impact. Science 294, 345–348.
), so we suggest that this hypothesis is now untenable.These new data establish a link between the high δ18O values of inclusions and the low 13C values of the diamond hosts. This relationship is firm evidence for a subducted protolith and makes it extremely unlikely that the associated negative δ13C values are related to deep mantle isotopic fractionation (Mikhail et al., 2014
Mikhail, S., Guillermier, C., Franchi, I.A., Beard, A.D., Crispin, K., Verchovsky, A.B., Jones, A.P., Milledge, H.J. (2014) Empirical evidence for the fractionation of carbon isotopes between diamond and iron carbide from the Earth’s mantle. Geochemistry, Geophysics, Geosystems 15, 855–866.
). In a subduction scenario, the low δ13C values (Fig. 2) imply that the carbon originated not as carbonate but as organic carbon – either residual from biological processes or produced abiotically by Fischer-Tropsch-type reactions. The δ18O values require that the host rocks were strongly weathered, and probably underwent substantial secondary hydration on the sea floor.This δ18O-δ13C relationship implies that the recovered ultradeep inclusions were derived from intensely hydrated (e.g., δ18O >> +7 ‰) and typically oxidised basaltic rock close to the seawater interface, and that the diamond carbon was closely associated with this protolith. Such rocks have a low relative abundance in the oceanic crust, and the coincidence of their rarity and their apparent association with diamonds most likely attests to a causal relationship. That reduced, rather than oxidised, carbon is involved suggests that after thermal cracking, the carbon is subducted as metastable graphite. Since conversion of metastable graphite to diamond is not expected to produce macrocrystals (Sung, 2000
Sung, J. (2000) Graphite - diamond transition under high pressure: A kinetics approach. Journal of Materials Science 35, 6041-6054
), diamond formation during a fluid/melt aided dissolution – precipitation process is likely. The volatile-rich nature of the protolith is consistent with the recent suggestion by Harte (2010)Harte, B. (2010) Diamond formation in the deep mantle: The record of mineral inclusions and their distribution in relation to mantle dehydration zones. Mineralogical Magazine 74, 189–215.
that ultradeep diamonds are associated with devolatilisation and possibly melting reactions.
Figure 2 The δ18O compositions of the majoritic garnets and the δ13C values of their host diamonds. The majoritic garnets and their diamond hosts plot well away from the mantle field. The histogram on the Y-axis is of garnets from eclogite xenoliths (compilation of Ickert et al., 2013
Ickert, R.B., Stachel, T., Stern, R.A., Harris, J.W. (2013) Diamond from recycled crustal carbon documented by coupled δ18O–δ13C measurements of diamonds and their inclusions. Earth and Planetary Science Letters 364, 85–97.
), the histogram on the X-axis is of cratonic diamonds (Stachel et al., 2009Stachel, T., Harris, J.W., Muehlenbachs, K. (2009) Sources of carbon in inclusion bearing diamonds. Lithos 112(S2), 625-637.
).top
Acknowledgements
Full datasets and methods can be found in the Supplementary Information. The diamonds for this project were gifted to JWH by the Diamond Trading Company, a member of the DeBeers Group of Companies. DeBeers are sincerely thanked for this material. RBI was supported by a Killam Memorial Postdoctoral Fellowship. The Canadian Centre for Isotopic Microanalysis (CCIM) was created through Canadian Foundation for Innovation and Alberta Science and Research Investment Program grants to TS. TS acknowledges support through a Natural Sciences and Engineering Research Council of Canada Discovery grant and the Canada Research Chairs program. This is a contribution of CCIM project P1014.
Editor: Bruce Watson
top
References
Akaogi, M., Akimoto, S. (1977) Pyroxene-garnet solid-solution equilibria in the systems Mg4Si4O12 -Mg3Al2Si3O12 and Fe4Si4O12-Fe3Al2Si3O12 at high pressures and temperatures. Physics of the Earth and Planetary Interiors 15, 90–106.

Experimental work (e.g., Akaogi and Akimoto, 1977; Irifune et al., 1986) demonstrated a linear relationship between the solubility of pyroxene (which can be parameterised by Si-excess over the available tetrahedral sites) and pressure, thereby providing crude minimum depth estimates.
View in article
Cartigny, P. (2005) Stable isotopes and the origin of diamond. Elements 1, 79–84.

However, this interpretation is non-unique and controversial (Cartigny, 2005; Corgne et al., 2012).
View in article
The carbon isotope ratios (δ13C values) in diamond have a wide range – from -41 ‰ to +3 ‰ (Deines, 1980; Cartigny, 2005; De Stefano et al., 2009) – however, there is controversy over whether the observed variability represents sampling of distinct, unhomogenised, primitive carbon domains, fractionation at mantle conditions, or subduction of carbon that was fractionated by near-surface processes (Cartigny, 2005).
View in article
Cartigny, P. (2010) Mantle-related carbonados? Geochemical insights from diamonds from the Dachine komatiite (French Guiana). Earth and Planetary Science Letters 296, 329–339.

Cartigny (2010) suggested that the depleted carbon isotope signatures of the Jagersfontein transition zone-suite of diamonds were derived from a primordial mantle reservoir and not from crustal materials.
View in article
Collerson, K.D., Williams, Q., Kamber, B.S., Omori, S., Arai, H., Ohtani, E. (2010) Majoritic garnet: A new approach to pressure estimation of shock events in meteorites and the encapsulation of sub-lithospheric inclusions in diamond. Geochimica et Cosmochimica Acta 74, 5939–5957.

Figure 1[...] The small circles are experimental results (from the compilation of Collerson et al., 2010, with the addition of data from Irifune et al., 1986), with colour shading corresponding to the apparent depth that their pressure of equilibration represents.
View in article
Corgne, A., Armstrong, L.S., Keshav, S., Fei, Y., McDonough, W.F., Minarik, W.G., Moreno, K. (2012) Trace element partitioning between majoritic garnet and silicate melt at 10–17 GPa: Implications for deep mantle processes. Lithos 148, 128–141.

However, this interpretation is non-unique and controversial (Cartigny, 2005; Corgne et al., 2012).
View in article
Similarly, evidence based on the rare earth element signatures (observation of negative Eu anomalies) of majoritic garnet inclusions from Jagersfontein, interpreted to reflect feldspar fractionation in crustal protoliths (Tappert et al., 2005b), was disputed by Griffin and O’Reilly (2007) and Corgne et al. (2012) who considered Eu anomalies to reflect redox-related metasomatic signatures, or crystallisation from a melt at high pressures.
View in article
Criss, R.E. (1991) Temperature dependence of isotopic fractionation factors. In: Taylor, H.P., O’Neil, J.R., Kaplan, I.R. (Eds.) Stable Isotope Geochemistry. The Geochemical Society Special Publication 3, 11–16.

This gross homogeneity is a consequence of an initially well-mixed Earth (Pahlevan and Stevenson, 2007), and small values of mass dependent stable isotope fractionation at high temperatures which prevent mantle silicates from substantially fractionating oxygen isotopes among each other (Urey, 1947; Criss, 1991).
View in article
Deines, P. (1980) The carbon isotopic composition of diamonds: Relationship to diamond shape, color, occurrence and vapor composition. Geochimica et Cosmochimica Acta 44, 943–961.

Much of the chemical information that we have on deep carbon comes from the study of diamonds and their inclusions (Deines, 1980).
View in article
The carbon isotope ratios (δ13C values) in diamond have a wide range – from -41 ‰ to +3 ‰ (Deines, 1980; Cartigny, 2005; De Stefano et al., 2009) – however, there is controversy over whether the observed variability represents sampling of distinct, unhomogenised, primitive carbon domains, fractionation at mantle conditions, or subduction of carbon that was fractionated by near-surface processes (Cartigny, 2005).
View in article
High temperature processes, such as CO2 escape from carbon-rich melts, can fractionate both carbon and oxygen isotope ratios (Deines, 1980; Ickert et al., 2013), however this will only occur under unusual circumstances, and the magnitudes of the fractionations are relatively small and cannot account for >4 ‰ shifts.
View in article
Deines, P., Harris, J.W., Gurney, J.J. (1991) The carbon isotopic composition and nitrogen content of lithospheric and asthenospheric diamonds from the Jagersfontein and Koffiefontein kimberlite, South Africa. Geochimica et Cosmochimica Acta 55, 2615–2625.

Rare diamonds from Jagersfontein (South Africa) contain inclusions of majoritic garnet (Deines et al., 1991; Tappert et al., 2005b), an ultra-high pressure mineral reflecting the increasing solubility of pyroxene in the garnet structure at sub-lithospheric depth (Ringwood and Major, 1971).
View in article
Eiler, J.M. (2001) Oxygen isotope variations of basaltic lavas and upper mantle rocks. In: Valley, J.W., Cole, D.R., Rosso, J.J. (Eds.) Reviews in Mineralogy and Geochemistry 43, 415–468.

In contrast, oxygen isotope compositions (d18OVSMOW values) in mantle peridotites, primitive basalts, and the moon have a narrow range, deviating only slightly from +5.5 ‰ (Eiler, 2001).
View in article
Eiler, J.M., Graham, C., Valley, J.W. (1997) SIMS analysis of oxygen isotopes: Matrix effects in complex minerals and glasses. Chemical Geology 138, 221–244.

A major obstacle to accurate SIMS analysis is the dependence of the instrumental mass fractionation on the chemical composition and structure of the target material, the so called “matrix effect” (Russell et al., 1980; Eiler et al., 1997; Vielzeuf et al., 2005; Page et al., 2010).
View in article
Eiler, J.M., Schiano, P., Kitchen, N., Stolper, E.M. (2000) Oxygen-isotope evidence for recycled crust in the sources of mid-ocean-ridge basalts. Nature 403, 530–534.

Thus, variation makes oxygen isotope compositions a uniquely robust tracer of crustal material in the mantle (Eiler et al., 2000; Schulze et al., 2003).
View in article
Griffin, W.L., O’Reilly, S.Y. (2007) Cratonic lithospheric mantle: Is anything subducted? Episodes 30, 43–53.

Similarly, evidence based on the rare earth element signatures (observation of negative Eu anomalies) of majoritic garnet inclusions from Jagersfontein, interpreted to reflect feldspar fractionation in crustal protoliths (Tappert et al., 2005b), was disputed by Griffin and O’Reilly (2007) and Corgne et al. (2012) who considered Eu anomalies to reflect redox-related metasomatic signatures, or crystallisation from a melt at high pressures.
View in article
Gurney, J.J. (1984) A correlation between garnets and diamonds in kimberlites. In: Glover, J.E., Harris, P.G. (Eds.) Kimberlite Occurrence and Origin: A Basis for Conceptual Models in Exploration. University of Western Australia Special Publication, 143–166.

Based on low Cr contents (<0.4 wt. %) the majoritic garnets are classified as "eclogitic", or based on their low Na concentrations, metapyroxenitic (Gurney, 1984 ; Schulze, 2003; Kiseeva et al., 2012).
View in article
Harte, B. (2010) Diamond formation in the deep mantle: The record of mineral inclusions and their distribution in relation to mantle dehydration zones. Mineralogical Magazine 74, 189–215.

The volatile-rich nature of the protolith is consistent with the recent suggestion by Harte (2010) that ultradeep diamonds are associated with devolatilisation and possibly melting reactions.
View in article
Hirschmann, M.M., Dasgupta, R. (2009) The H/C ratios of Earth’s near-surface and deep reservoirs, and consequences for deep Earth volatile cycles. Chemical Geology 262, 4–16.

The volatile abundance, bulk composition, and nature of deep Earth are unresolved geochemical questions (e.g., Hirschmann and Dasgupta, 2009).
View in article
The carbon isotopic systematics of the mantle – which may contain more carbon than the crust-biosphere system (Hirschmann and Dasgupta, 2009) – are not well understood.
View in article
Ickert, R.B., Stern, R.A. (2013) Matrix corrections and error analysis in high-precision SIMS 18O/16O measurements of Ca–Mg–Fe garnet. Geostandards and Geoanalytical Research 37, 429–448.

Analytical procedures follow Ickert and Stern (2013), with modifications described in the Supplementary Materials.
View in article
The total uncertainty for each spot analysis is approximately ±0.4 ‰ (2s), which is mainly due to overdispersion of the primary reference material (±0.3 ‰) and the matrix correction (±0.2 ‰), but includes the uncertainty in the primary reference material and the uncertainty in the session-level mass fractionation correction (see Ickert and Stern, 2013 for details).
View in article
Ickert, R.B., Stachel, T., Stern, R.A., Harris, J.W. (2013) Diamond from recycled crustal carbon documented by coupled δ18O–δ13C measurements of diamonds and their inclusions. Earth and Planetary Science Letters 364, 85–97.

Measuring the oxygen isotope composition of coesite (Schulze et al., 2003) and eclogitic garnet inclusions (Schulze et al., 2013; Ickert et al., 2013) in diamond, a coupled relationship of high d18O values (inclusions) and low δ13C values (host diamonds) emerged, constraining a crustal origin of both the diamond carbon and the diamond substrate.
View in article
Although Lowry et al. (1999) employed a novel, bulk laser fluorination technique to measure oxygen isotope compositions of mineral inclusions in diamond, most subsequent workers have employed secondary ion mass spectrometry (SIMS) for d18O measurements (Schulze et al., 2003; Smart et al., 2012; Ickert et al., 2013; Schulze et al., 2013).
View in article
High temperature processes, such as CO2 escape from carbon-rich melts, can fractionate both carbon and oxygen isotope ratios (Deines, 1980; Ickert et al., 2013), however this will only occur under unusual circumstances, and the magnitudes of the fractionations are relatively small and cannot account for >4 ‰ shifts.
View in article
Moreover, if the carbon isotope data are also considered, there are no processes that can simultaneously create depletion in 13C and enrichment in 18O (Ickert et al., 2013).
View in article
Figure 2 [...] The histogram on the Y-axis is of garnets from eclogite xenoliths (compilation of Ickert et al., 2013), the histogram on the X-axis is of cratonic diamonds (Stachel et al., 2009).
View in article
Irifune, T., Sekine, T., Ringwood, A.E., Hibberson, W.O. (1986) The eclogite-garnetite transformation at high pressure and some geophysical implications. Earth and Planetary Science Letters 77, 245–256.

Experimental work (e.g., Akaogi and Akimoto, 1977; Irifune et al., 1986) demonstrated a linear relationship between the solubility of pyroxene (which can be parameterised by Si-excess over the available tetrahedral sites) and pressure, thereby providing crude minimum depth estimates.
View in article
Figure 1[...] The small circles are experimental results (from the compilation of Collerson et al., 2010, with the addition of data from Irifune et al., 1986), with colour shading corresponding to the apparent depth that their pressure of equilibration represents.
View in article
Kiseeva, E.S., Yaxley, G.M., Hermann, J., Litasov, K.D., Rosenthal, A., Kamenetsky, V.S. (2012) An experimental study of carbonated eclogite at 3.5-5.5 GPa — implications for silicate and carbonate metasomatism in the cratonic mantle. Journal of Petrology 53, 727–759.

Based on low Cr contents (<0.4 wt. %) the majoritic garnets are classified as "eclogitic", or based on their low Na concentrations, metapyroxenitic (Gurney, 1984 ; Schulze, 2003; Kiseeva et al., 2012).
View in article
Lowry, D., Mattey, D.P., Harris, J.W. (1999) Oxygen isotope composition of syngenetic inclusions in diamond from the Finsch Mine, RSA. Geochimica et Cosmochimica Acta 63, 1825–1836.

Although Lowry et al. (1999) employed a novel, bulk laser fluorination technique to measure oxygen isotope compositions of mineral inclusions in diamond, most subsequent workers have employed secondary ion mass spectrometry (SIMS) for d18O measurements (Schulze et al., 2003; Smart et al., 2012; Ickert et al., 2013; Schulze et al., 2013).
View in article
Mattey, D., Lowry, D., Macpherson, C. (1994) Oxygen-isotope composition of mantle peridotite. Earth and Planetary Science Letters 128, 231–241.

These values far exceed the range of upper mantle peridotite and derivative melts (+5.5 ±0.4 ‰; Mattey et al., 1994); in the crust, values this high (in silicate rocks) are only associated with strong interactions between rocks and water at low temperature (Taylor and Sheppard, 1986).
View in article
Mikhail, S., Guillermier, C., Franchi, I.A., Beard, A.D., Crispin, K., Verchovsky, A.B., Jones, A.P., Milledge, H.J. (2014) Empirical evidence for the fractionation of carbon isotopes between diamond and iron carbide from the Earth’s mantle. Geochemistry, Geophysics, Geosystems 15, 855–866.

For example, Mikhail et al. (2014) suggested that iron carbide inclusions in diamonds from Jagersfontein document isotopic fractionation (Δ13Ccarbide-diamond >7 ‰) sufficiently strong to cause the observed 13C depleted signature.
View in article
This relationship is firm evidence for a subducted protolith and makes it extremely unlikely that the associated negative δ13C values are related to deep mantle isotopic fractionation (Mikhail et al., 2014).
View in article
Page, F.Z., Kita, N.T., Valley, J.W. (2010) Ion microprobe analysis of oxygen isotopes in garnets of complex chemistry. Chemical Geology 270, 9–19.

A major obstacle to accurate SIMS analysis is the dependence of the instrumental mass fractionation on the chemical composition and structure of the target material, the so called “matrix effect” (Russell et al., 1980; Eiler et al., 1997; Vielzeuf et al., 2005; Page et al., 2010).
View in article
We employ the technique described by Page et al. (2010) to correct for the bias between the primary reference material and the majorite samples based on Ca-content.
View in article
Pahlevan, K., Stevenson, D.J. (2007) Equilibration in the aftermath of the lunar-forming giant impact. Earth and Planetary Science Letters 262, 438–449.

This gross homogeneity is a consequence of an initially well-mixed Earth (Pahlevan and Stevenson, 2007), and small values of mass dependent stable isotope fractionation at high temperatures which prevent mantle silicates from substantially fractionating oxygen isotopes among each other (Urey, 1947; Criss, 1991).
View in article
Ringwood, A.E., Major, A. (1971) Synthesis of majorite and other high pressure garnets and perovskites. Earth and Planetary Science Letters 12, 411–418.

Rare diamonds from Jagersfontein (South Africa) contain inclusions of majoritic garnet (Deines et al., 1991; Tappert et al., 2005b), an ultra-high pressure mineral reflecting the increasing solubility of pyroxene in the garnet structure at sub-lithospheric depth (Ringwood and Major, 1971).
View in article
Russell, W.A., Papanastassiou, D.A., Tombrello, T.A. (1980) The fractionation of Ca isotopes by sputtering. Radiation Effects and Defects in Solids 52, 41–52.

A major obstacle to accurate SIMS analysis is the dependence of the instrumental mass fractionation on the chemical composition and structure of the target material, the so called “matrix effect” (Russell et al., 1980; Eiler et al., 1997; Vielzeuf et al., 2005; Page et al., 2010).
View in article
Schulze, D.J. (2003) A classification scheme for mantle-derived garnets in kimberlite: A tool for investigating the mantle and exploring for diamonds. Lithos 71, 195–213.

Based on low Cr contents (<0.4 wt. %) the majoritic garnets are classified as "eclogitic", or based on their low Na concentrations, metapyroxenitic (Gurney, 1984 ; Schulze, 2003; Kiseeva et al., 2012).
View in article
Schulze, D.J., Harte, B., Valley, J.W., Brenan, J.M., Channer, D.M.D.R. (2003) Extreme crustal oxygen isotope signatures preserved in coesite in diamond. Nature 423, 68–70.

Thus, variation makes oxygen isotope compositions a uniquely robust tracer of crustal material in the mantle (Eiler et al., 2000; Schulze et al., 2003).
View in article
Measuring the oxygen isotope composition of coesite (Schulze et al., 2003) and eclogitic garnet inclusions (Schulze et al., 2013; Ickert et al., 2013) in diamond, a coupled relationship of high d18O values (inclusions) and low δ13C values (host diamonds) emerged, constraining a crustal origin of both the diamond carbon and the diamond substrate.
View in article
Although Lowry et al. (1999) employed a novel, bulk laser fluorination technique to measure oxygen isotope compositions of mineral inclusions in diamond, most subsequent workers have employed secondary ion mass spectrometry (SIMS) for d18O measurements (Schulze et al., 2003; Smart et al., 2012; Ickert et al., 2013; Schulze et al., 2013).
View in article
Schulze, D.J., Harte, B., EIMF staff, Page, F.Z., Valley, J.W., Channer, D.M.D.R., Jaques, A.L. (2013) Anticorrelation between low δ13C of eclogitic diamonds and high δ18O of their coesite and garnet inclusions requires a subduction origin. Geology 41, 455-458.

Measuring the oxygen isotope composition of coesite (Schulze et al., 2003) and eclogitic garnet inclusions (Schulze et al., 2013; Ickert et al., 2013) in diamond, a coupled relationship of high d18O values (inclusions) and low δ13C values (host diamonds) emerged, constraining a crustal origin of both the diamond carbon and the diamond substrate.
View in article
As in previous studies (e.g., Schulze et al., 2013), the inclusions, which are < 200 μm in their largest dimension, were released by crushing the host diamond, and mounted in epoxy grain mounts along with reference materials.
View in article
Although Lowry et al. (1999) employed a novel, bulk laser fluorination technique to measure oxygen isotope compositions of mineral inclusions in diamond, most subsequent workers have employed secondary ion mass spectrometry (SIMS) for d18O measurements (Schulze et al., 2003; Smart et al., 2012; Ickert et al., 2013; Schulze et al., 2013).
View in article
Simon, L., Lécuyer, C. (2005) Continental recycling: The oxygen isotope point of view. Geochemistry, Geophysics, Geosystems 6, 1–10.

Crustal rocks typically have higher d18O values mainly within the range +6 to +12 ‰ (Simon and Lécuyer, 2005) due to low-temperature reactions involving water.
View in article
Smart, K.A., Chacko, T., Stachel, T., Tappe, S., Stern, R.A., Ickert, R.B., EIMF staff (2012) Eclogite formation beneath the northern Slave craton constrained by diamond inclusions: Oceanic lithosphere origin without a crustal signature. Earth and Planetary Science Letters 319-320, 165–177.

Although Lowry et al. (1999) employed a novel, bulk laser fluorination technique to measure oxygen isotope compositions of mineral inclusions in diamond, most subsequent workers have employed secondary ion mass spectrometry (SIMS) for d18O measurements (Schulze et al., 2003; Smart et al., 2012; Ickert et al., 2013; Schulze et al., 2013).
View in article
Sung, J. (2000) Graphite - diamond transition under high pressure: A kinetics approach. Journal of Materials Science 35, 6041-6054

Since conversion of metastable graphite to diamond is not expected to produce macrocrystals (Sung, 2000), diamond formation during a fluid/melt aided dissolution – precipitation process is likely.
View in article
Stachel, T., Harris J.W., Aulbach, S., Deines, P. (2002) Kankan diamonds (Guinea) III: δ13C and nitrogen characteristics of deep diamonds. Contributions to Mineralogy and Petrology 142, 465–475.

δ13C values for sub-lithospheric diamond have a wide range (from 0 ‰ to -25 ‰; Stachel et al., 2002; Tappert et al., 2005a; Walter et al., 2011) and have often been interpreted as reflecting incorporation of subducted carbon, thereby documenting deep subduction and the subsequent return of originally crustal carbon.
View in article
Stachel, T., Brey, G.P., Harris, J.W. (2005) Inclusions in sublithospheric diamonds: Glimpses of deep Earth. Elements 1, 73–78.

Diamonds and their mineral inclusions are the only materials available from sublithospheric depths and provide the best geochemical information from these otherwise inaccessible regions (Stachel et al., 2005).
View in article
However, there is a small fraction of inclusion assemblages that imply trapping in the asthenosphere, transition zone, or even the lower mantle (Stachel et al., 2005).
View in article
Stachel, T., Harris, J.W., Muehlenbachs, K. (2009) Sources of carbon in inclusion bearing diamonds. Lithos 112(S2), 625-637.

Figure 2 [...] The histogram on the Y-axis is of garnets from eclogite xenoliths (compilation of Ickert et al., 2013), the histogram on the X-axis is of cratonic diamonds (Stachel et al., 2009).
View in article
De Stefano, A., Kopylova, M.G., Cartigny, P., Afanasiev, V. (2009) Diamonds and eclogites of the Jericho kimberlite (Northern Canada). Contributions to Mineralogy and Petrology 158, 295–315.

The carbon isotope ratios (δ13C values) in diamond have a wide range – from -41 ‰ to +3 ‰ (Deines, 1980; Cartigny, 2005; De Stefano et al., 2009) – however, there is controversy over whether the observed variability represents sampling of distinct, unhomogenised, primitive carbon domains, fractionation at mantle conditions, or subduction of carbon that was fractionated by near-surface processes (Cartigny, 2005).
View in article
Tappert, R., Stachel, T., Harris, J.W., Muehlenbachs, K., Ludwig, T., Brey, G.P. (2005a) Diamonds from Jagersfontein (South Africa): Messengers from the sublithospheric mantle. Contributions to Mineralogy and Petrology 150, 505–522.

δ13C values for sub-lithospheric diamond have a wide range (from 0 ‰ to -25 ‰; Stachel et al., 2002; Tappert et al., 2005a; Walter et al., 2011) and have often been interpreted as reflecting incorporation of subducted carbon, thereby documenting deep subduction and the subsequent return of originally crustal carbon.
View in article
Tappert, R., Stachel, T., Harris, J.W., Muehlenbachs, K., Ludwig, T., Brey, G.P. (2005b) Subducting oceanic crust: The source of deep diamonds. Geology 33, 565–568.

Such ultra-deep diamonds have been interpreted as having formed in subducted oceanic crust based on trace element patterns of their inclusions showing negative Eu anomalies and 13C depletion of their diamond hosts (Tappert et al., 2005b).
View in article
Similarly, evidence based on the rare earth element signatures (observation of negative Eu anomalies) of majoritic garnet inclusions from Jagersfontein, interpreted to reflect feldspar fractionation in crustal protoliths (Tappert et al., 2005b), was disputed by Griffin and O’Reilly (2007) and Corgne et al. (2012) who considered Eu anomalies to reflect redox-related metasomatic signatures, or crystallisation from a melt at high pressures.
View in article
Rare diamonds from Jagersfontein (South Africa) contain inclusions of majoritic garnet (Deines et al., 1991; Tappert et al., 2005b), an ultra-high pressure mineral reflecting the increasing solubility of pyroxene in the garnet structure at sub-lithospheric depth (Ringwood and Major, 1971).
View in article
The studied inclusions from Jagersfontein have Si contents of up to 3.5 atoms per formula unit, indicating that some of the inclusions came from depths of at least 500 km (Tappert et al., 2005b), well within the transition zone (Fig. 1).
View in article
We made high-precision SIMS d18O measurements on the majorite inclusions in diamond originally studied by Tappert et al. (2005b), who published trace element measurements of the majorities and carbon isotope compositions of their host diamonds.
View in article
Table 1 Oxygen and carbon isotope compositions of majoritic inclusions and their diamond hosts, respectively. The carbon isotope compositions are from Tappert et al. (2005b).
View in article
Taylor, H.P. (1968) The oxygen isotope geochemistry of igneous rocks. Contributions to Mineralogy and Petrology 19, 1–71.

In rare cases, crustal rocks can become relatively depleted in 18O, with values below +5.5 ‰ (Taylor, 1968).
View in article
Taylor, H.P., Sheppard, S.M.F. (1986) Igneous rocks: 1. Processes of isotopic fractionation and isotope systematics. In: Valley, J.W., Taylor, H.P., O’Neil, J.R. (Eds.) Stable Isotopes in High Temperature Geological Processes. Reviews in Mineralogy. Mineralogical Society of America, 227–271.

These values far exceed the range of upper mantle peridotite and derivative melts (+5.5 ±0.4 ‰; Mattey et al., 1994); in the crust, values this high (in silicate rocks) are only associated with strong interactions between rocks and water at low temperature (Taylor and Sheppard, 1986).
View in article
Urey, H.C. (1947) The thermodynamic properties of isotopic substances. Journal of the Chemical Society (Resumed), 562–562.

This gross homogeneity is a consequence of an initially well-mixed Earth (Pahlevan and Stevenson, 2007), and small values of mass dependent stable isotope fractionation at high temperatures which prevent mantle silicates from substantially fractionating oxygen isotopes among each other (Urey, 1947; Criss, 1991).
View in article
Vielzeuf, D., Champenois, M., Valley, J.W., Brunet, F., Devidal, J.L. (2005) SIMS analyses of oxygen isotopes: Matrix effects in Fe-Mg-Ca garnets. Chemical Geology 223, 208–226.

A major obstacle to accurate SIMS analysis is the dependence of the instrumental mass fractionation on the chemical composition and structure of the target material, the so called “matrix effect” (Russell et al., 1980; Eiler et al., 1997; Vielzeuf et al., 2005; Page et al., 2010).
View in article
Walter, M.J., Kohn, S.C., Araujo, D., Bulanova, G.P., Smith, C.B., Gaillou, E., Wang, J., Steele, A., Shirey, S.B. (2011) Deep mantle cycling of oceanic crust: Evidence from diamonds and their mineral inclusions. Science 334, 54–57.

δ13C values for sub-lithospheric diamond have a wide range (from 0 ‰ to -25 ‰; Stachel et al., 2002; Tappert et al., 2005a; Walter et al., 2011) and have often been interpreted as reflecting incorporation of subducted carbon, thereby documenting deep subduction and the subsequent return of originally crustal carbon.
View in article
Wiechert, U., Halliday, A.N., Lee, D.C., Snyder, G.A., Taylor, L.A., Rumble, D. (2001) Oxygen isotopes and the Moon-forming giant impact. Science 294, 345–348.

The presence of a previously undetected high-d18O, primordial reservoir in the deep Earth is unlikely, given the homogeneity of the mantle and the near identical oxygen isotope systematics between the Earth and Moon (Wiechert et al., 2001), so we suggest that this hypothesis is now untenable.
View in article
top
Supplementary Information
Supplementary Methods
Majoritic garnets were embedded in epoxy resin along with reference garnets S0068 and S0088B (Ickert and Stern, 2013
Ickert, R.B., Stern, R.A. (2013) Matrix corrections and error analysis in high-precision SIMS 18O/16O measurements of Ca–Mg–Fe garnet. Geostandards and Geoanalytical Research 37, 429–448.
). Using the University of Alberta ims1280 at the Canadian Centre for Isotopic Microanalysis, a ~15 μm diameter 133Cs+ (20 keV impact energy) beam was used to sputter 1-2 μm deep pits in inclusions that were mounted in epoxy with garnet reference material. Garnet S0068 was used as the primary reference material for the 18O/16O measurements on the day of the analyses (Fig. S-1). However, the measured δ18O value of the majoritic garnets will also depend on the difference in chemical composition between S0068 and the majoritic garnets. This “matrix effect” is corrected by referencing the garnet measurements to a calibration defined by garnets of known isotopic composition and varying Ca-Mg-Fe abundances, in a manner similar to Page et al. (2010)Page, F.Z., Kita, N.T., Valley, J.W. (2010) Ion microprobe analysis of oxygen isotopes in garnets of complex chemistry. Chemical Geology 270, 9–19.
and described by Ickert and Stern (2013)Ickert, R.B., Stern, R.A. (2013) Matrix corrections and error analysis in high-precision SIMS 18O/16O measurements of Ca–Mg–Fe garnet. Geostandards and Geoanalytical Research 37, 429–448.
. The magnitude of this secondary effect is small and ranges from +0.1 ‰ to +1.1 ‰ and in all cases produces a lower δ18O value than an “uncorrected” value (Fig. S-2).The accuracy of the secondary matrix correction is assessed by the measurement of secondary reference material S0088B, which requires a substantial (>3 ‰) correction (Fig. S-2). The mean δ18O value measured for S0088B was +4.06 ‰, in excellent agreement with the conventionally determined δ18O value of +4.13 ± 0.09 ‰ (Fig. S-2; Ickert and Stern, 2013
Ickert, R.B., Stern, R.A. (2013) Matrix corrections and error analysis in high-precision SIMS 18O/16O measurements of Ca–Mg–Fe garnet. Geostandards and Geoanalytical Research 37, 429–448.
). The repeatability of S0068 provided a measure of single spot precision. On the two mounts on which the majoritic garnets were embedded, S0068 yielded repeatabilities of ±0.16 ‰ and ±0.17 ‰ (1SD, n1 = 16, n2 = 14, no rejections). Each individual grain was measured twice, and the difference between the two spots varied from 0.02 ‰ to 0.26 ‰, well within the 2σ uncertainty for each spot (Fig. S-3). Final uncertainties are a combination of the repeatability of S0068 (which is the dominant source of uncertainty), the correction for session-related mass bias, the uncertainty in the matrix correction, and the uncertainty in the bulk δ18O value of S0068, and are better than ±0.38 ‰ (2σ).We have made no correction for any potential matrix effects associated with the majorite component. However, we note that despite the wide range of majorite component in these crystals (including grains that include only very small amounts of majorite), there is no correlation between the measured δ18O values and the amount majorite in the garnet. This strongly suggests that any matrix effect related to majorite is negligible. This is expected, given that the compositional and crystal-chemical differences between pyrope-rich Ca-Mg-Fe2+ garnets and these majoritic garnets are small.
Two pairs of inclusions (JF-01A, JF-01B and JF-37A, JF37B) are each from the same diamond. In both cases, the measured δ18O values are identical within uncertainty.

Figure S-1 Time series of the majorite analyses. All data are plotted as raw isotope ratios relative to 0.0020052, corrected only for amplifier gains and backgrounds. There are no corrections needed for drift or to eliminate outliers. Tie lines connect analyses of the same grain.
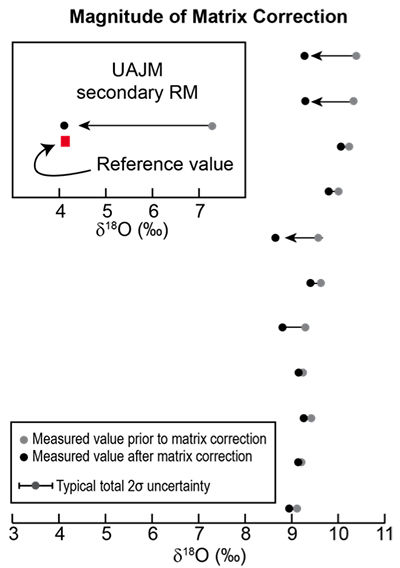
Figure S-2 Magnitude of the matrix correction. Each pair of points represents a single analysis, with the grey point representing the value prior to correction for the matrix effect, and the black point representing the value after the matrix correction. For reference, secondary reference material UAJM is plotted, which, after making a much larger correction for matrix effects than needed for the majorities, agrees within uncertainty with the nominal reference value.

Figure S-3 Plot of each pair of analyses from the same grain. The analyses all agree within uncertainty, suggesting that the uncertainties have been estimated correctly.
Table S-1 Full analytical data for oxygen isotope measurements of majorites.
ID | Session | Time (hours) | δ18Oraw | 1σ1 | δ18OUAG | XCa* | δ18OVSMOW |
S0068_1@28 | IP10155 | 0.92 | 8.15 | 0.05 | |||
S0068_1@29 | IP10155 | 0.98 | 8.60 | 0.05 | |||
S0068_2@07 | IP10155 | 0.07 | 8.67 | 0.05 | |||
S0068_2@08 | IP10155 | 0.13 | 8.78 | 0.04 | |||
S0068_2@09 | IP10155 | 0.20 | 8.72 | 0.05 | |||
S0068_2@10 | IP10155 | 0.27 | 8.76 | 0.04 | |||
S0068_2@11 | IP10155 | 0.33 | 8.77 | 0.04 | |||
S0068_2@12 | IP10155 | 0.40 | 8.70 | 0.07 | |||
S0068_2@13 | IP10155 | 0.47 | 8.48 | 0.04 | |||
S0068_2@14 | IP10155 | 1.48 | 8.69 | 0.06 | |||
S0068_2@15 | IP10155 | 1.60 | 8.63 | 0.04 | |||
S0068_2@16 | IP10155 | 2.40 | 8.68 | 0.07 | |||
S0068_2@17 | IP10155 | 2.50 | 8.77 | 0.05 | |||
S0068_2@18 | IP10155 | 2.57 | 8.65 | 0.04 | |||
S0068_2@19 | IP10155 | 2.63 | 8.66 | 0.06 | |||
S0068_2@6 | IP10155 | 0.00 | 8.51 | 0.07 | |||
S1318_1 | IP10155 | 0.55 | 11.85 | 0.05 | 8.93 | 0.16 | 8.78 |
S1318_2 | IP10155 | 1.70 | 12.04 | 0.05 | 9.11 | 0.16 | 8.97 |
S1319_1 | IP10155 | 0.65 | 12.14 | 0.06 | 9.22 | 0.16 | 9.07 |
S1319_2 | IP10155 | 1.78 | 12.40 | 0.06 | 9.47 | 0.16 | 9.32 |
S1320_1 | IP10155 | 0.73 | 13.02 | 0.05 | 10.09 | 0.16 | 9.94 |
S1320_2 | IP10155 | 1.87 | 13.14 | 0.05 | 10.21 | 0.16 | 10.06 |
S1321_1 | IP10155 | 1.07 | 12.03 | 0.04 | 9.10 | 0.15 | 9.02 |
S1321_2 | IP10155 | 1.95 | 12.10 | 0.05 | 9.17 | 0.15 | 9.09 |
S1322_1 | IP10155 | 1.17 | 12.43 | 0.06 | 9.50 | 0.17 | 9.28 |
S1322_2 | IP10155 | 2.03 | 12.50 | 0.06 | 9.58 | 0.17 | 9.36 |
S1323_1 | IP10155 | 1.25 | 12.85 | 0.04 | 9.92 | 0.17 | 9.71 |
S1323_2 | IP10155 | 2.12 | 12.88 | 0.05 | 9.94 | 0.17 | 9.73 |
S1324_1 | IP10155 | 1.33 | 12.19 | 0.06 | 9.26 | 0.15 | 9.19 |
S1324_2 | IP10155 | 2.20 | 11.99 | 0.07 | 9.06 | 0.15 | 8.98 |
S1325_1 | IP10155 | 1.42 | 13.22 | 0.05 | 10.29 | 0.30 | 9.26 |
S1325_2 | IP10155 | 2.28 | 13.11 | 0.06 | 10.18 | 0.30 | 9.15 |
S0068_1@1 | IP10156 | 3.57 | 9.02 | 0.05 | |||
S0068_1@2 | IP10156 | 3.63 | 9.12 | 0.04 | |||
S0068_1@3 | IP10156 | 3.70 | 9.19 | 0.04 | |||
S0068_1@4 | IP10156 | 3.77 | 9.04 | 0.04 | |||
S0068_2@1 | IP10156 | 3.85 | 8.91 | 0.06 | |||
S0068_2@10 | IP10156 | 5.28 | 9.11 | 0.04 | |||
S0068_2@2 | IP10156 | 3.92 | 8.69 | 0.05 | |||
S0068_2@3 | IP10156 | 3.98 | 8.82 | 0.05 | |||
S0068_2@4 | IP10156 | 4.07 | 8.79 | 0.04 | |||
S0068_2@5 | IP10156 | 4.28 | 8.95 | 0.05 | |||
S0068_2@6 | IP10156 | 4.35 | 8.83 | 0.06 | |||
S0068_2@7 | IP10156 | 4.70 | 9.06 | 0.04 | |||
S0068_2@8 | IP10156 | 5.13 | 9.26 | 0.05 | |||
S0068_2@9 | IP10156 | 5.20 | 9.15 | 0.05 | |||
S0088B_1@1 | IP10156 | 4.13 | 10.44 | 0.08 | 7.16 | 0.97 | 4.02 |
S0088B_1@2 | IP10156 | 4.20 | 10.51 | 0.07 | 7.23 | 0.97 | 4.09 |
S1326_1 | IP10156 | 4.45 | 12.38 | 0.05 | 9.10 | 0.21 | 8.62 |
S1326_2 | IP10156 | 4.80 | 12.61 | 0.05 | 9.33 | 0.21 | 8.85 |
S1328_1 | IP10156 | 4.52 | 12.83 | 0.04 | 9.54 | 0.19 | 8.62 |
S1328_2 | IP10156 | 4.95 | 12.72 | 0.05 | 9.43 | 0.19 | 8.52 |
S1331_1 | IP10156 | 4.62 | 13.52 | 0.04 | 10.23 | 0.12 | 9.14 |
S1331_2 | IP10156 | 5.05 | 13.66 | 0.06 | 10.37 | 0.12 | 9.28 |
δ18Oraw | The 18O/16O ratio measured on the instrument. corrected only for amplifier gains and baslines | ||||||
δ18OUAG | The 18O/16O ratio corrected for mass bias using the UAG garnet | ||||||
δ18OVSMOW | The 18O/16O ratio corrected for Ca-related (matrix effect) mass bias | ||||||
S0068 (UAG) | |||||||
Session | SD | n | SE | 1σ (δ18O)1 | 1σmatrix2 | 2σ total | |
IP10155 | 0.16 | 16 | 0.04 | 0.065 | 0.09 | 0.37 | |
IP10156 | 0.17 | 14 | 0.05 | 0.065 | 0.07 | 0.38 | |
1 The uncertainty on the determination of the bulk δ18O value of the refernce material by laser fluorination. from Ickert and Stern, 2013 Ickert, R.B., Stern, R.A. (2013) Matrix corrections and error analysis in high-precision SIMS 18O/16O measurements of Ca–Mg–Fe garnet. Geostandards and Geoanalytical Research 37, 429–448. . | |||||||
2 The uncertainty on the matrix correction (Ickert and Stern, 2013 Ickert, R.B., Stern, R.A. (2013) Matrix corrections and error analysis in high-precision SIMS 18O/16O measurements of Ca–Mg–Fe garnet. Geostandards and Geoanalytical Research 37, 429–448. ). The uncertainty is a function of the Ca-abundance. and for these garnets varies from 0.05-0.09 ‰. and for simplicity. the largest value is used for all garnets. | |
Supplementary Information References
Ickert, R.B., Stern, R.A. (2013) Matrix corrections and error analysis in high-precision SIMS 18O/16O measurements of Ca–Mg–Fe garnet. Geostandards and Geoanalytical Research 37, 429–448.

Majoritic garnets were embedded in epoxy resin along with reference garnets S0068 and S0088B (Ickert and Stern, 2013).
View in supplementary information
This “matrix effect” is corrected by referencing the garnet measurements to a calibration defined by garnets of known isotopic composition and varying Ca-Mg-Fe abundances, in a manner similar to Page et al. (2010) and described by Ickert and Stern (2013).
View in supplementary information
The mean δ18O value measured for S0088B was +4.06 ‰, in excellent agreement with the conventionally determined δ18O value of +4.13 ± 0.09 ‰ (Fig. S-2; Ickert and Stern, 2013).
View in supplementary information
1 The uncertainty on the determination of the bulk δ18O value of the refernce material by laser fluorination. from Ickert and Stern, 2013.
2 The uncertainty on the matrix correction (Ickert and Stern, 2013). The uncertainty is a function of the Ca-abundance. and for these garnets varies from 0.05-0.09 ‰. and for simplicity. the largest value is used for all garnets.
View in supplementary information
Page, F.Z., Kita, N.T., Valley, J.W. (2010) Ion microprobe analysis of oxygen isotopes in garnets of complex chemistry. Chemical Geology 270, 9–19.

This “matrix effect” is corrected by referencing the garnet measurements to a calibration defined by garnets of known isotopic composition and varying Ca-Mg-Fe abundances, in a manner similar to Page et al. (2010) and described by Ickert and Stern (2013).
View in supplementary information
Figures and Tables
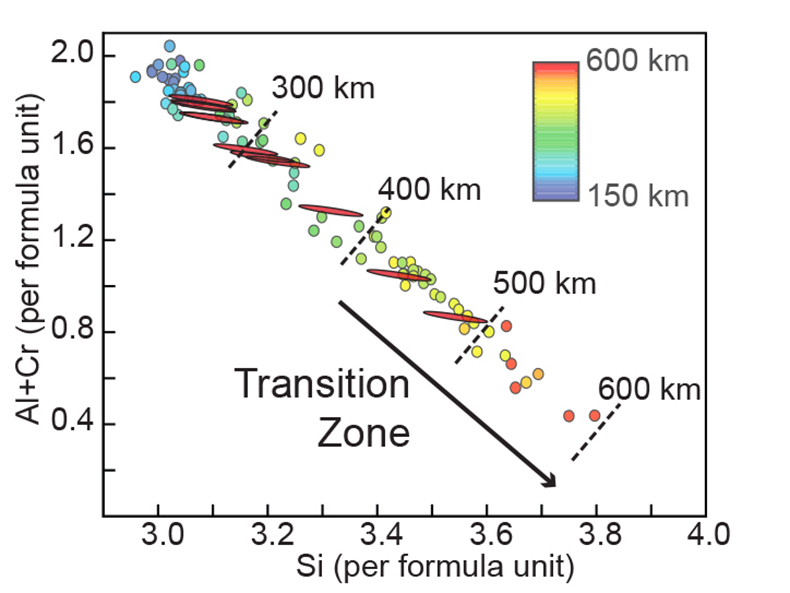
Figure 1 The chemical composition of the Jagersfontein majoritic garnet inclusions based on a formula unit containing twelve oxygens, and their relationship to minimum entrapment depth. The elongate ellipses are the chemical compositions of the garnets and the size and shape of the ellipse represents the 95 % confidence limit based on electron microprobe uncertainties. The small circles are experimental results (from the compilation of Collerson et al., 2010, with the addition of data from Irifune et al., 1986), with colour shading corresponding to the apparent depth that their pressure of equilibration represents. For clarity, uncertainties are not shown on the experimental results; however they are approximately the same size as the uncertainties for the natural garnets. Depth contours are derived from a linear regression of the experimental data relating Si to equilibration pressure.

Figure 2 The δ18O compositions of the majoritic garnets and the δ13C values of their host diamonds. The majoritic garnets and their diamond hosts plot well away from the mantle field. The histogram on the Y-axis is of garnets from eclogite xenoliths (compilation of Ickert et al., 2013), the histogram on the X-axis is of cratonic diamonds (Stachel et al., 2009).
Table 1 Oxygen and carbon isotope compositions of majoritic inclusions and their diamond hosts, respectively. The carbon isotope compositions are from Tappert et al. (2005b). Uncertainties on δ18O values include repeatability of the reference material (RM), the calibration uncertainty, and the uncertainty in the bulk values of the RM. Note that diamonds JF-01 and JF-37 have two inclusions each.
Alias | Spot ID | δ18OVSMOW | Mean | ±95 % c.l. | δ13CVPDB |
JF-01A | S1318_1 | 8.78 | 8.88 | 0.37 | -21.2 |
S1318_2 | 8.97 | ||||
JF-01B | S1319_1 | 9.07 | 9.20 | 0.37 | -21.2 |
S1319_2 | 9.32 | ||||
JF-09A | S1320_1 | 9.94 | 10.00 | 0.37 | -21.8 |
S1320_2 | 10.06 | ||||
JF-22A | S1321_1 | 9.02 | 9.06 | 0.37 | -17.3 |
S1321_2 | 9.09 | ||||
JF-37A | S1322_1 | 9.28 | 9.32 | 0.37 | -21.7 |
S1322_2 | 9.36 | ||||
JF-37B | S1323_1 | 9.71 | 9.72 | 0.37 | -21.7 |
S1323_2 | 9.73 | ||||
JF-39A | S1324_1 | 9.19 | 9.09 | 0.37 | -17.9 |
S1324_2 | 8.98 | ||||
JF-42A | S1325_1 | 9.26 | 9.21 | 0.37 | -17.4 |
S1325_2 | 9.15 | ||||
JF-44B | S1326_1 | 8.62 | 8.74 | 0.38 | -17.7 |
S1326_2 | 8.85 | ||||
JF-55A | S1328_1 | 8.62 | 8.57 | 0.38 | -17.6 |
S1328_2 | 8.52 | ||||
JF-58B | S1331_1 | 9.14 | 9.21 | 0.38 | -23.0 |
S1331_2 | 9.28 |
Supplementary Figures and Tables
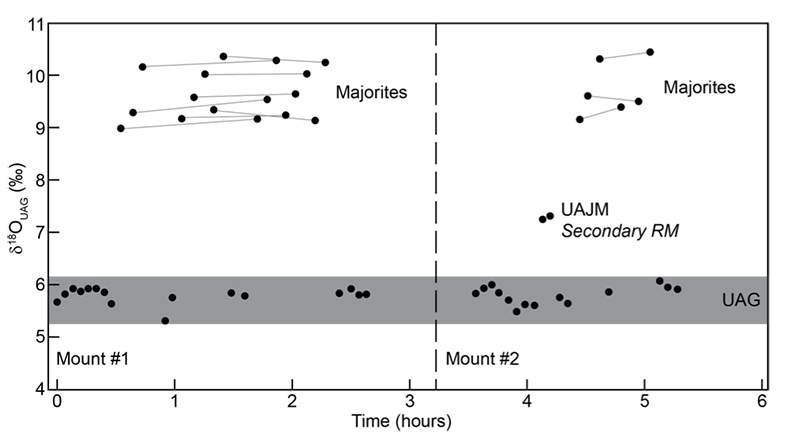
Figure S-1 Time series of the majorite analyses. All data are plotted as raw isotope ratios relative to 0.0020052, corrected only for amplifier gains and backgrounds. There are no corrections needed for drift or to eliminate outliers. Tie lines connect analyses of the same grain.
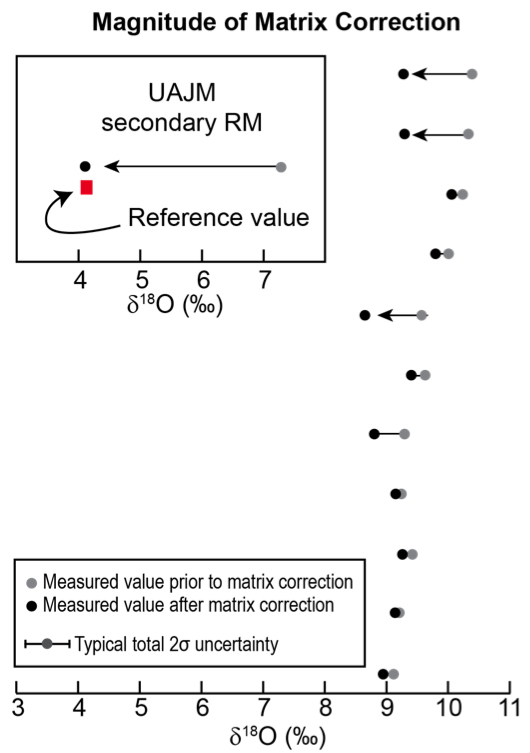
Figure S-2 Magnitude of the matrix correction. Each pair of points represents a single analysis, with the grey point representing the value prior to correction for the matrix effect, and the black point representing the value after the matrix correction. For reference, secondary reference material UAJM is plotted, which, after making a much larger correction for matrix effects than needed for the majorities, agrees within uncertainty with the nominal reference value.

Figure S-3 Plot of each pair of analyses from the same grain. The analyses all agree within uncertainty, suggesting that the uncertainties have been estimated correctly.
Table S-1 Full analytical data for oxygen isotope measurements of majorites.
ID | Session | Time (hours) | δ18Oraw | 1σ1 | δ18OUAG | XCa* | δ18OVSMOW |
S0068_1@28 | IP10155 | 0,92 | 8,15 | 0,05 | |||
S0068_1@29 | IP10155 | 0,98 | 8,60 | 0,05 | |||
S0068_2@07 | IP10155 | 0,07 | 8,67 | 0,05 | |||
S0068_2@08 | IP10155 | 0,13 | 8,78 | 0,04 | |||
S0068_2@09 | IP10155 | 0,20 | 8,72 | 0,05 | |||
S0068_2@10 | IP10155 | 0,27 | 8,76 | 0,04 | |||
S0068_2@11 | IP10155 | 0,33 | 8,77 | 0,04 | |||
S0068_2@12 | IP10155 | 0,40 | 8,70 | 0,07 | |||
S0068_2@13 | IP10155 | 0,47 | 8,48 | 0,04 | |||
S0068_2@14 | IP10155 | 1,48 | 8,69 | 0,06 | |||
S0068_2@15 | IP10155 | 1,60 | 8,63 | 0,04 | |||
S0068_2@16 | IP10155 | 2,40 | 8,68 | 0,07 | |||
S0068_2@17 | IP10155 | 2,50 | 8,77 | 0,05 | |||
S0068_2@18 | IP10155 | 2,57 | 8,65 | 0,04 | |||
S0068_2@19 | IP10155 | 2,63 | 8,66 | 0,06 | |||
S0068_2@6 | IP10155 | 0,00 | 8,51 | 0,07 | |||
S1318_1 | IP10155 | 0,55 | 11,85 | 0,05 | 8,93 | 0,16 | 8,78 |
S1318_2 | IP10155 | 1,70 | 12,04 | 0,05 | 9,11 | 0,16 | 8,97 |
S1319_1 | IP10155 | 0,65 | 12,14 | 0,06 | 9,22 | 0,16 | 9,07 |
S1319_2 | IP10155 | 1,78 | 12,40 | 0,06 | 9,47 | 0,16 | 9,32 |
S1320_1 | IP10155 | 0,73 | 13,02 | 0,05 | 10,09 | 0,16 | 9,94 |
S1320_2 | IP10155 | 1,87 | 13,14 | 0,05 | 10,21 | 0,16 | 10,06 |
S1321_1 | IP10155 | 1,07 | 12,03 | 0,04 | 9,10 | 0,15 | 9,02 |
S1321_2 | IP10155 | 1,95 | 12,10 | 0,05 | 9,17 | 0,15 | 9,09 |
S1322_1 | IP10155 | 1,17 | 12,43 | 0,06 | 9,50 | 0,17 | 9,28 |
S1322_2 | IP10155 | 2,03 | 12,50 | 0,06 | 9,58 | 0,17 | 9,36 |
S1323_1 | IP10155 | 1,25 | 12,85 | 0,04 | 9,92 | 0,17 | 9,71 |
S1323_2 | IP10155 | 2,12 | 12,88 | 0,05 | 9,94 | 0,17 | 9,73 |
S1324_1 | IP10155 | 1,33 | 12,19 | 0,06 | 9,26 | 0,15 | 9,19 |
S1324_2 | IP10155 | 2,20 | 11,99 | 0,07 | 9,06 | 0,15 | 8,98 |
S1325_1 | IP10155 | 1,42 | 13,22 | 0,05 | 10,29 | 0,30 | 9,26 |
S1325_2 | IP10155 | 2,28 | 13,11 | 0,06 | 10,18 | 0,30 | 9,15 |
S0068_1@1 | IP10156 | 3,57 | 9,02 | 0,05 | |||
S0068_1@2 | IP10156 | 3,63 | 9,12 | 0,04 | |||
S0068_1@3 | IP10156 | 3,70 | 9,19 | 0,04 | |||
S0068_1@4 | IP10156 | 3,77 | 9,04 | 0,04 | |||
S0068_2@1 | IP10156 | 3,85 | 8,91 | 0,06 | |||
S0068_2@10 | IP10156 | 5,28 | 9,11 | 0,04 | |||
S0068_2@2 | IP10156 | 3,92 | 8,69 | 0,05 | |||
S0068_2@3 | IP10156 | 3,98 | 8,82 | 0,05 | |||
S0068_2@4 | IP10156 | 4,07 | 8,79 | 0,04 | |||
S0068_2@5 | IP10156 | 4,28 | 8,95 | 0,05 | |||
S0068_2@6 | IP10156 | 4,35 | 8,83 | 0,06 | |||
S0068_2@7 | IP10156 | 4,70 | 9,06 | 0,04 | |||
S0068_2@8 | IP10156 | 5,13 | 9,26 | 0,05 | |||
S0068_2@9 | IP10156 | 5,20 | 9,15 | 0,05 | |||
S0088B_1@1 | IP10156 | 4,13 | 10,44 | 0,08 | 7,16 | 0,97 | 4,02 |
S0088B_1@2 | IP10156 | 4,20 | 10,51 | 0,07 | 7,23 | 0,97 | 4,09 |
S1326_1 | IP10156 | 4,45 | 12,38 | 0,05 | 9,10 | 0,21 | 8,62 |
S1326_2 | IP10156 | 4,80 | 12,61 | 0,05 | 9,33 | 0,21 | 8,85 |
S1328_1 | IP10156 | 4,52 | 12,83 | 0,04 | 9,54 | 0,19 | 8,62 |
S1328_2 | IP10156 | 4,95 | 12,72 | 0,05 | 9,43 | 0,19 | 8,52 |
S1331_1 | IP10156 | 4,62 | 13,52 | 0,04 | 10,23 | 0,12 | 9,14 |
S1331_2 | IP10156 | 5,05 | 13,66 | 0,06 | 10,37 | 0,12 | 9,28 |
δ18Oraw | The 18O/16O ratio measured on the instrument, corrected only for amplifier gains and baslines | ||||||
δ18OUAG | The 18O/16O ratio corrected for mass bias using the UAG garnet | ||||||
δ18OVSMOW | The 18O/16O ratio corrected for Ca-related (matrix effect) mass bias | ||||||
S0068 (UAG) | |||||||
Session | SD | n | SE | 1σ (δ18O)1 | 1σmatrix2 | 2σ total | |
IP10155 | 0,16 | 16 | 0,04 | 0,0065 | 0,09 | 0,37 | |
IP10156 | 0,17 | 14 | 0,05 | 0,0065 | 0,07 | 0,38 | |
1 The uncertainty on the determination of the bulk δ18O value of the refernce material by laser fluorination, from Ickert and Stern (2013). | |||||||
2 The uncertainty on the matrix correction (Ickert and Stern, 2013). The uncertainty is a function of the Ca-abundance, and for these garnets varies from 0.05-0.09 ‰, and for simplicity, the largest value is used for all garnets. | |